Abstract
ClbR is a Zn(II)2Cys6 transcriptional activator that controls the expression of cellulase-related genes in response to Avicel and cellobiose in Aspergillus aculeatus. A clbR-overexpressing strain (clbR-OE) that expresses the clbR gene at levels sevenfold higher than the control strain sustainably produced xylanolytic and cellulolytic activities during 10-day cultivation of A. aculeatus, enabling synchronization of xylanolytic and cellulolytic activities at a maximum level. However, clbR overexpression did not simultaneously increase levels of all xylanolytic and cellulolytic enzymes. Peptide mass fingerprint analysis revealed markedly increased production of FIa-xylanase in clbR-OE, whereas expression of FIII-avicelase and FII-carboxymethyl cellulase was unaffected and expression of hydrocellulase was lower in clbR-OE than in the control. Northern blot analysis confirmed that these effects of clbR overexpression on enzyme production were mediated at the transcriptional level. These data suggest that ClbR participates in diverse signaling pathways to control the expression of cellulosic biomass-degrading enzymes in A. aculeatus.
Graphical Abstract
Effects of clbR overexpression on enzyme production in Aspergillus aculeatus vary depending on the cellulosic biomass degrading enzyme species.
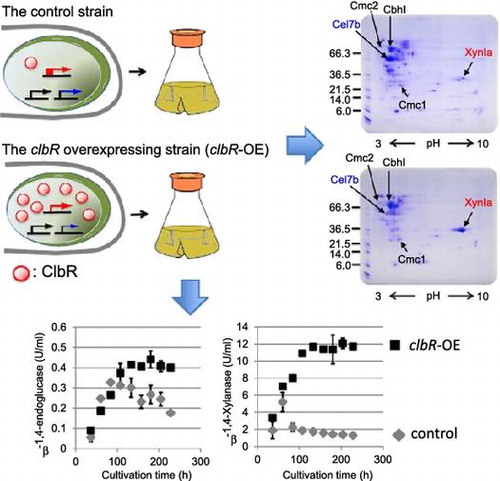
Aspergillus aculeatus No. F-50 [NBRC 108796], which was originally isolated from soil, produces a number of cellulose- and hemicellulose-degrading enzymes that effectively hydrolyze pulp in combination with enzymes from Trichoderma reesei.Citation1) Although A. aculeatus produces cellulolytic and hemicellulolytic enzymes with various substrate specificities that are not provided by enzymes from T. reesei, the productivity of these enzymes is not high enough for industrial use. It is therefore crucial to identify factors that regulate the expression of these genes and apply this information to improve protein production.
The Zn(II)2Cys6-transcription factor XlnR was first identified as a positive regulator for hemicellulolytic enzyme genes in Aspergillus niger.Citation2) Functional analysis of XlnR revealed that XlnR mediates not only xylanolytic-inductive but also cellulose-inductive expression in Aspergillus.Citation3,4) Furthermore, overexpression of xlnR results in increased expression of both xylanolytic and cellulolytic genes in Aspergillus oryzae.Citation4) These data demonstrated that enzyme production could be improved by exploiting the function of a pathway-specific positive transcription factor. Although Xyr1, a XlnR homolog in T. reesei, plays a major role in all induction modes of genes encoding cellulosic biomass-degrading enzymes, it has been reported that a XlnR-independent signaling pathway exists in other species such as Aspergillus nidulans,Citation5) A. oryzae,Citation4) A. aculeatus,Citation6) and Fusarium graminearum.Citation7) Recent studies identified new positive transcription factors that possess the Zn(II)2Cys6 binuclear cluster domain and are involved in an XlnR-independent signaling pathway.Citation8–11) For example, ManR regulates the mannobiose-inductive and cellobiose-inductive expression of mannanolytic and cellulolytic genes in A. oryzae.Citation12)
We have also identified a new transcription factor that possesses the Zn(II)2Cys6 binuclear cluster domain—cellobiose response regulator (ClbR)—from a random insertional mutagenesis library constructed by Agrobacterium tumefaciens-mediated transformation of A. aculeatus.Citation11,13) ClbR has been shown to drive cellulolytic-inductive expression of carbohydrate-active enzyme (CAZy) genes regulated by both the XlnR-independent and XlnR-dependent signaling pathways in A. aculeatus.Citation11) Therefore, we presumed that overexpression of clbR would increase the production of various enzymes in A. aculeatus. In this study, we assessed the effect of overexpression of clbR on the production of the CAZy enzymes and expression of the corresponding genes in A. aculeatus. Although clbR overexpression successfully improved production of both β-1,4-endoglucanase and β-1,4-xylanase, comparative 2D analysis and Northern blot analysis demonstrated that ClbR regulates their genes via diverse signaling pathways. Furthermore, overexpression of clbR resulted in reduced hyphal growth, especially on minimal media. We propose a model for the regulatory mechanism of the CAZy genes in A. aculeatus in which ClbR regulates gene expression by competitively or cooperatively interacting with other transcription factors.
Materials and methods
Strains
The A. aculeatus ku80 mutant MR12 (pyrG1; Δku80) was used as the host for overexpression of the clbR gene.Citation14) MR12 and the clbR-overexpressing strain (pyrG1; Δku80; ΔclbR::Ptef-clbR::pyrG) were propagated at 30 °C in minimal medium (MM) supplemented with and without 0.1% uridine, respectively. MM was converted to complete medium (CM) by the addition of 0.2% (w/v) Difco Bacto-peptone, 0.1% (w/v) Difco Bacto yeast extract, and 0.1% (w/v) casein hydrolysate (http://www.fgsc.net/methods/anidmed.html). The growth rate of the control strain (MR12) and clbR-overexpressing strain (clbR-OE) was investigated on MM and CM plates supplemented with 0.1% uridine and 1% of the indicated carbon sources on day 5 post-inoculation. Escherichia coli DH5αF′ was used for plasmid construction.
Nucleic acid analysis
PCR was performed using PrimeSTAR HS DNA polymerase (TaKaRa, Kyoto, Japan) as described in the manufacturer’s instructions, except that the annealing temperature was 55 °C and we performed 30 PCR cycles. All primers used in this study are shown in Table . Genomic DNA and total RNA were isolated from ground mycelia with Micro Smash MS-100R (Tomy, Tokyo, Japan) as described previously.Citation15)
Table 1. List of primers.
clbR overexpression
The A. aculeatus clbR-overexpressing strain (pyrG1; Δku80; ΔclbR::Ptef-clbR::pyrG) was generated by replacing the promoter region of clbR with the translation elongation factor 1-alpha (Ptef) promoter using pyrG as a marker. To express clbR under the control of Ptef at the clbR locus, the 5′ regions flanking clbR and Ptef were amplified from genomic DNA using the primers FA5-F1_SacII and FA5-tef-R or tef-FA5-F and Ptef-R1_Not, respectively. The resultant DNA fragments were fused by PCR using the primers FA5-F1_SacII and Ptef-R1_Not and digested with SacII and NotI. The clbR ORF with the 3′ flanking region was amplified from genomic DNA using the primers FAoe-F1_Not and FA3-R1_EV and digested with NotI and EcoRV. pCFAP possessing the A. nidulans pyrG gene and the 3′ region flanking clbRCitation11) was digested with NotI, blunted with T4 DNA polymerase, and digested with SacII. SacII was used to clone the above DNA fragments to yield the clbR-overexpressing cassette, namely pTFAgP-FA. The clbR-overexpressing cassette was amplified with the primers FA5-F1_SacII and co22aC-R using pTFAgP-FA as a template and was introduced into the MR12 strain using the protoplast-PEG method. Insertion of the clbR-overexpressing cassette into the clbR locus was confirmed by Southern blot analysis using a DNA probe amplified by the primers FA5-F1_SacII and FArH-R1_Bam. The primers used to amplify these fragments are shown in Table . Vectors were constructed using standard cloning methods, and critical regions were confirmed by sequencing prior to use.
Gene expression analysis
Gene expression levels were quantitated by Northern blot hybridization. The mycelia were harvested by paper filtration, washed with double-distilled water, and immediately frozen in liquid nitrogen. Total RNA was isolated using TRIzol reagent as recommended by the manufacturer (Invitrogen/Life Technologies, Carlsbad, CA, USA). Data shown are representative of biologic replicates performed in three independent experiments. Total RNA (500 ng) was primed with oligo-dT using the ReverTra Ace qPCR Master Mix with gDNA Remover (Toyobo, Osaka, Japan). Quantitative real-time PCR (qPCR) was performed in a Thermal Cycler Dice Real-Time system (TaKaRa). For the amplification reactions, a SYBR Green I assay using THUNDERBIRD qPCR Mix (Toyobo) was performed in a reaction volume in 20 μl. The primers used for qPCR are listed in Table . Expression of the glyceraldehyde-3-phosphate dehydrogenase A gene (gpdA) was used as an internal control.
Peptide mass fingerprinting
A. aculeatus MR12 and clbR-OE strains were cultured in CM including 1% wheat bran as a sole carbon source. A. aculeatus culture supernatants were collected on the 4th day, filtered with Miracloth (Calbiochem, La Jolla, CA, USA), and precipitated with ethanol. Samples were applied to 2D electrophoresis as recommended by the manufacturer (Life Technologies). The protein band was excised from the Coomassie brilliant blue-stained gel (Bio-Safe CBB G-250 stain, Bio-Rad Laboratories), destained, and subjected to in-gel digestion with trypsin according to Shevchenko et al.Citation16) The samples were subjected to mass spectrometry using a hybrid ion-trap–time-of-flight mass spectrometer (LCMS-IT-TOF, Shimadzu, Kyoto, Japan) in positive-ion mode and equipped with a nanoelectrosprayer (GL Science, Kyoto, Japan). The A. aculeatus in-house draft genome sequence database was searched to identify sequences corresponding to spectral data using the Mascot MS/MS ion search engine (Matrix Science, London, UK).
Enzyme assays
Enzyme production by the control and clbR-OE strains was measured using 1.5 mM p-nitrophenyl-β-D-glucoside (pNp-glc, Sigma) as a specific substrate for β-glucosidase; by the Somogyi–Nelson method with 1% birchwood xylan (Sigma–Aldrich, Tokyo, Japan) for β-1,4-xylanase; and using 1% sodium carboxymethyl cellulose (CMC; Wako Pure Chemical Industries, Osaka, Japan) for β-1,4-endoglucanase. The reaction was performed in 100 mM sodium acetate buffer (pH 5.0) at 37 °C. One unit of enzyme activity of β-glucosidase was defined as the amount of enzyme releasing 1 μmol of p-nitrophenol per minute. In reactions using CMC or xylan as substrate, one unit of enzyme activity was defined as the amount of enzyme releasing 1 μmol of glucose or xylose per minute, respectively. Endoprotease activities were assayed as described by Chang et al. with minor modification.Citation17) Proteinase activities were measured using 0.4%(w/v) azocasein in 150 mM acetate buffer (pH 5.0). One unit of azocasein was defined as the amount of enzyme producing an increase of 0.1 optical density units per minute.
Other methods
Genomic DNA was isolated as described previously.Citation15) Dig-labeled DNA probes were amplified by PCR with the primer pairs listed in Table .
Results
Isolation of the clbR-overexpressing strain
To assess the effect of overexpression of clbR on the production of cellulosic biomass-degrading enzymes and expression of their genes in A. aculeatus, we first constructed a clbR-overexpressing strain that expresses clbR under the control of the Ptef promoter, which is a high-level constitutive promoter in Aspergillus. The clbR-overexpressing cassette was introduced into the clbR locus as a single copy by homologous recombination, as confirmed by Southern blot analysis (Fig. ), yielding the clbR-overexpressing strain (clbR-OE). Expression of clbR in the presence of 1% glucose, 1% Avicel, and 1% wheat bran was increased approximately sevenfold in the clbR-OE strain compared with that in the control strain (Fig. ).
Fig. 1. Construction of the clbR-overexpressing strain (clbR-OE).
Notes: The illustrations show restriction enzyme maps of the clbR locus in the MR12 and clbR-OE strains. The solid bars indicate DNA regions used as probes for Southern blot analysis to detect DNA fragments digested with HindIII and ScaI. The vertical and horizontally hatched boxes are the flanking regions for gene replacement at the clbR locus. The 0.5-kb downstream flanking region of clbR (solid boxes) was attached at 5′-end of pyrG, introducing direct repeats for marker recycling. The clbR promoter region (PclbR) was replaced with the tef promoter (Ptef).
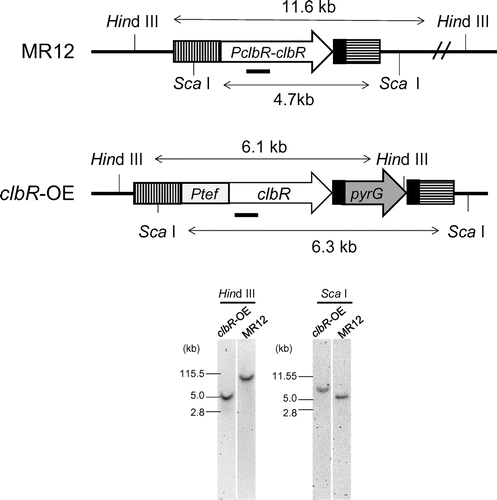
Fig. 2. qPCR analysis of clbR in MR12 and clbR-OE grown for 12 h in MM containing 1% D-glucose (solid bars), 1% Avicel (hatched bars), and 1% wheat bran (open bars).
Notes: gpdA was used as an endogenous control in all samples. The level of clbR under the glucose condition in MR12 was assigned a value of 1 (broken line). The results shown are the means of three independent experiments, and the error bars indicate the standard deviations.
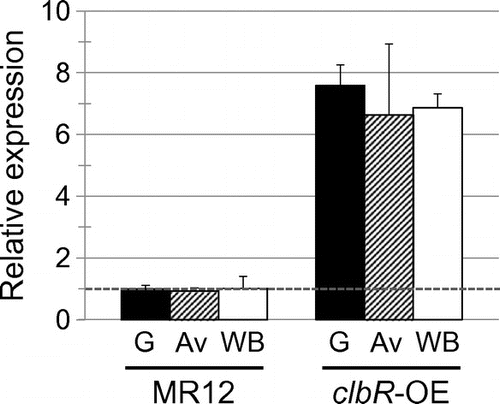
The physiological phenotype of the representative clbR-OE strain was investigated in details with respect to the utilization of various carbon sources because three independently isolated clbR-OE strains showed the same reduced growth on MM supplemented with indicated carbon sources (Table ). Contrary to our assumption that clbR-OE would grow well on media containing cellulosic carbon sources, the clbR-OE strain formed colonies approximately half the size of those produced by the control MR12 strain on MM supplemented with 1% glucose, 1% Avicel, 1% xylan, and 1% wheat bran as a sole carbon source, although the poor hyphal growth tended to be complemented on CM, especially by supplementation with glucose (p < 0.05 by Student’s t-test). In contrast, conidial production per fungal colony area was increased in clbR-OE, suggesting that the clbR overexpression may affect not only the production of cellulosic biomass-degrading enzymes in A. aculeatus but also hyphal growth, although further analysis of this is required.
Table 2. Scoring of growth rate of the control and clbR-OE strains on minimal medium (MM) and complete medium (CM) containing 1% (w/v) indicated carbon sources.
clbR overexpression enabled sustainable production of cellulase and xylanase
We next assessed the effect of clbR overexpression on the production of cellulosic biomass-degrading enzymes in liquid complete media supplemented with wheat bran as an inducible carbon source. This culture condition is one of the best conditions not only for cellulosic biomass-degrading enzyme production in A. aculeatus, but also for complementing the reduced growth of clbR-OE (Table ). The production of β-1,4-endoglucanase, β-1,4-xylanase, and β-glucosidase was assayed and normalized against culture volume rather than fungal biomass for MR12 and clbR-OE, which is relevant for the application of this technique to enzyme production systems in industrial use (Fig. ). Production of β-1,4-endoglucanase by MR12 reached a maximum level (0.33 U/ml) on the 4th day, followed by a gradual decrease. Interestingly, β-1,4-endoglucanase activity in clbR-OE continued to increase until the 8th day (0.44 U/ml) and subsequently remained at a high level. As a result, compared with MR12, β-1,4-endoglucanase production in clbR-OE was increased 1.3-fold for comparison of maximum level and 2.3-fold for comparison of production on the 10th day (Fig. (A)). Furthermore, clbR overexpression had a marked effect on β-1,4-xylanase production. Accumulation of β-1,4-xylanase in MR12 culture supernatant reached a maximum on the 3rd day, followed by a drastic reduction, whereas β-1,4-xylanase activity in the clbR-OE culture supernatant reached a maximum level on the 6th day and was sustained for 10 days. clbR overexpression improved β-1,4-xylanase production 2.3-fold by maximum level and 9.0-fold by production on the 10th day (Fig. (B)). It should be noted that this improvement in enzyme productivity established a simple method to prepare enzyme solution containing maximum levels of both β-1,4-endoglucanase and β-1,4-xylanase activities. However, β-glucosidase production was not improved in the clbR-OE strain of A. aculeatus compared with the control (Fig. (C)).
Fig. 3. Enzyme activity analysis of the MR12 and clbR-OE strains.
Notes: (A) β-1,4-endoglucanase activities using carboxymethyl cellulose as a substrate. (B) β-1,4-xylanase activities using beechwood xylan as a substrate. (C) β-glucosidase activities using pNp-glc as a substrate. The fungal strains were cultivated in complete medium supplemented with 1% (w/v) wheat bran as a sole carbon source and inducers for their enzymes at 30 °C for the indicated time. The values are means of three biologic replicates. The error bars show standard deviations. Black boxes and gray diamonds indicate enzyme activities in the clbR-OE and MR12 strains, respectively.
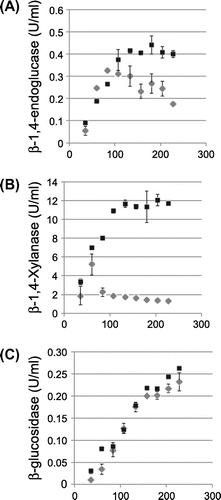
Accumulation of enzymes in the late growth phase in clbR-OE might be caused by a reduction in extracellular proteases. Because acid protease such as aspergillopepsin (PEP) are the major extracellular proteases in A. niger and A. fumigatus,Citation18,19) extracellular endoprotease activities were assayed using azocasein as a substrate. The pH of the culture supernatant of both the control and clbR-OE strains was approximately 4.0 during cultivation, which satisfied the conditions required for maturation of the pro-PEP enzyme.Citation20) An endoprotease assay revealed no difference in extracellular endoprotease activities between MR12 and clbR-OE (data not shown), suggesting that accumulation of β-1,4-endoglucanase and β-1,4-xylanase could be triggered by stimulation at the transcriptional level, although further analysis of protease production in clbR-OE is required.
Comparative two-dimensional electrophoresis of secreted proteins in control and clbR-OE strains
We identified enzyme components secreted from MR12 and clbR-OE strains by peptide mass fingerprinting. An equal volume of culture supernatant from each strain was analyzed by two-dimensional (2D) polyacrylamide gel electrophoresis using a non-linear immobilized pH gradient (3–10) in the first dimension of separation. Detected protein spots were excised from the gels, digested with trypsin, and analyzed by LCMS-IT-TOF. Comparative 2D analysis revealed that the abundance of several spots changed, but no noticeable differences in the pattern of spots between the two gels were observed (Fig. ). The protein showing the greatest increase in abundance in clbR-OE was FIa-xylanase (XynIa; Glycoside hydrolase family 10, GH10), which was present at a much higher level than in MR12 (Fig. ). This result implied that accumulation of XynIa resulted in the increase of β-1,4-xylanase activity in culture supernatant of the clbR-OE strain. In contrast, the level of hydrocellulase (Cel7b; GH7), one of the most abundant proteins in MR12 that is homologous to cellobiohydrolase but without the carbohydrate-binding module, was substantially lower in clbR-OE. Furthermore, FIII-avicelase (CbhI; GH7), FII-carboxymethyl cellulase (Cmc2; GH5), and FI-carboxymethyl cellulase (Cmc1; GH12) appear to be accumulated to equivalent extents in the culture supernatant of both strains.
Fig. 4. 2D gel electrophoresis of day 4 culture supernatant from MR12 (A) and clbR-OE (B) strains.
Notes: An equal volume of culture supernatant was desalted and analyzed by 2DE using standard protocols, and visualized by Coomassie brilliant blue. The identity of proteins determined by LCMS-IT-TOF is indicated by arrows.
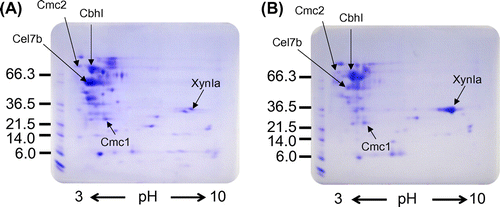
ClbR regulates gene expression via multiple signaling pathways
It should be noted that the expression of cbhI, cmc1, cmc2, xynIa, and cel7b showed an approximately 80% reduction in the clbR deletion mutant compared with MR12 under the Avicel-inducing condition according to the previous reportCitation11) and the recent study of cel7b (unpublished), indicating that the induction of all tested genes by Avicel is regulated by ClbR. To investigate whether the modified production of enzymes in the clbR-OE strain was controlled at the transcriptional level, transcripts of the cbhI, cel7b, cmc1, cmc2, and xynIa genes were quantified at 6- and 12-h post-induction (i.e. the early stage of induction) under Avicel- and wheat bran-inducing conditions in MR12 and clbR-OE (Fig. ). Under the Avicel-inducing condition, the expression of cbhI, cel7b, and cmc2 was enhanced markedly at 6- and 12-h post-induction in clbR-OE. However, the expression of xynIa was the same in clbR-OE and MR12, whereas the expression of cmc1 was slightly reduced in clbR-OE. Similar varied effects on the expression profiles of tested genes were observed under wheat bran-inducing condition but with a different profile. The expression levels of cbhI and cmc2 in response to wheat bran in both MR12 and clbR-OE were similar to those in MR12 in the presence of Avicel. In the case of cel7b and cmc1, expression was induced to a greater extent in response to wheat bran than to Avicel in MR12, whereas the response to wheat bran was lower in clbR-OE than that in MR12. The expression of xynIa was induced more strongly by wheat bran more than by Avicel in MR12 and was further stimulated in clbR-OE. These data demonstrated a highly significant correlation between the amount of protein and transcript in the early stage of induction in the presence of wheat bran, except for cmc1. However, the slight reduction in the cmc1 transcript expression level in clbR-OE might not affect enzyme accumulation in the culture supernatant during the first four days.
Fig. 5. Northern blot analysis of expression of the CAZy genes in MR12 and clbR-OE strains.
Notes: Cells were grown in minimal medium (MM) supplemented with 1% (w/v) glucose at 30 °C for 24 h. Cells were harvested, washed with carbon-free MM, transferred to fresh MM supplemented with 1% (w/v) Avicel (Avi) and 1% (w/v) wheat bran (WB) at time 0, and grown at 30 °C for 6- and 12-h post-induction. Blots were hybridized with specific probes for cbhI, cel7b, cmc2, xynIa, cmc1, and gpdA. gpdA was used as an endogenous control in all samples.
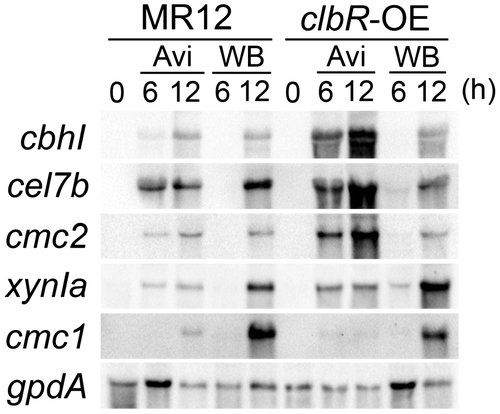
We next investigated how clbR overexpression enabled sustainable enzyme production at the late growth phase; however, we failed to quantify transcripts of clbR and the cellulose-degrading enzyme genes at time points later than 24-h post-induction. This presumably reflects the general difficulties in RNA preparation from well-grown hyphae of A. aculeatus. Together, our data on enzyme activities and the expression profiles of tested genes suggest that ClbR regulates the expression of these genes via multiple signaling pathways in A. aculeatus.
Discussion
In this study, we demonstrate that overexpression of clbR in A. aculeatus enabled sustainably increased production of β-1,4-endoglucanase and β-1,4-xylanase, but not β-glucosidase. A. aculeatus produces various enzymes with different specific activities, such as CbhI, Cel7b, Cmc1, Cmc2, and XynIa. Because the genes encoding the above enzymes are all upregulated by Avicel via a ClbR-dependent signaling pathway in A. aculeatus, we presumed that the expression of their genes would be stimulated in clbR-OE under the wheat bran-inducing condition. However, the effect of wheat bran on clbR-OE varied: production of β-1,4-xylanase, especially XynIa, was drastically increased, whereas that of Cel7b decreased and there was no effect on the other enzymes. Moreover, expression was regulated at the transcriptional level, suggesting that ClbR diversely controls expression of the genes encoding CAZy enzymes in A. aculeatus.
Unlike β-1,4-endoglucanase and β-1,4-xylanase, production of β-glucosidase was not affected by clbR overexpression. Although β-glucosidase was produced in wheat bran-induced culture, transcript analysis of bgl1 (GH3), the major β-glucosidase gene in A. aculeatus, revealed that bgl1 transcripts were not detected in the presence of wheat bran whereas the other genes (e.g., cbhI, cel7b, cmc2, and xynIa) were induced by wheat bran (data not shown). It has been reported that BglR regulates expression of the β-glucosidase gene in T. reesei.Citation21) Therefore, ClbR might not be involved in regulation of the bgl1 expression in A. aculeatus.
A particularly interesting finding of this study is the multiple effects of clbR overexpression in A. aculeatus on the expression of the cellulosic biomass-degrading enzyme genes. One plausible mechanism for this diversity invokes titration among several transcription factors involved in the regulation of these genes. One of the best-understood examples of the complex interplay among zinc cluster proteins involves the regulatory circuit of multiple or pleiotropic drug resistance (PDR) in Saccharomyces cerevisiae. The yeast zinc cluster proteins Pdr1p and Pdr3p bind as homo- or heterodimers to pleiotropic drug response elements in the promoters of the target genes.Citation22) Pdr1p and Pdr3p transduce signals by directly binding to chemically distinct drugs and xenobiotics, such as the antifungal ketoconazole and the translation inhibitor cycloheximide.Citation23) Furthermore, another zinc cluster protein, Stb5p, participates in the regulation of the pleiotropic drug resistance genes. Stb5p dominantly exists as a Pdr1p heterodimer and shows little homodimerization.Citation24) The selection of dimerization partners appears to be a key factor in PDR regulation.
From these facts of complex regulatory circuit with involvement of multiple regulatory factors in S. cerevisiae, there is the possibility that various factors are involved in the regulation. Indeed, several Zn(II)2Cys6 binuclear cluster proteins have been identified as positive regulators controlling the expression of the cellulosic biomass-degrading enzyme genes in filamentous fungi, such as XlnR,Citation3,6,25) AraR,Citation10) ManR/ClrB/Clr-2,Citation8,9,12) and Clr-1.Citation9) Among these, it has been suggested that ClrB cooperatively binds to the cellulose responsive element (CeRE) with McmA, a MADS-box binding protein that regulates cellulolytic genes.Citation26,27) Mcm1p, a McmA homolog in S. cerevisiae, forms homodimers and enables mutual interactions with various transcription factors such as α1, α2, Ste12p, Yox1p, Yhp1p, Fkh2p, Arg80p, and Arg81p, thus regulating different sets of genes depending on the interacting protein.Citation28) It has been reported that interaction with one factor triggers a conformational change of Mcm1p, which impairs interaction with another protein.Citation29)
Although interaction among the identified regulators in A. aculeatus should be further investigated, we hypothesize that ClbR selects a counterpart in response to the inducible carbon source. Under the Avicel-inducing conditions, the effects of cellulosic signals were restricted to stimulation of the cellulolytic-inductive transcription factors including ClbR, thus overexpression of clbR resulted in stimulation of the regulated gene expression. However, under the wheat bran-inducing condition, multiple signals from wheat bran may activate various transcription factors involved in different signaling pathways, and the activated transcription factors might scramble to compete for each transcription factor, including ClbR. This would cause transcription factor titration, resulting in diverse effects of clbR overexpression. The reduced hyphal growth of the clbR-OE strain might be explained by scrambling of other transcription factors involved in hyphal growth, although the stimulus is currently unknown.
β-1,4-xylanase activity was drastically increased in clbR-OE because of a marked increase in xynIa transcription in the overexpressing strain. ClbR may be a principle transcription factor in this response because XlnR does not regulate xynIa expression in A. aculeatus,Citation6) although the expression of xynF3, the xynIa homolog in A. oryzae, is controlled by XlnR.Citation30) Therefore, the effect of clbR overexpression could directly account for xynIa expression. Furthermore, the properties of major extracellular β-1,4-xylanases in A. aculeatus could be critical for the increased activity of β-1,4-xylanases. Two β-1,4-xylanases, designated XynIa and XynIb, have been purified and characterized from A. aculeatus culture supernatant. The expression level of xynIb is higher than that of xynIa,Citation6,11) although it seems that XynIa is a dominant β-1,4-xylanase in acidic culture supernatant of A. aculeatus because XynIa and XynIb are stable between pH 3.0–9.0 and pH 5.0–7.0, respectively (unpublished data). Therefore, an increase in the dominant β-1,4-xylanase, XynIa, appears to be critical for enhanced xylanase activity in A. aculeatus.
Because Aspergillus possesses more paralogs of zinc cluster proteins than Trichoderma and Neurospora,Citation31) gene duplication followed by mutations may contribute to the establishment of complex signaling pathways to respond to various stimuli from the natural plant biomass in Aspergillus. The logical next step is to identify factors that interact with ClbR or whose gene expression is cooperatively controlled with ClbR.
Additional information
Funding
References
- Murao S, Kanamoto J, Arai M. Isolation and identification of a cellulolytic enzyme producing microorganism. J. Ferment. Technol. 1979;57:151–156.
- van Peij N, Visser J, de Graaff L. Isolation and analysis of xlnR, encoding a transcriptional activator co-ordinating xylanolytic expression in Aspergillus niger. Mol Microbiol. 1998;27:131–142.10.1046/j.1365-2958.1998.00666.x
- van Peij N, Gielkens M, de Vries R, Visser J, de Graaff L. The transcriptional activator XlnR regulates both xylanolytic and endoglucanase gene expression in Aspergillus niger. Appl. Environ. Microbiol. 1998;64:3615–3619.
- Marui J, Kitamoto N, Kato M, Kobayashi T, Tsukagoshi N. Transcriptional activator, AoXlnR, mediates cellulose-inductive expression of the xylanolytic and cellulolytic genes in Aspergillus oryzae. FEBS Lett. 2002;528:279–282.10.1016/S0014-5793(02)03328-8
- Endo Y, Yokoyama M, Morimoto M, Shirai K, Chikamatsu G, Kato N, Tsukagoshi N, Kato M, Kobayashi T. Novel promoter sequence required for inductive expression of the Aspergillus nidulans endoglucanase gene eglA. Biosci. Biotechnol. Biochem. 2008;72:312–320.10.1271/bbb.70278
- Tani S, Kanamasa S, Sumitani J, Arai M, Kawaguchi T. XlnR-independent signaling pathway regulates both cellulase and xylanase genes in response to cellobiose in Aspergillus aculeatus. Curr. Genet. 2012;58:93–104.10.1007/s00294-012-0367-5
- Brunner K, Lichtenauer AM, Kratochwill K, Delic M, Mach RL. Xyr1 regulates xylanase but not cellulase formation in the head blight fungus Fusarium graminearum. Curr. Genet. 2007;52:213–220.10.1007/s00294-007-0154-x
- Ogawa M, Kobayashi T, Koyama Y. ManR, a novel Zn(II)2Cys6 transcriptional activator, controls the β-mannan utilization system in Aspergillus oryzae. Fungal Genet. Biol. 2012;49:987–995.10.1016/j.fgb.2012.09.006
- Coradetti S, Craig J, Xiong Y, Shock T, Tian C, Glass N. Conserved and essential transcription factors for cellulase gene expression in ascomycete fungi. Proc. Nat. Acad. Sci. U.S.A. 2012;109:7397–7402.10.1073/pnas.1200785109
- Battaglia E, Hansen SF, Leendertse A, Madrid S, Mulder H, Nikolaev I, de Vries RP. Regulation of pentose utilisation by AraR, but not XlnR, differs in Aspergillus nidulans and Aspergillus niger. Appl. Microbiol. Biotechnol. 2011;91:387–397.10.1007/s00253-011-3242-2
- Kunitake E, Tani S, Sumitani J, Kawaguchi T. A novel transcriptional regulator, ClbR, controls the cellobiose- and cellulose-responsive induction of cellulase and xylanase genes regulated by two distinct signaling pathways in Aspergillus aculeatus. Appl. Microbiol. Biotechnol. 2013;97:2017–2028.10.1007/s00253-012-4305-8
- Ogawa M, Kobayashi T, Koyama Y. ManR, a transcriptional regulator of the beta-mannan utilization system, controls the cellulose utilization system in Aspergillus oryzae. Biosci. Biotechnol. Biochem. 2013;77:426–429.
- Kunitake E, Tani S, Sumitani J, Kawaguchi T. Agrobacterium tumefaciens-mediated transformation of Aspergillus aculeatus for insertional mutagenesis. AMB Express. 2011;1:46.10.1186/2191-0855-1-46
- Tani S, Tsuji A, Kunitake E, Sumitani J, Kawaguchi T. Reversible impairment of the ku80 gene by a recyclable marker in Aspergillus aculeatus. AMB Express. 2013;3:4.10.1186/2191-0855-3-4
- Adachi H, Tani S, Kanamasa S, Sumitani J, Kawaguchi T. Development of a homologous transformation system for Aspergillus aculeatus based on the sC gene encoding ATP-Sulfurylase. Biosci. Biotechnol. Biochem. 2009;73:1197–1199.10.1271/bbb.80772
- Shevchenko A, Tomas H, Havlis J, Olsen JV, Mann M. In-gel digestion for mass spectrometric characterization of proteins and proteomes. Nat. Protoc. 2006;1:2856–2860.
- Chang AK, Kim HY, Park JE, Acharya P, Park IS, Yoon SM, You HJ, Hahm KS, Park JK, Lee JS. Vibrio vulnificus secretes a broad-specificity metalloprotease capable of interfering with blood homeostasis through prothrombin activation and fibrinolysis. J. Bacteriol. 2005;187:6909–6916.10.1128/JB.187.20.6909-6916.2005
- Reichard U, Monod M, Odds F, Rüchel R. Virulence of an aspergillopepsin-deficient mutant of Aspergillus fumigatus and evidence for another aspartic proteinase linked to the fungal cell wall. J. Med. Vet. Mycol. 1997;35:189–196.10.1080/02681219780001131
- Mattern IE, van Noort JM, van den Berg P, Archer DB, Roberts IN, van den Hondel CA. Isolation and characterization of mutants of Aspergillus niger deficient in extracellular proteases. Mol. Gen. Genet. 1992;234:332–336.10.1007/BF00283855
- Zhu LY, Nguyen CH, Sato T, Takeuchi M. Analysis of secreted proteins during conidial germination of Aspergillus oryzae RIB40. Biosci. Biotechnol. Biochem. 2004;68:2607–2612.10.1271/bbb.68.2607
- Nitta M, Furukawa T, Shida Y, Mori K, Kuhara S, Morikawa Y, Ogasawara W. A new Zn(II)2Cys6-type transcription factor BglR regulates β-glucosidase expression in Trichoderma reesei. Fungal Genet. Biol. 2012;49:388–397.10.1016/j.fgb.2012.02.009
- Mamnun YM, Pandjaitan R, Mahé Y, Delahodde A, Kuchler K. The yeast zinc finger regulators Pdr1p and Pdr3p control pleiotropic drug resistance (PDR) as homo- and heterodimers in vivo. Mol. Microbiol. 2002;46:1429–1440.10.1046/j.1365-2958.2002.03262.x
- Thakur JK, Arthanari H, Yang F, Pan SJ, Fan X, Breger J, Frueh DP, Gulshan K, Li DK, Mylonakis E, Struhl K, Moye-Rowley WS, Cormack BP, Wagner G, Näär AM. A nuclear receptor-like pathway regulating multidrug resistance in fungi. Nature. 2008;452:604–609.10.1038/nature06836
- Akache B, MacPherson S, Sylvain MA, Turcotte B. Complex interplay among regulators of drug resistance genes in Saccharomyces cerevisiae. J. Biol. Chem. 2004;279:27855–27860.10.1074/jbc.M403487200
- Marui J, Tanaka A, Mimura S, de Graaff LH, Visser J, Kitamoto N, Kato M, Kobayashi T, Tsukagoshi N. A transcriptional activator, AoXlnR, controls the expression of genes encoding xylanolytic enzymes in Aspergillus oryzae. Fungal Genet. Biol. 2002;35:157–169.10.1006/fgbi.2001.1321
- Yamakawa Y, Endo Y, Li N, Yoshizawa M, Aoyama M, Watanabe A, Kanamaru K, Kato M, Kobayashi T. Regulation of cellulolytic genes by McmA, the SRF-MADS box protein in Aspergillus nidulans. Biochem. Biophys. Res. Commun. 2013;431:777–782.10.1016/j.bbrc.2013.01.031
- Kobayashi T. Cooperative regulation of Aspergillus nidulans cellulase genes by transcription factors McmA and ManR/ClrB. Poster session presented at the 27th Fungal Genetics Conference; 2013 Mar 14; Asilomar, CA.
- Messenguy F, Dubois E. Role of MADS box proteins and their cofactors in combinatorial control of gene expression and cell development. Gene. 2003;316:1–21.10.1016/S0378-1119(03)00747-9
- Darieva Z, Clancy A, Bulmer R, Williams E, Pic-Taylor A, Morgan B, Sharrocks A. A competitive transcription factor binding mechanism determines the timing of late cell cycle-dependent gene expression. Mol. Cell. 2010;38:29–40.10.1016/j.molcel.2010.02.030
- Noguchi Y, Sano M, Kanamaru K, Ko T, Takeuchi M, Kato M, Kobayashi T. Genes regulated by AoXlnR, the xylanolytic and cellulolytic transcriptional regulator, in Aspergillus oryzae. Appl. Microbiol. Biotechnol. 2009;85:141–154.10.1007/s00253-009-2236-9
- Tani S, Kawaguchi T, Kobayashi T. Complex regulation of hydrolytic enzyme genes for cellulosic biomass degradation in filamentous fungi. Appl. Microbiol. Biotechnol. 2014;98:4829–4837.10.1007/s00253-014-5707-6