Abstract
The influence of water-miscible alcohols (methanol, 1-propanol, 2-propanol, and t-butyl alcohol) on the isomerization of glucose to fructose and mannose was investigated under subcritical aqueous conditions (180–200 °C). Primary and secondary alcohols promoted the conversion and isomerization of glucose to afford fructose and mannose with high and low selectivity, respectively. On the other hand, the decomposition (side-reaction) of glucose was suppressed in the presence of the primary and secondary alcohols compared with that in subcritical water. The yield of fructose increased with increasing concentration of the primary and secondary alcohols, and the species of the primary and secondary alcohols tested had little effect on the isomerization behavior of glucose. In contrast, the isomerization of glucose was suppressed in subcritical aqueous t-butyl alcohol. Both the conversion of glucose and the yield of fructose decreased with increasing concentration of t-butyl alcohol. In addition, mannose was not detected in reactions using subcritical aqueous t-butyl alcohol.
Graphical Abstract
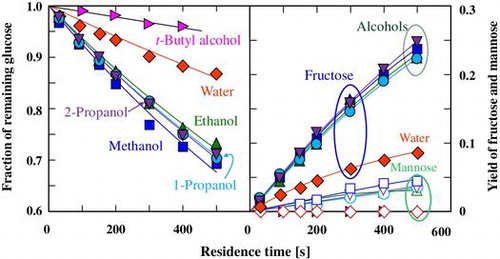
In recent decades, subcritical water has been widely employed as a reaction medium and extractantCitation1–4) due to its high ion product and low dielectric constant. With these two properties, subcritical water can work as a specific solvent, influencing chemical reactivity,Citation5) and also as an environmentally friendly acid–base catalyst for hydrolysis and isomerization reactions.Citation6–9) Many researchers have focused on the decomposition, hydrolysis, and isomerization behavior of saccharides in subcritical water.Citation10–16) It has also been reported that glucose-, mannose-, and fructose-type monosaccharides can mutually isomerize through an alkali-catalysis-like pathway in subcritical water.Citation17,18) When isomerization of glucose, mannose, and fructose was performed in subcritical water, mannose could isomerize to fructose more easily than could other hexoses.Citation17) However, the yields of fructose from both mannose and glucose were not high.
The influence of the addition of alcohols on the decomposition of disaccharides and the isomerization of monosaccharides has been investigated.Citation19–21) It was reported that ethanol can markedly accelerate the alkaline metal hydroxide- and disodium pentasilicate-catalyzed isomerization of glucose to fructose, and vice versa, and can change the apparent isomerization equilibrium.Citation20,21) In the case of the calcium chloride-catalyzed isomerization of glucose in basic solutions, the reaction reached equilibrium faster in aqueous methanol than in aqueous ethanol.Citation22) Fructose and mannose were obtained with almost the same selectivity in aqueous methanol, while mannose was produced with a higher selectivity than fructose in aqueous ethanol at 65 °C for 10 min.Citation22) However, unlike methanol and ethanol, 1- and 2-propanol cannot give these satisfactory results in alkali-catalyzed isomerization of monosaccharides.Citation22)
Our preliminary work showed that subcritical aqueous ethanol could remarkably promote the isomerization of glucose and mannose to fructose and that the isomerizations were accelerated with increasing ethanol concentration.Citation23) Therefore, in this study, we investigated the influence of mixtures of water and water-miscible alcohol (methanol, ethanol, 1-propanol, 2-propanol, and t-butyl alcohol) on the isomerization of glucose under subcritical conditions.
Materials and methods
Materials
Straight-chain alcohols (methanol, ethanol, and 1-propanol), branched-chain alcohol (2-propanol and t-butyl alcohol), d-glucose (>99%), and d-fructose (>99%) were purchased from Wako Pure Chemical Industries (Osaka, Japan). d-Mannose (>99%) was purchased from Nacalai Tesque (Kyoto, Japan).
Isomerization of glucose in subcritical aqueous alcohols
Glucose was dissolved in distilled water and then mixed with alcohol to produce glucose solution at a final concentration of 0.5% (w/v) and an alcohol concentration of 0–80% (v/v). The solution was sonically degassed under reduced pressure before subcritical treatment. The solution reservoir was connected to a helium gasbag to prevent redissolution of atmospheric oxygen. The solution was delivered into a coiled stainless steel tubular reactor (0.8 mmϕ × 1.0 m for subcritical aqueous methanol, ethanol, and 1- and 2-propanol and 0.8 mmϕ × 2.0 m for subcritical aqueous t-butyl alcohol) immersed in an SRX-310 silicone oil bath (Dow Corning Toray Silicone Co., Ltd, Tokyo, Japan) using an LC-10AD VP HPLC pump (Shimadzu Corp., Kyoto, Japan). In order to rapidly terminate the reaction, the reactor effluent was directly introduced into a stainless steel tube (0.8 mmϕ) that was immersed in an ice bath. The temperature and residence time were set to 180 °C and 30–500 s for isomerization in aqueous methanol, ethanol, and 1- and 2-propanol. For isomerization in subcritical aqueous t-butyl alcohol, they were set to 180 °C or 200 °C and 100–1000 s. The pressure inside the reactor was regulated at ca. 10 MPa using a back pressure regulator (Upchurch Scientific, Oak Harbor, Washington, USA).
The residence time was calculated according to our previous method.Citation24) The density of methanol, 1-propanol, or 2-propanol under subcritical conditions was calculated based on the reported data.Citation25–27) However, because the density of t-butyl alcohol under subcritical conditions was not reported, it was assumed to be the same as that of 2-propanol.
The number of replication is one because the error was small for the isomerization of glucose in 40% (v/v) subcritical aqueous ethanol in triplicateCitation23).
Carbohydrate analysis
The reactor effluent was collected in a test tube for HPLC analysis. The HPLC system consisted of an LC-10AD VP HPLC pump (Shimadzu Corp.), an RI-101 refractometer (Showa Denko K. K., Tokyo), and a Cosmosil Sugar-D column (4.6 mmϕ × 250 mm, Nacalai Tesque). A mixture of water and acetonitrile (20:80, v/v) was employed as the mobile phase at a flow rate of 1 mL/min. The column was maintained at 30 °C in a CTO-10A VP column oven (Shimadzu Corp.).
Results and discussion
Isomerization of glucose in subcritical aqueous alcohols
Fig. shows the typical change in the fraction of remaining glucose with residence time at 180 °C in subcritical water and in 60% (v/v) subcritical aqueous alcohols. When glucose was treated for 500 s in subcritical water, the conversion was about 13%. However, the conversion of glucose at a residence time of 500 s was almost doubled and was 27–31% in the presence of any of the water-miscible primary or secondary alcohols. Our preliminary work showed that ethanol can promote the conversion of glucose.Citation23) These facts show that addition of the primary and secondary alcohols will also achieve this conversion. In addition, there was no obvious difference in the promotion ability among the four alcohols.
Fig. 1. Changes in the fraction of remaining glucose with residence time in (♢) subcritical water and 60% (v/v) subcritical aqueous alcohols ((□) methanol, (△) ethanol, (○) 1-propanol, (▽) 2-propanol, and (▷) t-butyl alcohol) at 180 °C.
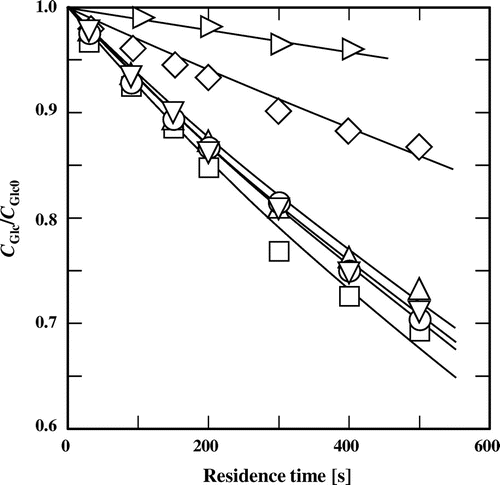
As in subcritical aqueous ethanol,Citation23) fructose were produced from glucose with high yield and selectivity, while mannose was produced with low yield and selectivity in subcritical aqueous methanol, 1- and 2-propanol (Fig. ), where the selectivity was defined as the molar ratio of the product to the consumed substrate. The yields of both fructose and mannose were increased by the addition of these alcohols. These facts indicate that the primary and secondary alcohols used can also promote isomerization. The type of alcohol slightly affected the yields of fructose and mannose. This is in contrast to the reported results, which showed that methanol promoted isomerization more efficiently than ethanol in the alkali-catalyzed isomerization of glucose at low temperature, and that 1- and 2-propanol could not promote the isomerization reaction.Citation20,22) On the other hand, the addition of t-butyl alcohol suppressed the conversion of glucose as discussed in detail later.
Fig. 2. Yields of fructose and mannose derived from glucose at various residence times in subcritical water and in 60% (v/v) subcritical aqueous alcohols at 180 °C.
Note: Symbols are the same as those in Fig. , and the open and closed symbols represent the yields of fructose and mannose, respectively.
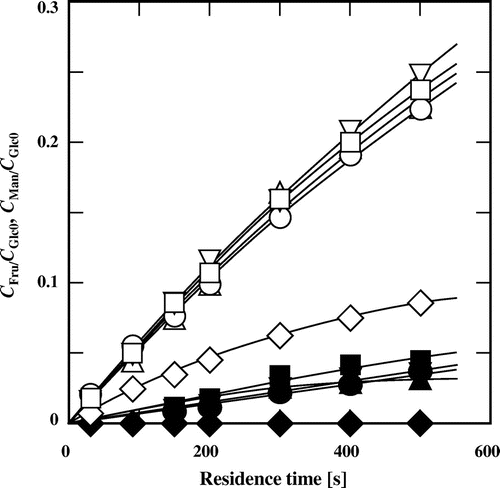
Effect of the concentration of methanol, 1- and 2-propanol on the isomerization of glucose
Fig. shows the typical influence of the concentrations of methanol, 1- and 2-propanol on the selectivities of the derived fructose and mannose, yield of fructose, and fraction of degraded hexoses, for the treatment of glucose at 180 °C for 500 s. The yields of fructose increased with increasing concentration of the alcohols. In the 0–40% (v/v) concentration range, the yield of fructose showed a weak dependence on the concentration of the alcohols. However, in the higher concentration range, increasing the concentration of the alcohols, especially of 2-propanol, greatly raised the yield of fructose. Dependence of the selectivity for fructose on the alcohol concentration was different to that of the yield: The selectivity for fructose reached a maximum value in 60% (v/v) alcohol and then decreased when the concentration of the alcohols increased to 80% (v/v). One reason for this decrease in 80% (v/v) alcohol may be that the decomposition of fructose is promoted by the addition of alcohols.Citation23) Although the addition of alcohol can also promote the decomposition of hexoses,Citation23) the fraction of degraded hexoses did not increase with an increasing concentration of methanol, 1-propanol, or, especially, 2-propanol. When the concentration of 2-propanol was increased to 80% (v/v), most of the consumed glucose was isomerized to fructose and mannose, and 2-propanol exhibited a better effect than methanol and 1-propanol on the isomerization of glucose to fructose. On the other hand, selectivity for mannose was kept at a low level in the presence of the three alcohols.
Fig. 3. Effects of the alcohol concentration on the selectivities of (♢) fructose and (□) mannose, (△) fraction of disappeared hexoses, and (○) yield of fructose for the treatment of glucose at 180 °C for 500 s.
Note: (a) Methanol, (b) 1-propanol, (c) 2-propanol.
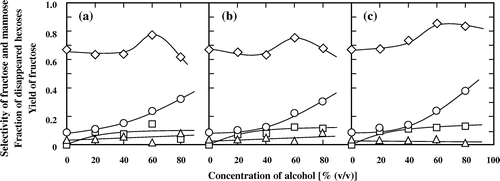
The mechanism of the promotion of isomerization in subcritical aqueous alcohols is unclear. However, isomerization of glucose depended very little on the particular primary and secondary alcohols in subcritical aqueous conditions. Similarity in the behaviors of methanol, ethanol, 1- and 2-propanol in promoting isomerization would indicate that these alcohols promote the isomerization by the same mechanism. Glucose, mannose, and fructose can be mutually interconverted by acid, base, or acid–base catalysts.Citation28–32) Recent research demonstrated that, in aqueous alcohol with high alcohol concentration, the strength of the bond between the proton and oxygen atom of the alcoholic hydroxyl group was weaker than in pure alcohol, especially when the temperature exceeded 130 °C.Citation33) It was also found that glucose could exchange its C-1 proton with the proton of the hydroxyl group of methanol to form fructose.Citation34) Accordingly, a possible mechanism under the subcritical aqueous conditions is that there is a higher dissociation of the alcohol (RO–H) to RO− and H+, either of which can catalyze the isomerization. Because the proton-accepting or electron-donating ability of RO− is higher than that of OH−,Citation35) RO− could promote the isomerizations more effectively than OH− through alkali isomerization.
Isomerization of glucose in subcritical aqueous t-butyl alcohol
The concentration of t-butyl alcohol also affected the conversion of glucose (Fig. ). However, in contrast to the cases for methanol, ethanol, 1- and 2-propanol, the isomerization behavior was different in t-butyl alcohol: Glucose was converted more slowly with an increasing concentration of t-butyl alcohol compared to the conversion of glucose in subcritical water. When glucose was treated in 20% (v/v) t-butyl alcohol at 180 °C for 1000 s, its conversion was almost the same as that at 180 °C for 400 s in subcritical water.
Fig. 4. Changes in the fraction of remaining glucose with residence time at 180 °C in (♢) 80%, (□) 60%, (△) 40%, (○) 20%, and (▷) 0% (v/v) t-butyl alcohol and at (■) 200 °C in 60% (v/v) t-butyl alcohol.
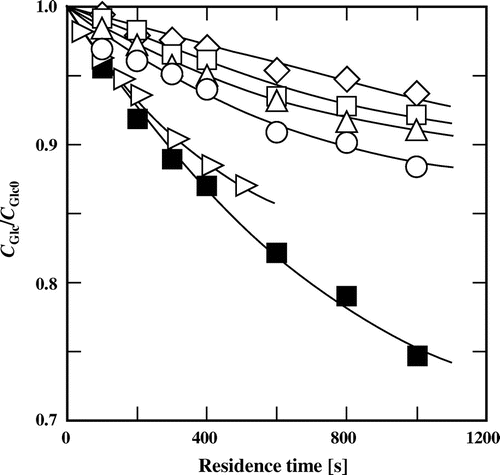
Fructose was also formed from glucose in subcritical aqueous t-butyl alcohol (Fig. ). However, the isomerization behavior was different from that in other subcritical aqueous alcohols, as described above: Only fructose was detected, and mannose was not formed in the presence of t-butyl alcohol. Besides, the yield of fructose was much lower than those obtained in subcritical water and in the presence of the other alcohols tested under the same reaction conditions.
Fig. 5. Yields of fructose derived from glucose at different residence times in subcritical aqueous t-butyl alcohol.
Note: The symbols are the same as those in Fig. .
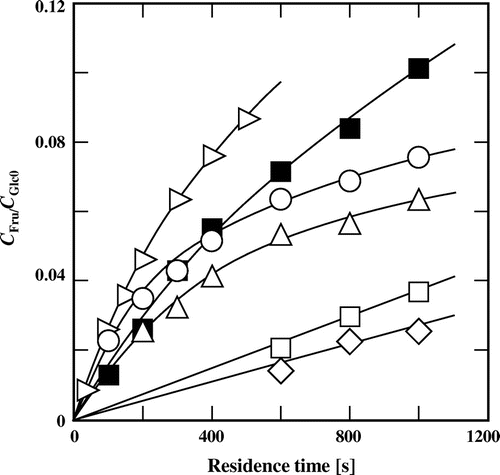
The formation of fructose was slower at a higher t-butyl alcohol concentration. However, the conversion and isomerization were accelerated by increasing the temperature (Figs. and ). When glucose was treated at 200 °C for 1000 s in 60% (v/v) t-butyl alcohol, the conversion of glucose and the yield of fructose reached about 25 and 10%, respectively, which was about threefold and 2.5-fold higher, respectively, than those obtained at 180 °C.
Fig. shows the typical influence of t-butyl alcohol concentration on the selectivity for fructose, the yield of fructose, and the fraction of degraded hexoses, for the treatment of glucose at 180 °C for 1000 s. The dependence of the selectivity for fructose on the t-butyl alcohol concentration was different to that of the other alcohols tested. The selectivity for fructose decreased with increasing t-butyl alcohol concentration. In particular, there was a large decrease in the selectivity when the concentration of t-butyl alcohol exceeded 40% (v/v). The yield of fructose almost linearly decreased with increasing t-butyl alcohol concentration. The fraction of degraded hexoses was also low in subcritical aqueous t-butyl alcohol and was only slightly affected by the t-butyl alcohol concentration.
Fig. 6. Effects of t-butyl alcohol concentrations on (♢) the selectivity of fructose, (△) the fraction of degraded hexoses, and (○) the yield of fructose for the treatment of glucose at 180 °C for 1000 s.
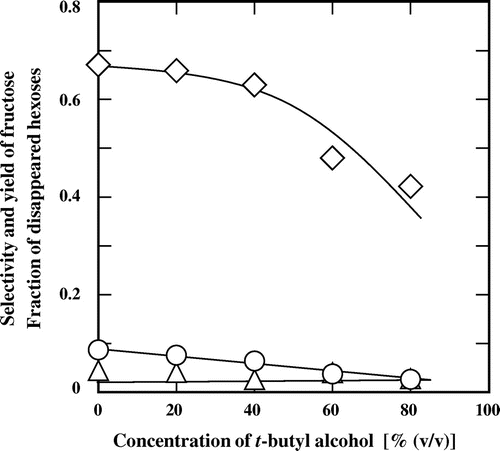
In line with the proposed mechanism regarding the promotion of the isomerization of glucose by primary and secondary alcohols, the reason for the suppression of the isomerization of glucose in subcritical aqueous t-butyl alcohol may be that t-butyl alcohol would be dissociated to H+ and the t-butoxy anion, (CH3)3CO−, which possesses much stronger proton-accepting ability than the alkoxide anions of the other alcohols tested. However, the bulky t-butyl group gives the t-butoxy anion greater steric hindrance, which could suppress the isomerization. As a result, the addition of t-butyl alcohol only caused a diluting effect on the water concentration, which was similar to the addition of ethanol to subcritical water during the hydrolysis of sucrose.Citation24)
In conclusion, the isomerization of glucose was promoted with increasing alcohol concentration in subcritical aqueous primary and secondary alcohols. The type of alcohol slightly affected the isomerization. However, the addition of t-butyl alcohol suppressed the isomerization. These facts suggest a mechanism for the promotion of the monosaccharide isomerization in subcritical aqueous alcohols.
Additional information
Funding
References
- Akiya N, Savage PE. Roles of water for chemical reactions in high-temperature water. Chem. Rev. 2002;102:2725–2750.10.1021/cr000668w
- Sasaki M, Kabyemela BM, Malaluan R, Hirose S, Takeda N, Adschiri T, Arai K. Cellulose hydrolysis in subcritical and supercritical water. J. Supercrit. Fluids. 1998;13:261–268.10.1016/S0896-8446(98)00060-6
- Khuwijitjaru P, Watsanit K, Adachi S. Carbohydrate content and composition of product from subcritical water treatment of coconut meal. J. Ind. Eng. Chem. 2012;18:225–229.10.1016/j.jiec.2011.11.010
- Singh PP, Saldaña MDA. Subcritical water extraction of phenolic compounds from potato peel. Food Res. Int. 2011;44:2452–2458.10.1016/j.foodres.2011.02.006
- Dack MRJ. The influence of solvent on chemical reactivity: an alternative approach. J. Chem. Educ. 1974;51:231–234.10.1021/ed051p231
- Kus NS. Organic reactions in subcritical and supercritical water. Tetrahedron. 2012;68:949–958.
- Patrick HR, Griffith K, Liotta CL., Eckert CA, Gläser R. Near-critical water: a benign medium for catalytic reactions. Ind. Eng. Chem. Res. 2001;40:6063–6067.10.1021/ie010178+
- Lu J, Brown JS, Boughner EC, Liotta CL, Eckert CA. Solvatochromic characterization of near-critical water as a benign reaction medium. Ind. Eng. Chem. Res. 2002;41:2835–2841.10.1021/ie020160e
- Sattar A, Ahmad R, Khan SA. Utilization of limonene fraction of the citrus essential oils. Part I. Production of carvacrol from orange oil. Pak. J. Sci. Res. 1980;23:177–181.
- Aida TM, Sato Y, Watanabe M, Tajima K, Nonaka T, Hattori H, Arai K. Dehydration of d-glucose in high temperature water at pressures up to 80 MPa. J. Supercrit. Fluids. 2007;40:381–388.10.1016/j.supflu.2006.07.027
- Watanabe M, Aizawa Y, Iida T, Aida T, Levy C, Sue K, Inomata H. Glucose reactions with acid and base catalysts in hot compressed water at 473 K. Carbohydr. Res. 2005;340:1925–1930.10.1016/j.carres.2005.06.017
- Ellis AV, Wilson MA. Carbon exchange in hot alkaline degradation of glucose. J. Org. Chem. 2002;67:8469–8474.10.1021/jo025912t
- Kabyemela BM, Adschiri T, Malaluan RM, Arai K. Glucose and fructose decomposition in subcritical and supercritical water: detailed reaction pathway, mechanisms, and kinetics. Ind. Eng. Chem. Res. 1999;38:2888–2895.10.1021/ie9806390
- Haghighat Khajavi S, Kimura Y, Oomori T, Matsuno R, Adachi S. Degradation kinetics of monosaccharides in subcritical water. J. Food Eng. 2005;68:309–313.10.1016/j.jfoodeng.2004.06.004
- Oomori T, Haghighat Khajavi S, Kimura Y, Adachi S, Matsuno R. Hydrolysis of disaccharides containing glucose residue in subcritical water. Biochem. Eng. J. 2004;18:143–147.10.1016/j.bej.2003.08.002
- Kabyemela BM, Adschiri T, Malaluan RM, Arai K. Kinetics of glucose epimerization and decomposition in subcritical and supercritical water. Ind. Eng. Chem. Res. 1997;36:1552–1558.10.1021/ie960250h
- Usuki C, Kimura Y, Adachi S. Isomerization of hexoses in subcritical water. Food Sci. Technol. Res. 2007;13:205–209.10.3136/fstr.13.205
- Lü X, Saka S. New insights on monosaccharides’ isomerization, dehydration and fragmentation in hot-compressed water. J. Supercrit. Fluid. 2012;61:146–156.
- Moody W, Richards GN. Thermolysis of sucrose in the presence of alcohols. A novel method for the synthesis of d-fructofuranosides. Carbohydr. Res. 1981;97:247–255.10.1016/S0008-6215(00)80670-9
- Asaoka H. Isomerization of d-glucose by disodium pentasilicate (Na2Si5O11·xCH3·yH2O) in methanol–water solutions. Carbohydr. Res. 1985;137:99–109.10.1016/0008-6215(85)85152-1
- Vuorinen T, Sjöström E. Kinetics of alkali-catalyzed isomerization of d-glucose and d-fructose in ethanol-water solutions. Carbohydr. Res. 1982;108:23–29.10.1016/S0008-6215(00)81886-8
- Yanagihara R, Soeda K, Shiina S, Osanai S, Yoshikawa S. C-2 epimerization of aldoses by calcium ion in basic solutions. A simple system to transform d-glucose and d-xylose into d-mannose and d-lyxose. Bull. Chem. Soc. Jpn. 1993;66:2268–2272.10.1246/bcsj.66.2268
- Gao D, Kobayashi T, Adachi S. Kinetic analysis for isomerization of glucose, mannose and fructose promoted by ethanol under subcritical aqueous condition. Abstract for the 44th Autumn Meeting of Society of Chemical Engineers; 2012; Japan. Paper ID:A117.(in Japanese)
- Gao D, Kobayashi T, Adachi S. Kinetics of sucrose hydrolysis in a subcritical water–ethanol mixture. J. Appl. Glycosci. 2014;61:9–13.10.5458/jag.jag.JAG-2013_006
- Bazaev AR, Abdulagatov IM, Bazaev EA, Abdurashidova AA, Ramazanova AE. PVT measurements for pure methanol in the near-critical and supercritical regions. J. Supercrit. Fluids. 2007;41:217–226.10.1016/j.supflu.2006.09.012
- Abdulagatov IM, Safarov JT, Aliyev FSh, Talibov MA, Shahverdiyev AN, Hassel EP. Experimental densities and derived thermodynamic properties of liquid propan-1-ol at temperatures from 298 to 423 K and at pressures up to 40 MPa. Fluid Phase Equilib. 2008;268:21–33.10.1016/j.fluid.2008.03.009
- Alaoui FEM, Montero EA, Bazile J-P, Aguilar F, Boned C. Liquid density of oxygenated additive 2-propanol at pressures up to 140 MPa and from 293.15 to 403.15 K. J. Chem. Thermodyn. 2012;54:358–365.10.1016/j.jct.2012.05.016
- Topper YJ, Stetten D Jr. The alkali-catalyzed conversion of glucose into fructose and mannose. J. Biol. Chem. 1951;189:191–202.
- Garrett ER, Young JF. Alkaline transformations among glucose, fructose, and mannose. J. Org. Chem. 1970;35:3502–3509.10.1021/jo00835a069
- De Wit G, Kieboom APG, van Bekkum H. Enolisation and isomerisation of monosaccharides in aqueous, alkaline solution. Carbohydr. Res. 1979;74:157–175.10.1016/S0008-6215(00)84773-4
- Harris DW, Feather MS. Evidence for a C-2→C-1 intramolecular hydrogen-transfer during the acid-catalyzed isomerization of d-glucose to d-fructose. Carbohydr. Res. 1973;30:359–365.10.1016/S0008-6215(00)81822-4
- Román-Leshkov Y, Moliner M, Labinger JA, Davis ME. Mechanism of glucose isomerization using a solid lewis acid catalyst in water. Angew. Chem. Int. Ed. 2010;49:8954–8957.10.1002/anie.v49.47
- Yoshida K, Kitajo A, Yamaguchi T. 17O NMR relaxation study of dynamics of water molecules in aqueous mixtures of methanol, ethanol, and 1-propanol over a temperature range of 283–403 K. J. Mol. Liq. 2006;125:158–163.10.1016/j.molliq.2005.11.009
- Tanase T, Takei T, Hidai M, Yano S. Substrate-dependent chemoselective aldose–aldose and aldose–ketose isomerizations of carbohydrates promoted by a combination of calcium ion and monoamines. Carbohydr. Res. 2001;333:303–312.10.1016/S0008-6215(01)00156-2
- Rived F, Rosés M, Bosch E. Dissociation constants of neutral and charged acids in methyl alcohol. The acid strength resolution. Anal. Chim. Acta. 1998;374:309–324.10.1016/S0003-2670(98)00418-8