Abstract
To verify the presence of enolase related to the chloroplastic glycolysis in rice, database search was carried out and identified seven putative enolase genes in the rice genome. Among them, OsEno1 and OsEno3 encode long proteins with N-terminal extensions. GFP protein fusions of these N-terminal extensions were both targeted to plastids of onion epidermal cell. Promoter::GUS analysis showed that OsEno3 was highly expressed in young developing leaves, but its expression was drastically decreased during leaf development and greening. On the other hand, the expression of OsEno1 was low and detected in limited portions such as leaf sheath at the tiller base. Recombinant OsEno1 protein showed enolase activity with a pH optimum at pH 8.0, whereas OsEno3 did not exhibit detectable activity. Although it remains obscure if OsEno3 encodes a functional enolase in vivo, our results demonstrate that the entire glycolytic pathway does not operate in rice chloroplasts.
Graphical Abstract
Two plastidic enolase genes were identified in rice. These were expressed in non-photosynthetic tissues such as vascular bundle of young leaf blade.
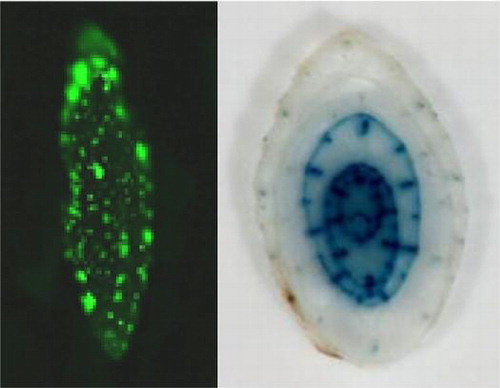
Key words:
Glycolysis is a central metabolic pathway occurring in most living organisms. This pathway, comprising the 10 enzymatic steps from glucose to pyruvate, produces reducing equivalent and ATP in cooperation with the mitochondrial respiration. In addition, it supplies precursors for biosynthesis of numerous compounds such as amino acids, fatty acids, and phenylpropanoids.Citation1) The glycolytic pathway is basically present in the cytosol but it is also present in plastids of some non-photosynthetic tissues.Citation2) In contrast, the chloroplast has not been shown to contain a complete set of glycolytic enzymes so far, because of lacking in enzymes catalyzing two consecutive steps of the pathway, i.e. phosphoglycerate mutase (EC 5.4.2.1) and enolase (EC 4.2.1.11).Citation2,3)
Enolase places at the second to the last step of glycolysis and it catalyzes the reversible dehydration of 2-phosphoglycerate (2-PGA) to phosphoenolpyruvate (PEP). Enolase is encoded by a small gene family in plantsCitation4,5) and present in the cytosol of most plant cells, and also found in plastids of some non-photosynthetic cell such as developing endosperm of Ricinus communisCitation6) and trichome of Arabidopsis thaliana.Citation3) Genes responsible for these differential subcellular localizations were already identified in Arabidopsis.Citation3,4) Arabidopsis contains three enolase genes. Among them, the expression of the cytosolic enolase (AtENO2 and AtENO3) is rather constitutive, whereas the expression of plastidic isozyme (AtENO1) is confined to non-photosynthetic tissues, being high in roots and trichomes.Citation3,4) PEP produced by plastidic enolase or imported via the PEP/Pi transporter from the cytosol is considered to be used for the production of aromatic amino acids and various secondary products through the shikimate pathway in the plastids.Citation7) Moreover, PEP can be converted to pyruvate by plastidic pyruvate kinase and it serves as a precursor for the synthesis of fatty acids, branched-chain amino acids, and isoprenoids in plastids.Citation8Citation−Citation10) Thus, the levels of PEP in the plastids could be an important factor determining the flux of primary and secondary metabolism. However, knockout of AtENO1 did not show any marked effects on plant growth and metabolism, because the cytosolic glycolysis can compensate for the absence of the plastidic pathway.Citation3,4) In fact, double mutant lacking both AtENO1 and PEP/Pi transporter was lethal, suggesting the importance of PEP provided by the action of plastidic enolase or PEP/Pi transporter.Citation11)
In our previous study, we found a novel chloroplastic phosphoenolpyruvate carboxylase (EC EC 4.1.1.31) designated as OsPPC4 (Os01g0208700) in rice (Oryza sativa).Citation12) Organic acids produced from PEP carboxylation by OsPPC4 in the chloroplast are major carbon skeletons used for the ammonium assimilation in rice. The chloroplastic PEPC is suggested to be present in a limited number of plant species which adapted to water logged soil.Citation13) These results raise the possibility that some plants containing the chloroplastic PEPC might have the ability to produce PEP through a novel pathway inside the chloroplast, most likely a glycolysis. In this study, we identified two putative plastidic enolase genes in the rice genome and carried out the expression analysis and functional analysis using recombinant protein, and discuss the presence of the chloroplastic glycolysis in rice.
Materials and methods
Plant growth conditions
Rice (O. sativa L. cv. Nipponbare) plants were grown in soil under natural light conditions from spring to early fall in a temperature-controlled greenhouse (28 °C day/23 °C night). Rice seedlings at the leaf stage of 4.5 were transplanted into 1 L pots supplemented with a chemical fertilizer (N:P:K = 8:8:8) at 0.3 g N per pot. At the panicle initiation stage, the same chemical fertilizer was applied at a rate of 0.1 g N as top dressing.
Determination of the full-length mRNA sequence
Because the mRNA sequence of OsEno3 in the rice annotation project databases (RAP-DB, http://rapdb.dna.affrc.go.jp) lacks in its 5′ region, encoding a truncated enolase protein, 5′RACE was carried out as described previously.Citation14) Total RNA was isolated from leaf sheath of rice using the RNeasy Plant Mini Kit (Qiagen, Valencia, CA). The 5′end of OsEno3 mRNA sequence was obtained by 5′RACE using a SMART RACE cDNA amplification kit (BD Bioscience, San Jose, CA) and Advantage-GC2 PCR kit (BD Bioscience). Primer used for 5′RACE (OsEno3-R2) and nested primer (OsEno3-R3) are listed in Table S1. RACE products were cloned into the pGEM-T Eazy vector (Promega, Madison, WI) and their nucleotide sequence was determined.
Subcellular localization
The cDNA sequences containing N-terminal regions of OsEno1 and OsEno3 were amplified by RT-PCR using the first strand cDNA of leaf blade as a template with the gene-specific primer pairs listed in Table S1. The amplified OsEno1 and OsEno3 cDNA sequences encoded additional 96 and 74 amino acid residues, respectively, at the N-terminal side of methionine, predicted N-terminus in databases. Two PCR products were each cloned into a GFP expression vector (pUC18-CaMV35S-sGFP(S65T)-nos),Citation15) to obtain two chimeric GFP constructs, OsEno1-GFP and OsEno3-GFP. They were introduced into epidermis of Allium cepa scaly bulb by micro projectile bombardment using 0.6 μm gold particles and the gene delivery system (PDS-1000, Bio-Rad, Hercules, CA). As a positive control for plastid targeting, a vector containing a chimeric construct, AtrecA-DsRed2Citation16) was introduced together with OsEno1-GFP or OsEno3-GFP. After incubation in darkness at 25 °C for 24 h, the epidermis was peeled from the bombarded bulb segment and fluorescence from GFP and DsRed was observed with a fluorescence micrometer (BX50, Olympus, Tokyo, Japan) through U-MNIBA and U-MWIG cubes (Olympus), respectively.
RT-PCR analysis
Total RNA was isolated from rice tissues using the RNeasy Plant Mini Kit (Qiagen). The first strand cDNA was synthesized from 1.2 μg of total RNA with oligo (dT)18 and random hexamer as primers using PrimeScript II first Strand cDNA Synthesis Kit (Takara, Otsu, Japan). Semi-quantitative RT-PCR was performed using Quick Taq HS DyeMix (Toyobo, Osaka, Japan) with 5% DMSO and primers listed in Table S1. The DNA polymerase was first activated at 94 °C for 2 min, and PCR was carried out for 23–30 cycles of 30 s at 94 °C, 30 s at 60 °C, and 2 min at 68 °C, followed by a final extension step for 4 min at 68 °C. Expression of the actin gene (AB047313) was examined as an internal control.
Histochemical expression analysis
Rice genomic DNA was prepared from newly developed leaves by the method with cetyltrimethylammonium bromide.Citation17) To construct the promoter::β-glucuronidase (GUS) chimeric gene, the 5′-flanking region of OsEno1 (from −1000 to + 1860, numbered from the translation start site) and OsEno3 (from −2030 to +870) was amplified by PCR using gene-specific primers (Table S1) as described previously.Citation18) The amplified DNA fragment was fused into upstream of the GUS in a binary vector pBI-Hm (a generous gift from Prof. Matsuoka, Nagoya University, Japan). The resultant construct was introduced into calli derived from rice via Agrobacterium-mediated gene transfer. Transgenic plants were regenerated from hygromycin-resistant calli and planted in soil.
Histochemical analysis of GUS activity was performed as described previously.Citation19) Plant tissues were incubated with a GUS staining solution (50 mM sodium phosphate, 0.5 mM 5-bromo-4-chloro-3-indolyl-β-d-glucuronide, 0.5 mM K3Fe(CN)6, 0.5 mM K4Fe(CN)6, and 0.05% (v/v) Triton X-100, pH 7.0) for 24–48 h at 37 °C. An ethanol wash was performed to stop the GUS reaction and remove chlorophyll from the tissues.
Expression and purification of recombinant protein
Recombinant OsEno1 and OsEno3 proteins His-tagged at their N-termini were produced basically as described previously.Citation12) Sequences encoding the mature portion of these genes were amplified by RT-PCR using primers listed in Table S1 and inserted into pET28 (Novagen, Madison, WI). The resultant plasmids were transformed into Escherichia coli expression strain BL21 GOLD (DE3) cells (Novagen). The transformed E. coli was grown in LB medium containing 25 mg L−1 kanamycin at 30 °C until the absorbance at 600 nm reached to 0.6 and then the expression of recombinant protein was induced by incubation with 0.5 mM isopropyl-β-d-thiogalactopyranoside at 30 °C for 3 h. Recombinant protein was purified from a cell lysate by chromatography on His-accept resin (Nacalai Tesque, Kyoto, Japan) according to the manufacture’s instruction and desalted by the NAP-5 gel filtration column (GE Healthcare Bioscience, Piscataway, NJ) using the equilibration buffer containing 50 mM Hepes-KOH pH7.5, 1 mM MgCl2, 0.1 mM EDTA, 1 mM dithiothreitol, 10 μM leaupeptin, and 10% (w/v) glycerol.
Enolase activity
The enolase activity was measured in the direction of PEP formation by coupling with pyruvate kinase and lactate dehydrogenase reaction and monitoring NADH oxidation at 340 nm.Citation3) The activity was measured at pH 6.0–8.5 using the assay mixture contained 100 mM Hepes-KOH (pH 6.0–7.5) or Tricine-KOH (pH 8.0–8.5), 1 mM MgSO4, 10 mM KCI, 1 mM ADP, 0.2 mM NADH, 2.0 units of pyruvate kinase, and 2.75 units of lactate dehydrogenase. The reaction was initiated by the addition of 2-PGA at final concentration of 2.0 mM. For determination of Vmax and Km, the concentrations of 2-PGA were varied from 0.05 to 2.0 mM. Vmax and Km were calculated by the Hanes-Woolf plot.Citation20)
Result
Identification of enolase genes in rice
Database searches identified seven putative enolase genes (OsEno1–7) in rice (Table S2). However, the genomic sequences of OsEno6 and OsEno7 were not found in databases and only their mRNA sequences were identified. Among the seven, OsEno1, 2, 4, 5, 6, and 7 contain the full-length coding sequence. By contrast, the sequence of OsEno3 (Os03g0266200) does not contain the full-length coding sequence and lacks in the 5′ region. Thus, we carried out 5′RACE to determine the 5′end of mRNA sequence and obtained the full-length mRNA sequence of OsEno3 (AB917428). OsEno2, 4, 5, 6, and 7 encode proteins of similar length (445–446 amino acid residues), whereas OsEno1 and OsEno3 encode longer proteins (478–479 residues). These differences in protein length arise from the presence of an N-terminal extension in deduced amino acid sequences of OsEno1 and OsEno3 (Fig. (A) and Supplemental Fig. 1). In addition, the scores for plastid targeting of OsEno1 and OsEno3 analyzed by Target P were higher than that of the other genes (Table S2). Phylogenetic analysis of deduced amino acid sequences of enolase genes from rice and A. thaliana indicates that OsEno3 and AtENO3 belong to the same group and are genetically distant from the other enolase genes (Fig. (B)). The other genes all belong to the same group. OsEno1 is genetically close to AtENO1 which has been shown to encode a plastidic enolase in Arabidopsis.Citation3) In addition, AtENO1 and AtENO3 also contain an N-terminal extension as observed in OsEno1 and OsEno3, whereas AtENO2 is considered to be cytosolic and reported to be a bifunctional protein as LOS2, a regulator of cold-responsive gene expression.Citation4,21)
Fig. 1. Comparisons of deduced amino acid sequences of enolase genes from rice, Arabidopsis and human.
Notes: (A) Alignment of N-terminal regions. Amino acid residues identical among more than six sequences are indicated in red. (B) Phylogenetic tree created by Neighbor-Joining method. Enolase genes from rice are shown in red. The sequences used were AtENO1 (AT1G74030), AtENO2 (AT2G36530), and AtENO3 (AT2G29560) from Arabidopsis and Homo sapiens (AK315417). Bootstrap values based on 1000 replications are shown at each node.
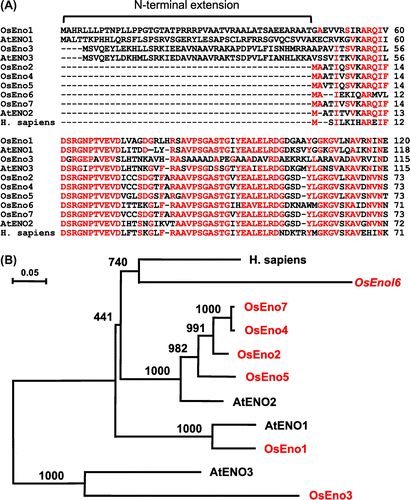
Subcellular localization of OsEno1 and OsEno3
To examine whether the N-terminal extensions of OsEno1 and OsEno3 act as a plastid targeting signal, fusion proteins of N-terminal portions of OsEno1 and OsEno3 with GFP were transiently expressed in epidermis of onion scaly bulb. As a positive control for plastid targeting, we co-expressed a fusion protein of the N-terminal portion of a chloroplastic protein AtrecA with DsRed2.Citation16) The fluorescence from OsEno1-GFP and OsEno3-GFP fusion proteins completely overlapped with the fluorescence from AtRecA-DsRed2 (Fig. ). These findings demonstrate that the N-terminal extensions of these genes act as transit peptides for plastid. Although OsEno3 and AtENO3 proteins belong to the same group in our phylogenetic tree (Fig. (B)), the subcellular localization of OsEno3 was different from that of AtENO3 which reported to be cytosolic.Citation4) Thus, another fusion protein of full-length OsEno3 with GFP was tested, and also showed the localization of plastid. From these results, it is likely that OsEno3 as well as OsEno1 are localized to the plastid in rice.
Fig. 2. Subcellular localization of OsEno1 and OsEno3.
Notes: The fusion protein, OsEno1-GFP, or OsEno3-GFP were expressed in onion epidermal cells after particle bombardment of the chimeric gene constructs. The AtrecA-DsRed2 protein fusion was co-expressed as a control for plastid localization. The fluorescence from GFP (green) and DsRed2 (red) was observed with a fluorescence microscope. Scale bars, 50 μm.
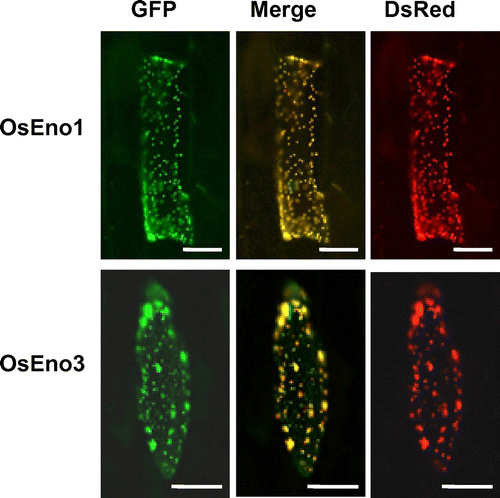
Expression of enolase gene family in various organs of rice
The expression of the enolase gene family was compared in various organs of rice by RT-PCR (Fig. ). OsEno1 and OsEno3, both of which encode plastidic isoforms, showed similar organ specificity and expressed in various organs; they were expressed at relatively high levels in immature seeds but at very low or undetectable levels in leaf blade. Judging from overall band intensities, the expression of OsEno1 would be lower than that of OsEno3. OsEno2 and OsEno4 were expressed in all organs examined at almost the same levels, though the expression in leaf blade was lower than in the other organs. In contrast, OsEno5 was highly expressed in leaf blade and leaf sheath. The expressions of OsEno6 and OsEno7 were very low in all organs examined and could not be detected by PCR even after 30 cycles.
Fig. 3. Organ specificity of the expression of enolase genes in rice examined by RT-PCR.
Notes: Leaf blade, leaf sheath, and root of 4.5 leaf stage, stem, and flower just after anthesis, and immature seed at 10 days after anthesis were sampled between 11:00 and 12:00 and used for analysis of the expression of enolase genes by RT-PCR. The actin gene expression was also analyzed as an internal control. Numbers of PCR cycles are indicated on the right.
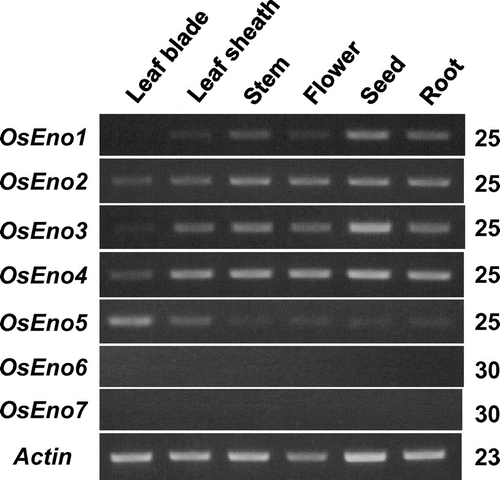
Expression of OsEno1 and OsEno3 was further analyzed by histochemical staining of GUS activity in transgenic rice plants introduced with the promoter::GUS chimeric gene. The expression of OsEno1 was low in most tissues and detected in limited portions such as leaf sheath at tiller base (Fig. ). The expression of OsEno3 was not observed in green tissues including leaf blade (Fig. ). OsEno3 was expressed in vascular bundle and epidermis of young developing leaf blade enclosed in outer leaf sheaths, whereas its expression was not detectable in outer leaf sheath (Fig. (B)). The expression of OsEno3 was particularly high in around xylem and outside of phloem (Fig. (C)). In addition, the expression of OsEno3 was also detected in vascular bundle of culm, but it was low in flower, seed, and root (Fig. (D–G)). Importantly, these results indicate that both putative plastidic enolase, i.e. OsEno1 and OsEno3 were not expressed in photosynthetically active cells like developed mesophyll cells containing chloroplast, suggesting that the chloroplast of rice does not contain enolase and hence, a complete glycolytic pathway.
Fig. 4. Histochemical localization of GUS activity in transgenic plants carrying OsEno1 promoter::GUS construct.
Notes: (A) Cross-section of a fully developed leaf blade. (B) Stem and tiller base of a 15-day-old plant. (C) Cross-section of culm at flowering stage. (D) Flower just after anthesis. (E) Immature seed at 15 days after anthesis (right) and its cross-section (left). (F) Root of a 15-day-old plant. Image in (A) was taken with a light microscope and other with a stereoscopic microscope. Scale bar in A, 20 μm.
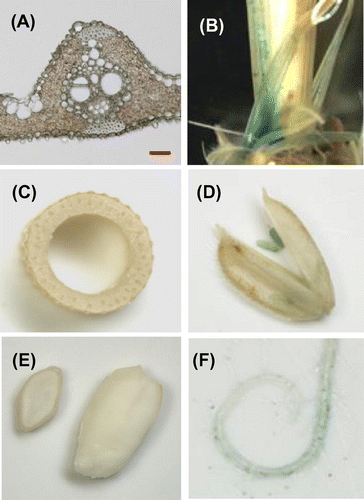
Fig. 5. Histochemical localization of GUS activity in transgenic rice plants carrying the OsEno3 promoter::GUS construct.
Notes: (A) Cross-sections of a fully developed leaf blade. (B) Cross-section of leaf sheath and leaf blade of a young developing leaf, of which leaf blade did not emerge from older leaf sheath. (C) Vascular bundle of a young developing leaf. (D) Cross-section of culm at flowering stage. (E) Flower just after anthesis. (F) Immature seed 10 days after anthesis. (G) Root of a 15-day-old plant. Images in (A) and (C) were taken with a light microscope, and other with a stereoscopic microscope. In (C), X and P indicate xylem and phloem, respectively. LB, Leaf blade; LS, leaf sheath. Scale bar in A and C, 20 μm.
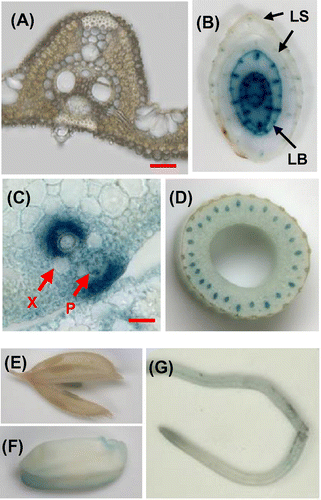
OsEno3 was most highly expressed in vascular bundle of young leaf blade (Fig. ). The expression of OsEno3 in leaf blade was further analyzed using promoter::GUS transgenic rice in relation to leaf development (Fig. (A)). The expression of OsEno3 was only observed in leaf blade before emergence from older leaf sheaths and not exposed to ambient light illumination. The expression was higher in lower positioned immature leaf blade and it was not detectable above 8 cm from the shoot base, suggesting that the expression of OsEno3 decrease with leaf development and greening. In addition, the expression of OsEno3 was largely not overlapped with chlorophyll (including protochlorophyllide) fluorescence in developing leaf blade (Fig. (B)). These results strongly suggest that OsEno3 does not express in the chloroplast of photosynthetic cell of rice.
Fig. 6. Expression of OsEno3 during leaf development. Histochemical localization of GUS activity of transgenic rice seedling carrying OsEno3 promoter::GUS construct is shown.
Notes: (A) Change in GUS activity during leaf development. Cross-sections of the seedling at different positions from the shoot base at the leaf stage of 4.5 are shown. (B) Comparison of GUS staining and chlorophyll fluorescence. Cross-section of the seedling at 1 cm from shoot base is shown. Scale bar, 1.0 mm. (C) GUS activity of an etiolated seedling. Rice plant was grown for 15 days under dark (left). An arrowhead indicates the node of the second leaf. Cross-section of the etiolated seedling (right). BF, bright field; Chl, chlorophyll fluorescence; GUS, GUS staining; LB, Leaf blade; LS, leaf sheath.
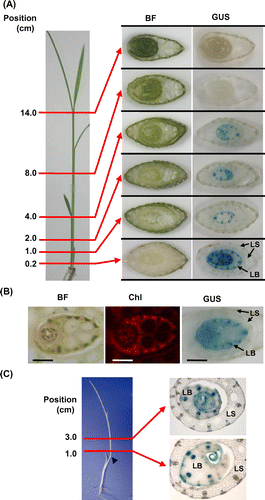
The expression pattern of OsEno3 was similar in etiolated seedling, showing the expression in vascular tissue and epidermis of young developing leaf blade, and not in outer leaf sheath (Fig. (C)). These observations suggest that the expression of OsEno3 is basically unaffected by the presence or absence of photomorphogenesis.
The enolase activity of OsEno1 and OsEno3
Recombinant OsEno1 without the N-terminal transit peptide was active in enolase reaction in the direction of PEP formation (Table and Fig. ). The activity of OsEno1 showed a pH optimum at pH 8.0 (Fig. ). The Vmax value of OsEno1 at pH 8.0 was four times higher than that at pH 7.0 (Table ), indicating that OsEno1 is more active under alkaline conditions as observed in many plastidic enzymes.Citation22) However, Km for 2-PGA at pH 8.0 was also higher than that at pH 7.0. In contrast, we could not detect any enolase activity of recombinant OsEno3. This result is consistent with AtENO3 whose recombinant protein expressed in E. coli also did not show any detectable activity.Citation4)
Table 1. Activity of recombinant OsEno1 and OsEno3 protein expressed in E. coli.
Discussion
It has been suggested that the chloroplast does not contain enolase, as judged from enzyme activities of isolated chloroplast.Citation23,24) Recently, it was shown that plastidic enolase AtENO1 of Arabidopsis was not expressed in photosynthetic tissues.Citation3) In this study, we identified two putative plastidic enolase genes, OsEno1 and OsEno3 in rice (Table S2). Their fusion proteins with GFP were targeted to the plastid (Fig. ), whereas RT-PCR and promoter GUS analyses demonstrated that these genes were not expressed in mature leaves containing chloroplast (Figs. ), suggesting that rice also does not contain enolase in the chloroplast. Considering these observation, we presume that complete glycolytic sequence should be absent in the chloroplast of most plants.
According to the phylogenetic analysis of deduced amino acid sequences, OsEno3 is orthologous to AtENO3 (Fig. (B)). In addition to the homology in amino acid sequence between OsEno3 and AtENO3, both recombinant proteins did not show detectable enolase activity (Fig. and Table ).Citation4) The conserved amino acid E210 (numbering based on Homo sapiens) among functional enolases was substituted to aspartate in OsEno3 and AtENO3 (Supplemental Fig. 1). Only one mutation of D210E in AtENO3 partially restored the enolase activity,Citation4) suggesting that the lack of activity in AtENO3 could be largely attributed to this mutation and AtENO3 should have almost all necessary amino acids for the catalysis of enolase activity. AtENO3 and OsEno3 are classified into different group from other enolase (Fig. (B)). Thus, it is possible that these enzymes may have different characteristics to become catalytically competent enzymes such as the regulation by phosphorylation, redox, or interaction with regulatory subunits. In contrast, some metabolic enzymes have been reported to function as a regulator of gene expression other than a metabolic enzyme. Human enolase reported to bind to the promoter of a c-myc transcription factor and acts as a transcription repressor.Citation25) In Arabidopsis, AtENO2/LOS2 encodes cytosolic enolase, whereas this protein also has DNA-binding activity and regulates gene expression involved in the acclimation to low temperature.Citation21) Therefore, it is also possible that OsEno3 and AtENO3 have some specialized function other than the catalysis of enolase reaction. However, it is unlikely that OsEno3 acts as a transcriptional regulator of nuclear encoded genes, because OsEno3 should be localized in the plastid (Fig. ).
Although OsEno3 is orthologous to AtENO3, their subcellular localization was different. OsEno3 was targeted to the plastid (Fig. ), whereas AtENO3 was reported to be cytosolic.Citation3,4) Although GFP is a relatively small protein, fusion proteins with GFP do not always perform correct folding or interact properly with proteins related to subcellular targeting. We tested two different constructs with different length of OsEno3 including full-length coding region and confirmed that these fusion proteins were both targeted to the plastid (Fig. ). Thus, at least, OsEno3 surely encodes the plastidic protein and rice might contain two distinct plastidic enolases. Considering the expression patterns, OsEno3 may have a similar role as AtENO1 if OsEno3 encoded a functional enolase in vivo. OsEno3 was highly expressed in young developing leaf (Fig. ). Likewise, AtENO1 was expressed in developing shootCitation3) and its expression pattern was more similar to OsEno3 than orthologous OsEno1. Detailed analysis using reversed genetic approaches will be needed for deep understanding the physiological function of OsEno3 in rice.
In this study, we demonstrated that plastidic enolase would not be operative in photosynthetic tissues of rice, as suggested in other plant species.Citation2,3) Thus, it is unlikely that PEP is produced in the chloroplast through the glycolytic sequence starting from 3-PGA which derived from CO2 fixation by rubisco. Probably, PEP in the chloroplast of rice is mainly provided from cytosolic glycolysis via PEP/Pi transporter in the same way as other plant species.Citation11) Considering the activity of chloroplastic PEPC and photosynthetic rate in rice,Citation12,19) the maximum flux of putative glycolytic sequence in concert with chloroplastic PEPC in the chloroplast is estimated to be approximately 6% of 3-PGA production rate. This value is considerably large, because even the sum of rates of all photosynthetic products including sucrose and starch should be less than one-sixth, i.e. 16.7% of the 3-PGA production rate. Thus, the conversion of 3-PGA to PEP should decrease the flux of its reduction to triose phosphate which is utilized for the regeneration of CO2 acceptor ribulose-1,5-bisphosphate and the synthesis of end products. Because triose phosphate production is essential for the photosynthesis and its related metabolisms, it might need not to be perturbed by PEP production. However, the partitioning of fixed carbon to PEP should be unchanged whether PEP is produced within the chloroplast or in the cytosol. If so, the chloroplastic PEP production does not make much effect on metabolism. The fact that complete chloroplast glycolytic sequence is absent in plants implies that the Calvin cycle would become difficult to control if PEP is withdrawn from initial CO2 fixation product. However, the effects of the presence of complete chloroplast glycolytic sequence on plant metabolism have not been demonstrated yet. It was reported that ectopic overexpression of AtENO1 did not largely perturb the photosynthesis and growth.Citation11) The cue1 mutant lacking a PEP/Pi transporter cannot import PEP from the cytosol, showing a reticulate leaf phenotype and stunt growth.Citation26) The overexpression of AtENO1 in cue1 background totally restored the photosynthetic capacity and growth, suggesting that substantial phosphoglycerate mutase activity would be present in the chloroplast.Citation11) In addition, four putative chloroplastic phosphoglycerate mutase genes are identified in the genome of Arabidopsis.Citation11) However, to bring an end to this argument and validate the effects of chloroplastic glycolysis, phosphoglycerate mutase as well as enolase should be overexpressed in a chloroplast and study the metabolism and photosynthesis in detail. Studies on chloroplastic glycolysis may gain a new understanding of the regulation of primary metabolism in plants.
Supplemental material
The supplemental material for this paper is available at http://dx.doi.org/10.1080/09168451.2014.980219.
Supplemental Materials
Download Zip (63.3 KB)Acknowledgments
We would like to thank Prof Makoto Matsuoka (Nagoya University, Japan) and Dr Haruko Imaizumi-Anraku (NIAS, Japan) for generous gifts of vectors.
Additional information
Funding
Notes
Abbreviations: DsRed, Discosoma red fluorescent protein; GFP, Green fluorescent protein; GUS, β-Glucuronidase; PEP, Phosphoenolpyruvate; 2-PGA, 2-Phosphoglycerate; 3-PGA, 3-Phosphoglycerate.
References
- Ap Rees, T. 1990. Carbon metabolism in mitochondria. In: Dennis, DT, Turpin, DH, editors. Plant physiology, biochemistry and molecular biology. Harlow: Longman Scientific & Technical; 1990. p 106–123.
- Givan CV. Evolving concepts in plant glycolysis: two centuries of progress. Biol. Rev. 1999;74:277–309.10.1017/S0006323199005344
- Prabhakar V, Löttgert T, Gigolashvili T, Bell K, Flügge UI, Häusler RE. Molecular and functional characterization of the plastid-localized Phosphoenolpyruvate enolase (ENO1) from Arabidopsis thaliana. FEBS Lett. 2009;583:983–991.10.1016/j.febslet.2009.02.017
- Andriotis VM, Kruger NJ, Pike MJ, Smith AM. Plastidial glycolysis in developing Arabidopsis embryos. New Phytol. 2010;185:649–662.10.1111/j.1469-8137.2009.03113.x
- Lal SK, Lee C, Sachs MM. Differential regulation of enolase during anaerobiosis in Maize. Plant Physiol. 1998;118:1285–1293.10.1104/pp.118.4.1285
- Miernyk JA, Dennis DT. Enolase isozymes from Ricinus communis: partial purification and characterization of the isozymes. Arch. Biochem. Biophys. 1984;233:643–651.10.1016/0003-9861(84)90490-9
- Herrmann KM, Weaver LM. The shikimate pathway. Annu. Rev. Plant Physiol. Plant Mol. Biol. 1999;50:473–503.10.1146/annurev.arplant.50.1.473
- Schulze-Siebert D, Heineke D, Scharf H, Schultz G. Pyruvate-derived amino acids in spinach chloroplasts: synthesis and regulation during photosynthetic carbon metabolism. Plant Physiol. 1984;76:465–471.10.1104/pp.76.2.465
- Ohlrogge JB, Jaworski JG. Regulation of fatty acid synthesis. Annu. Rev. Plant Physiol. Plant Mol. Biol. 1997;48:109–136.10.1146/annurev.arplant.48.1.109
- Lichtenthaler HK. The 1-deoxy-D-xylulose-5-phosphate pathway of isoprenoid biosynthesis in plants. Annu. Rev. Plant Physiol. Plant Mol. Biol. 1999;50:47–65.10.1146/annurev.arplant.50.1.47
- Prabhakar V, Löttgert T, Geimer S, Dörmann P, Krüger S, Vijayakumar V, Schreiber L, Göbel C, Feussner K, Feussner I, Marin K, Staehr P, Bell K, Flügge UI, Häusler RE. Phosphoenolpyruvate provision to plastids is essential for gametophyte and sporophyte development in Arabidopsis thaliana. Plant Cell. 2010;22:2594–2617.10.1105/tpc.109.073171
- Masumoto C, Miyazawa S, Ohkawa H, Fukuda T, Taniguchi Y, Murayama S, Kusano M, Saito K, Fukayama H, Miyao M. Phosphoenolpyruvate carboxylase intrinsically located in the chloroplast of rice plays a crucial role in ammonium assimilation. Proc. Natl. Acad. Sci. USA. 2010;107:5226–5231.10.1073/pnas.0913127107
- Fukayama H, Fujiwara N, Hatanaka T, Misoo S, Miyao M. Nocturnal phosphorylation of phosphoenolpyruvate carboxylase in the leaves of hygrophytic C3 monocots. Biosci. Biotech. Biochem. 2014;78:609–613.10.1080/09168451.2014.891930
- Fukayama H, Tamai T, Taniguchi Y, Sullivan S, Miyao M, Nimmo HG. Characterization and functional analysis of phosphoenolpyruvate carboxylase kinase genes in rice. Plant J. 2006;47:258–268.10.1111/tpj.2006.47.issue-2
- Chiu W, Niwa Y, Zeng W, Hirano T, Kobayashi H, Sheen J. Engineered GFP as a vital reporter in plants. Curr. Biol. 1996;6:325–330.10.1016/S0960-9822(02)00483-9
- Imaizumi-Anraku H, Takeda N, Charpentier M, Perry J, Miwa H, Umehara Y, Kouchi H, Murakami Y, Mulder L, Vickers K, Pike J, Downie JA, Wang T, Sato S, Asamizu E, Tabata S, Yoshikawa M, Murooka Y, Wu GJ, Kawaguchi M, Kawasaki S, Parniske M, Hayashi M. Plastid proteins crucial for symbiotic fungal and bacterial entry into plant roots. Nature. 2005;433:527–531.10.1038/nature03237
- Ku MS, Agarie S, Nomura M, Fukayama H, Tsuchida H, Ono K, Hirose S, Toki S, Miyao M, Matsuoka M. High-level expression of maize phosphoenolpyruvate carboxylase in transgenic rice plants. Nat. Biotechnol. 1999;17:76–80.10.1038/5256
- Fukayama H, Tsuchida H, Agarie S, Nomura M, Onodera H, Ono K, Lee B-H, Hirose S, Toki S, Ku MSB, Makino A, Matsuoka M, Miyao M. Significant accumulation of C4-specific pyruvate, orthophosphate dikinase in a C3 plant. Plant Physiol. 2001;127:1136–1146.10.1104/pp.010641
- Morita K, Hatanaka T, Misoo S, Fukayama H. Unusual small subunit that is not expressed in photosynthetic cells alters the catalytic properties of rubisco in rice. Plant Physiol. 2014;164:69–79.10.1104/pp.113.228015
- Hanes CS. Studies on plant amylases: The effect of starch concentration upon the velocity of hydrolysis by the amylase of germinated barley. Biochem. J. 1932;26:1406–1421.
- Lee H, Guo Y, Ohta M, Xiong L, Stevenson B, Zhu JK. LOS2, a genetic locus required for cold-responsive gene transcription encodes a bi-functional enolase. EMBO J. 2002;21:2692–2702.10.1093/emboj/21.11.2692
- Leegood, RC. Enzymes of the Calvin cycle. In: Lea, PJ, editor. Methods in plant biochemistry. Vol. 3, London: Academic Press; 1990. p. 15–38.
- Stitt M, Ap Rees T. Capacities of pea chloroplasts to catalyze the oxidative pentose phosphate pathway and glycolysis. Phytochem. 1979;18:1905–1911.10.1016/S0031-9422(00)82700-4
- Bagge P, Larsson C. Biosynthesis of aromatic amino acids by highly purified spinach chloroplasts – Compartmentation and regulation of the reactions. Physiol. Plant. 1986;68:641–647.10.1111/ppl.1986.68.issue-4
- Feo S, Arcuri D, Piddini E, Passantino R, Giallongo A. ENO1 gene product binds to the c-myc promoter and acts as a transcriptional repressor: relationship with Myc promoter-binding protein 1 (MBP-1). FEBS Lett. 2000;473:47–52.10.1016/S0014-5793(00)01494-0
- Streatfield SJ, Weber A, Kinsman EA, Häusler RE, Li J, Post-Beittenmiller D, Kaiser WM, Pyke KA, Flügge UI, Chory J. The phosphoenolpyruvate/phosphate translocator is required for phenolic metabolism, palisade cell development, and plastid-dependent nuclear gene expression. Plant Cell. 1999;11:1609–1622.10.1105/tpc.11.9.1609