Abstract
Nudix hydrolases are widely distributed in all kingdoms of life and have the potential to hydrolyze a wide range of organic pyrophosphates, including nucleoside di- and triphosphates, nucleotide coenzymes, nucleotide sugars, and RNA caps. However, except for E. coli MutT and its orthologs in other organisms that sanitize oxidized nucleotides to prevent DNA and RNA mutations, the functions of Nudix hydrolases had largely remained unclear until recently, because many members of this enzyme family exhibited broad substrate specificities. There is now increasing evidence to show that their functions extend into many aspects of the regulation of cellular responses. This review summarizes current knowledge on the molecular and enzymatic properties as well as physiological functions of Arabidopsis Nudix hydrolases. The information presented here may provide novel insights into the physiological roles of these enzymes in not only plant species, but also other organisms.
Graphical Abstract
Arabidopsis Nudix hudrolases act on the hydrolysis of diverse biomolecules and play versatile physiological functions. SA, salicylic acid; PAR, poly(ADP-ribosyl)ation.
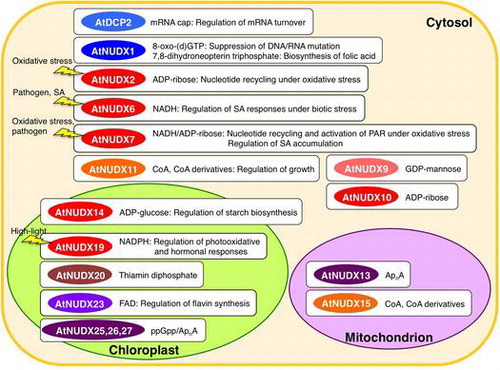
Since the 1960s, pyrophosphohydrolase activities toward several essential coenzymes, such as NAD(P)H, FAD, and coenzyme A (CoA), as well as various types of nucleotide sugars and diadenosine polyphosphates (ApnA), have been detected in extracts prepared from various types of organisms including bacteria, animals, and plants. However, the molecular properties and physiological roles of these enzymes remained unclear until the 1990s.
The Nudix hydrolase (NUDX)(PDOC00695, PROSITE, http://prosite.expasy.org) is a pyrophosphohydrolase that acts on substrates with the general structure NDP-X (Nucleoside diphosphate linked to some moiety, X) to yield nucleoside monophosphate (NMP) plus phosphate-X (P-X) as its products.Citation1,2) Various cellular compounds include the structure NDP-X and also contain nucleoside di- and triphosphates (dNDP and dNTP, both canonical and oxidized derivatives), nucleotide sugars and alcohols, dinucleoside polyphosphates (NpnN), dinucleotide coenzymes, and RNA caps. These compounds are potentially toxic signaling molecules, metabolic intermediates, or coenzymes, the levels of which must be properly modulated. Therefore, Nudix hydrolases were originally proposed to function in housecleaning to eliminate excess toxic metabolites or controlling the availability of intermediates in metabolic pathways.Citation1,2) Some non-nucleotide molecules have also been identified as substrates for NUDXs, and other relevant substrates may also exist.
NUDXs are a diverse superfamily of proteins defined by a conserved amino acid sequence termed the Nudix box or Nudix motif, represented by GX5EX7REUXEEXGU, in which U depicts an aliphatic, hydrophobic residue, such as isoleucine, leucine, or valine, and X is any amino acid (PS00893, PROSITE) (Fig. ).Citation1) The Nudix motif functions as an essential catalytic site of the enzyme and the glutamine residues in the core sequences, REUXEE, play an important role in binding essential divalent cations, such as Mg2+ and Mn2+, as a cofactor.Citation2) Additional sequences associated with this motif affect the substrate specificity of the enzyme as described below. The Nudix motif is shared not only by the NUDX family, but also the isopentenyl diphosphate (IPP) isomerases, MutY-type DNA glycosylases, a subunit of a cleavage factor required for RNA processing (NUDT21), and a cation channel (TRPM2/NUDT9L1), suggesting a common evolutionary ancestor.Citation2)
Fig. 1. Alignment of partial amino acid sequences surrounding the Nudix motifs in AtNUDXs.
Note: The preferred substrate is indicated in the left side.
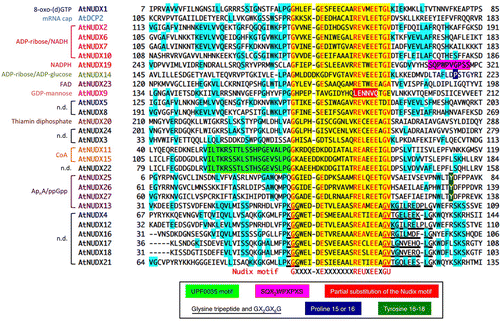
The molecular and enzymatic properties as well as physiological roles of NUDXs in various organisms including bacteria, yeast, algae, and vertebrates have been revealed and summarized in previous reviews.Citation1–3) Of these, E. coli MutT and its orthologs in other organisms have been shown to function as antimutators; oxidized nucleotides in the nucleotide pools of cells are sanitized to avoid DNA and RNA mutations.Citation4,5) It is now clear that their functions extend into many aspects of metabolic regulation, although the functions of many NUDXs have not yet been clarified. However, information on the properties and functions of NUDXs in a model plant, Arabidopsis, has been intensively uncovered in the past decade. This review summarizes recent findings on NUDXs in Arabidopsis.
I. Molecular and enzymatic properties of NUDXs in Arabidopsis
1. The number of NUDX genes in Arabidopsis
NUDXs are widespread in all three domains—the Archaea, Bacteria, and Eukarya. Bioinformatics searches revealed that thousands of open reading frames could potentially encode the NUDX proteins of more than 300 species.Citation6,7) E. coli, Deinococcus radiodurans, Bacillus cereus, Saccharomyces cerevisiae, and humans have 13, 21, 26, 7, and 24 genes, respectively, that encode NUDXs.Citation2,8,9) Some of the NUDX genes in humans have been shown to produce more than one mRNA variant, which encodes a protein with a different subcellular localization and/or substrate specificity, by alternative splicing events.
A recent database search of sequenced genomes led to the identification of NUDX genes in plant species. KraszawskaCitation6) reported that there are 33, 53, and 30 genes encoding NUDXs in Oryza sativa, Populus trichocarpa, and Vitis vinifera, respectively. We identified 32 of these genes in Solanum lycopersicum (Tomato SBM database, http://www.kazusa.or.jp/tomato_sbm/). The numbers of NUDX genes must be carefully determined in an organism, because of the relatively low identities and similarities in the amino acid sequences of NUDXs. Our database search revealed that the number of genes encoding NUDXs was 20 in O. sativa (Rice TOGO Browser, http://agri-trait.dna.affrc.go.jp/) (Table ). The differences observed in these numbers from those reported by KraszawskaCitation6) may depend on the existence of putative alternative splicing variants and/or the inclusion of another homologous protein family such as IPP isomerase.
Table 1. Summary of AtNUDX genes and enzymes.
Differences have also been reported in the numbers of NUDX genes in Arabidopsis. We initially reported that 24 genes encoding NUDXs in Arabidopsis were registered in the National Center for Biotechnology Information DataBase (www.ncbi.nlm.nih.gov/).Citation10) Muñoz et al.Citation11) and KraszewskaCitation6) subsequently showed that there are 31 and 29 NUDX genes, respectively, in Arabidopsis (Table ). However, two genes (At5g16440 and At3g02780) encoding IPP isomerases, which lacked the Nudix motif and, thus, had no activity as the NUDX enzyme, and one gene (At2g04440) encoding a non-NUDX protein with moderate similarities to the other NUDXs and without most of the Nudix motif were included as members. Therefore, we concluded that Arabidopsis possessed 28 NUDX genes (Table and Fig. ).Citation12) Of these, the protein encoded by At5g13570 has been characterized as an mRNA-decapping enzyme; therefore, the gene has been named AtDCP2.Citation13–15) Regarding the remaining 27 NUDX genes, we initially named them “AtNUDT”.Citation10) Thereafter, we renamed them “AtNUDX” because the same number between AtNUDT and human NUDT did not indicate that the Arabidopsis gene was the closest ortholog of the human gene (Table ).Citation12)
Among the AtNUDX genes, the AtNUDX15 gene was shown to produce two types of intron retention-type alternatively spliced variants (named AtNUDX15 and AtNUDX15a). The AtNUDX15 and AtNUDX15a mRNAs were predicted to encode distinct proteins that differed by the C-terminal regions due to the usage of alternative stop codons (Table ). The genes encoding AtNUDX7, 10, 11, 13, 16, 24, and 25, as well as AtDCP2 were also registered to produce ≥2 types of intron retention-type alternatively spliced variants, although the expression of their variants in cells under physiological conditions has yet to be confirmed (TAIR, https://www.arabidopsis.org/).
The predicted molecular weights of AtNUDXs ranged from 16.4 (AtNUDX1) to 86.9 kDa (AtNUDX3) (Table ). The largest one, AtNUDX3 contained the domain for the Peptidase_M49 super family (accession no. cl20254, The Conserved Domain Database, http://www.ncbi.nlm.nih.gov/cdd) at the C-terminus, although its impact on the action of the protein currently remains unknown.
AtNUDXs can be classified into three types according to their predicted subcellular localizations (TargetP, http://www.cbs.dtu.dk/services/TargetP/; PSORT, http://psort.ims.u-tokyo.ac.jp/): the cytosol (AtNUDX1 to -11 and 25, and AtDCP2), mitochondrion (AtNUDX12 to −18), or chloroplast (AtNUDX19 to −24, 26, and 27) (Table ).Citation10,12,16) The prediction program for subcellular localization showed that such organelle-type AtNUDXs had either mitochondrial or chloroplastic transit peptides at their N-terminus. The alternatively spliced variant of AtNUDX15, AtNUDX15a, had the type-1 peroxisomal targeting signal (PTS1) at the C-terminus, in addition to the mitochondrial transit peptide at the N-terminus, suggesting a dual subcellular distribution in mitochondria and peroxisomes.Citation17)
2. Preferred substrates for AtNUDXs
To date, the in vitro activities of all AtNUDXs toward various substrates with the structure NDP-X have been tested using recombinant proteins produced by E. coli. In these analyses, the activity of organelle-type AtNUDXs was assayed using the recombinant protein in the absence of the predicted transit peptide.Citation12) An alkaline pH and the presence of divalent cations, such as Mg2+ and Mn2+, were found to be necessary for the activity of typical NUDXs. In most cases, Mg2+ most effectively induced the activities of NUDXs and this is believed to be relevant to their physiological actions.Citation1,2) Dobrzanska et al.Citation18) reported that AtNUDX1 hydrolyzed NADH; however, this activity was negligible at physiological levels of Mn2+ as a cofactor (Table ). Klaus et al.Citation19) demonstrated that AtNUDX1 preferred dihydroneopterin triphosphate, an intermediate in the folate biosynthetic pathway, and (d)NTP to NADH in the presence of Mg2+, suggesting an involvement of the enzyme in the pathway. Similar to E. coli MutT and human MTH1, which are known as the sanitization enzymes of oxidized nucleotides, we subsequently found that 8-oxo-7, 8-dihydro-2′-deoxy GTP (8-oxo-dGTP) was the most favored substrate for AtNUDX1 in the presence of Mg2+ (Table ).Citation10) AtNUDX1 could also hydrolyze an oxidized ribonucleotide, 8-oxo-GTP, with high affinity (Km), although the enzyme was also active toward canonical dNTPs.Citation16)
AtNUDX2, 6, and 7 could hydrolyze both ADP-ribose and NADH to yield AMP and ribose 5-phosphate, and AMP and reduced nicotinamide mononucleotide (NMNH), respectively, with almost equal catalytic efficiencies (kcat/Km) (Table ).Citation10,20,21) On the other hand, AtNUDX10 preferred ADP-ribose over NADH. Although AtNUDX14 hydrolyzed both ADP-glucose and ADP-ribose, its affinity was nearly 100-fold higher toward ADP-ribose than ADP-glucose.Citation11,12) KraszewskaCitation6) summarized present knowledge on the additional conserved regions identified in some NUDXs. A proline residue downstream of the Nudix motif, which is characteristic of NUDX exhibiting pyrophosphohydrolase activity toward ADP-ribose, was detected in the sequence of AtNUDX14, but not in that of AtNUDX2, 6, 7, or 10 (Table and Fig. ). In addition, AtNUDX14 as well as its closest homolog in rice (Os06g0129700) and Solanum tuberosum (AM180509, EMBL database) contained a modified Nudix motif; a substitution of the conserved glutamate at position 7.Citation11) A mutational analysis of AtNUDX7 revealed that the residues (phenylalanine 73 and valine 168) were required for dimer formation and its activity.Citation22)
Although AtNUDX19 had the sequence, SQX2WPXPXS, which is characteristic of the NADH pyrophosphohydrolase (Table and Fig. ),Citation6) the enzyme preferred NADPH over NADH with nearly 10-fold higher affinity.Citation12) AtNUDX23 hydrolyzed FAD to FMN and AMP. AtNUDX11, 15, and 22 had the sequence motif, LLTXR[SA]X3RX3GX3FPGG (UPF0035, PROSITE), characteristic of the CoA pyrophosphohydrolase (Table and Fig. ).Citation6) CoA was hydrolyzed to 4′-phosphopantetheine and adenosine-3′, 5′-bisphosphate (3′, 5′-ADP) by the hydrolysis reaction of AtNUDX11, 15, and 15a, but not AtNUDX22. These enzymes were active toward not only CoA, but also its derivatives such as succinyl-CoA, malonyl-CoA, lauroyl-CoA, and myristoyl-CoA, and its oxidized form (Oxidized CoA).Citation10,12,17) AtNUDX15 preferred such CoA derivatives over CoA, although its alternatively spliced variant, AtNUDX15a, preferred CoA over CoA derivatives.
Many AtNUDXs have been shown to exhibit ApnA pyrophosphohydrolase activity, by which ATP is always produced as a product (Table ). A previous study demonstrated that AtNUDX13 was active toward long-chain ApnA (n = 5 and 6).Citation23) A tyrosine residue downstream of the Nudix motif found in the other Ap4A pyrophosphohydrolases was conserved in AtNUDX25-27 (Table and Fig. ). AtNUDX25 and AtNUDX26 hydrolyzed both Ap4A and Ap5A with similar affinities, whereas AtNUDX27 preferred Ap5A.Citation12,16) Similar to Thermus thermophilus NUDX (Ndx8), AtNUDX11, 15, 25, and 26 exhibited pyrophosphohydrolase activity toward both diphosphate linkages in guanosine-3′,5′-tetraphosphate (ppGpp), yielding guanosine 3′,5′-bisphosphate (pGp) as the final product.Citation24) Of these, AtNUDX26 showed the highest catalytic efficiency. We recently found that, similar to E. coli orf1.9, AtNUDX9, whose Nudix motif is partially substituted (Fig. ), was active toward GDP-mannose, yielding mannose-1-phosphate and GMP (Yoshimura et al. unpublished data) (Table ). AtNUDX20 was found to hydrolyze not only (d)NTP but also thiamin diphosphate and its toxic form, the diphosphates of oxothiamin and oxythiamin, in the presence of Mn2+.Citation25)
AtDCP2 specifically hydrolyzed capped mRNA to produce 7-methyl GDP and decapped (5′-phosphorylated) RNA.Citation13–15) A mutation in conserved glutamate 154 and 158 in the Nudix motif in AtDCP2 reduced its activity by up to 400-fold (Fig. ).Citation15)
AtNUDX3–5, 8, 12, 16–18, 21, 22, and 24 were incapable of hydrolyzing any of the classical NUDX substrates. Glycine tripeptide and GX2GX6G, characteristic of the ApnA pyrophosphohydrolase, were closely located upstream and downstream, respectively, of the Nudix motif in AtNUDX4, 16–18, and 21 (Table and Fig. ). AtNUDX20 and AtNUDX24 lacked the arginine residue in the Nudix motif, suggesting the importance of the residue in their activities as NUDX enzymes.
Olejnik et al.Citation23) reported that AtNUDX13 displayed ApnA pyrophosphohydrolase activity in the presence of dithiothreitol (DTT). Reducing conditions also increased the activities of AtNUDX26 and AtNUDX27, whereas that of AtNUDX15 was markedly decreased.Citation12) These findings suggested that reducing conditions may be essential for ApnA pyrophosphohydrolases. However, even under reducing conditions, no activity was detected in AtNUDX3–5, 8, 12, 16–18, 21, 22, or 24 toward any of the substrates tested.Citation12)
In conclusion, AtNUDXs can be divided into eight subfamilies based on their preferred substrate (Table ) as follows; (1) 8-oxo-(d)GTP, AtNUDX1; (2) ADP-ribose/ NAD(P)H, AtNUDX2, 6, 7, 10, 14, and 19; (3) GDP-mannose, AtNUDX9; (4) CoA, AtNUDX11 and 15; (5) ApnA/ppGpp, AtNUDX13, 25-27; (6) thiamin diphosphate, AtNUDX20; (7) FAD, AtNUDX23; (8) mRNA cap, AtDCP2. The spectrum of activities of the respective subfamilies covers almost all of the bacterial and animal enzymes.Citation1,2,8) In addition, most of the subfamilies include multiple AtNUDXs. These findings suggest that plants have amplified and developed NUDX genes in each subfamily to adapt their physiology. A phylogenetic analysis (MEGA 6.06 software) showed that almost all members in each subfamily were clustered in the monophyletic group (Fig. ), suggesting that the genes encoding AtNUDXs with the same substrate specificity may have been duplicated from a common evolutionary origin by recent segmental duplication events. In addition, most AtNUDX subfamilies have at least one homolog of rice NUDXs (Fig. ). Therefore, it is likely that the subfamilies of NUDXs are commonly conserved in both mono- and dicotyledonous plants, and appeared prior to the evolutionary branch point of plant species from other organisms.
Fig. 2. The phylogenetic tree of NUDXs from Arabidopsis and rice.
Note: The tree was drawn using MEGA 6.06 software to depict the phylogenetic relationships between each subfamily of AtNUDXs and rice NUDXs. The preferred substrate is indicated. The scale bar corresponds to the branch length and shows 0.2 amino acid substitutions per site.
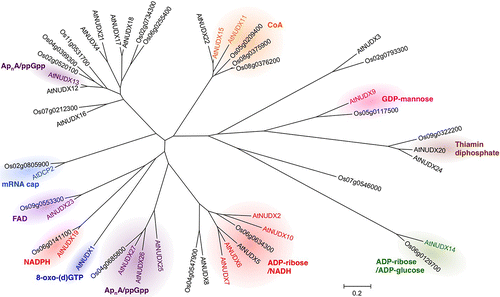
3. Possible factors modulating AtNUDX activity
Similar to NUDXs in the other organisms, AtNUDXs can hydrolyze a broad spectrum of substrates with varying degrees of substrate specificity. Therefore, careful consideration is needed when speculating about and demonstrating the physiological roles of respective enzymes. Namely, sometimes the defined enzymatic characteristics of NUDXs do not reflect their physiological functions. For example, the availability of substrates and cofactors must be limited, and changes depend on various internal and external factors, e.g. differences in the subcellular location, types of tissues, developmental stages, and environmental conditions.
Considering the typical cytosolic concentrations of Mg2+ (1 mM) in plants,Citation19) AtNUDXs can be activated by certain concentrations of Mg2+. However, the activity of AtNUDX15 in the presence of 5 mM Mn2+, which was estimated to be >103-fold greater than the typical levels in cells, were more than 2-fold higher than that in the presence of 5 mM Mg2+.Citation12) On the other hand, Zn2+ was the most effective divalent ion for AtNUDX23 toward FAD. AtNUDX23 activity was more than 4-fold higher in the presence of 5 mM Zn2+ than in the presence of 5 mM Mg2+. The hydrolase activity of AtNUDX7 was markedly inhibited in the presence of Ca2+.Citation26) These findings suggested that the actions of some AtNUDXs may be regulated by the flux of divalent cations in subcellular compartments, in which each AtNUDX was localized.
II. Physiological roles of AtNUDXs
1. Sanitization of oxidized nucleotides
Cellular DNA, RNA, and their precursor nucleotides are at high risk of being oxidized by reactive oxygen species (ROS). Of the various types of oxidized damage to nucleic acids, 8-oxo-7, 8-dihydro-guanine (8-oxo-G) in DNA or the free nucleotide form was found to be one of the most predominant.Citation27,28) 8-Oxo-G-containing nucleotide, 8-oxo-(d)GTP, can be incorporated into DNA as well as RNA, causing both replicational and transcriptional errors, because the base can pair with not only cytosine, but also adenine with almost the same efficiency.Citation29,30) The Arabidopsis 8-oxo-(d)GTP pyrophosphohydrolase, AtNUDX1 was shown to be distributed in the cytosol, in which the main pool of nucleotides existed in cells (Table ).Citation16) Among all AtNUDXs, only AtNUDX1 completely suppressed the increased frequency of both DNA mutations and transcriptional errors in the E. coli MutT-deficient strain, suggesting that AtNUDX1 may be a functional homolog of E. coli MutT in Arabidopsis.Citation10,12,16) Knockout (KO)-nudx1 plants did not show any discernible phenotype under normal or oxidative stress (3 μM paraquat, PQ, an agent producing O2−) conditions; however, the levels of 8-oxo-G in the genomic DNA of KO-nudx1 plants were significantly higher than those of wild-type plants under both conditions.Citation16) These results indicated that AtNUDX1 functioned in cellular defense against oxidative DNA and RNA damage through the sanitization of their precursor pools in the cytosol in Arabidopsis cells.
Although AtNUDX1 is the only MutT-type NUDX in Arabidopsis, mammalian cells have developed multiple MutT-type enzymes (MTH1, MTH2, MTH3, and NUDT5) that exhibit pyrophosphohydrolase activity with differences in substrate specificity to various types of oxidized nucleotides in the cytosol, mitochondria, and nucleus.Citation31–36) When the human MutT-type NUDX, hMTH1, was ectopically expressed in the chloroplasts (chl-hMTH1) and mitochondria (mit-hMTH1) of Arabidopsis cells, both plants exhibited higher tolerance to oxidative stress caused by heating and the PQ treatment than that of control plants and plants ectopically expressing hMTH1 in the cytosol.Citation37) The poly(ADP-ribosyl)ation (PAR) reaction is a posttranslational modification that regulates many biological processes including DNA repair, epigenetic regulation, and transcription, and is known to lead cells either toward the repair of DNA or programmed cell death, depending on the severity of the damage induced.Citation38,39) Activation of the PAR reaction and suppression of DNA fragmentation, which leads to programmed cell death, were observed in mit-hMTH1 and chl-hMTH1 plants. These findings suggested that the accumulation of oxidized nucleotides and/or oxidized bases in chloroplasts and mitochondria may trigger programmed cell death in plant cells under oxidative stress. Therefore, improving defense mechanisms against oxidatively-induced DNA damage in organelles could provide a powerful strategy to enhance the tolerance of plants to environmental stress.
2. Regulation of abiotic stress responses
Six AtNUDXs exhibit pyrophosphohydrolase activity toward ADP-ribose and/or NAD(P)H (Table ), suggesting the importance of the metabolic regulation of ADP-ribose and NAD(P)H in plant cells. Of these, the distribution of AtNUDX14 and AtNUDX19 in chloroplasts was confirmed using GFP fusion proteins at their C-terminus, although the former was predicted to be localized in mitochondria.Citation12) AtNUDX2, 6, 7, and 10 are cytosolic enzymes, because their amino acid sequences do not contain any transit peptide.
Nicotinamide adenine dinucleotide (NAD+) and its derivative NADP+ are indispensable cofactors that orchestrate cellular redox homeostasis in all living organisms. In addition to their fundamental functions in hundreds of cellular redox reactions, these compounds are required for the regulation of complex cellular processes, including transcriptional regulation and microtubule metabolism via NAD+-dependent deacetylation and/or mono/poly(ADP-ribosyl)ation as well as intracellular Ca2+ signaling.Citation40–43) On the other hand, ADP-ribose is a potentially toxic product of NAD+ catabolism because it is able to non-enzymatically induce the glycation of the protein N-terminal and lysyl amino groups and cysteinyl thiols, leading to the loss of function.Citation44–47)
The AtNUDX2 gene was originally identified as the gene responsible for the phenotype of increased tolerance to oxidative stress caused by PQ treatments when Arabidopsis activation-tagged lines were screened.Citation48) Based on differences in the amounts of ADP-ribose and NADH as well as the activities of pyrophosphohydrolase toward both molecules in the leaves of transgenic Arabidopsis plants overexpressing and suppressing the AtNUDX2 gene, it was revealed that the enzyme acted on the hydrolysis of ADP-ribose, but not NADH in vivo, and this may be attributed to a posttranslational protein modification. Importantly, the depletion of NAD+ and ATP, which causes cell death, from activation of the PAR reaction under oxidative stress was significantly suppressed by overexpression of the AtNUDX2 gene. These findings indicated that AtNUDX2 functioned in the maintenance of NAD+ and ATP levels by nucleotide recycling from the free ADP-ribose molecules produced by the degradation of poly(ADP-ribose) polymers in order to prevent excess stimulation by the PAR reaction under oxidative stress conditions (Fig. ).Citation48) However, the action of endogenous AtNUDX2 may not markedly contribute to cellular defense systems in nature because of its low expression level even under stress conditions.
Fig. 3. A proposed model for the regulation of biotic and abiotic stress responses by AtNUDX6 and AtNUDX7.
Note: Solid and dotted arrows indicate direct and indirect actions, respectively. The PAR reaction is enhanced by the activation of PARPs and PARGs caused by DNA damages under abiotic stress.Citation54) Hyperactivation of the PAR reaction causes the production of highly reactive free ADP-ribose and the overconsumption of NAD+ and ATP. The reaction products AMP and ribose 5-P of the AtNUDX2 and 7 might be utilized for nucleotide recyclingCitation82), leading to suppression of the overconsumption of NAD+ and ATP under the stress.Citation48,49) AtNUDX7 is also involved in modulation of the PAR reaction and suppression of the excess accumulation of SA, production of which is activated by the EDS1 signaling under biotic stress.Citation49) AtNUDX6 is involved in regulation of the NPR1-dependent SA signaling pathways through induction of the TRX-h5 expression in response to biotic stress.Citation57) The modulation of intracellular NADH levels by AtNUDX6 and 7 might be important in these biotic and abiotic stress responses.Citation51) NMNH, nicotinamide mononucleotide; PARP, poly(ADP-ribose) polymerase; PARG, poly(ADP- ribose) glycohydrolase; ROS, reactive oxygen species; ribose 5-P, ribose 5-posphate.
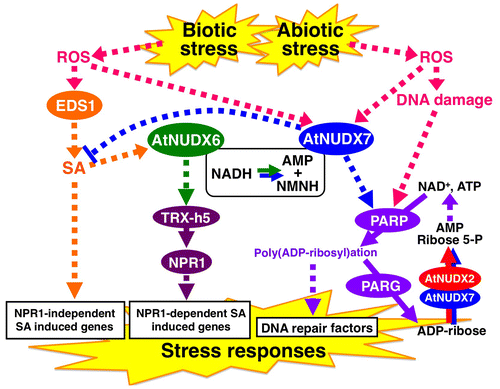
AtNUDX7 was initially identified as Arabidopsis growth factor gene 1 (AtGFG1), which shows high homology to the mammalian GFG gene encoded by antisense fibroblast growth factor (FGF-2); however, any functional link between AtNUDX7 and the mammalian GFG protein has yet to be found.Citation20,21) Using AtNUDX7-overexpressing and KO-nudx7 plants, AtNUDX7 was found to hydrolyze both ADP-ribose and NADH as physiological substrates,Citation49) whereas Ge et al.Citation26) reported that the enzyme preferred NADH over ADP-ribose in vivo. The expression of AtNUDX7 was induced by various types of abiotic stresses, such as drought, salinity, wounding, and high light, as well as various oxidative treatments, such as PQ, ozone, O2−, and H2O2.Citation21,49–52) Overexpression and KO of the AtNUDX7 gene caused increased and decreased tolerance, respectively, to oxidative stress.Citation26,49) In AtNUDX7-overexpressing plants, but not in AtNUDX6-overexpressing plants, the PAR reaction was hyperactivated under oxidative stress while the depletion of NAD+ and ATP due to the activation of the PAR reaction was completely suppressed (Fig. ).Citation49) These findings suggested that, similar to AtNUDX2, AtNUDX7 served to maintain NAD+ levels by supplying ATP via nucleotide recycling from free ADP-ribose molecules. In addition, AtNUDX7 was shown to be involved in the regulation of defense mechanisms against oxidative DNA damage by modulating the PAR reaction (Fig. ).
The expression of AtNUDX19, the NADPH pyrophosphohydrolase in chloroplasts, was markedly induced by high light irradiation. A recent study demonstrated that KO of the AtNUDX19 gene enhanced photosynthetic activity and oxidative stress tolerance (Yoshimura et al., unpublished data). On the other hand, KO-nudx19 plants showed altered hormonal responses, such as hypersensitivity to salicylic acid (SA) and insensitivity to jasmonic acid (JA) and abscisic acid (ABA), which are known to act antagonistically with SA. These findings suggested that AtNUDX19 may harmonize the regulation of oxidative and hormonal responses by modulating NADPH levels in chloroplasts.
3. Regulation of biotic stress responses
There is increasing evidence to indicate that AtNUDX7 and its closest homolog, AtNUDX6, are involved in the regulation of biotic stress responses. The expression of AtNUDX7 was shown to be induced by avirulent, virulent, and nonhost pathogenic attacks, as well as treatments with flagellin.Citation21,52–55) KO-nudx7 plants exhibited enhanced resistance to both virulent and avirulent pathogenic strains.Citation21,53,54) Reverse genetic analysis using KO-nudx7 plants and/or several loss-of-function mutants of pathogen resistance-related genes revealed that AtNUDX7 suppressed the ENHANCED DISEASE SUSCEPTIBILITY1 (EDS1) signaling required for basal resistance to invasive pathogens (Fig. ).Citation53,56) Furthermore, Ge et al.Citation26) reported that AtNUDX7 functioned to maintain cellular redox homeostasis and prevent excessive stimulation of defense responses, which is dependent on and independent of NONEXPRESSOR OF PATHOGENESISRELATED GENES1 (NPR1), a master regulator of SA-induced defense genes (SAIGs), and SA accumulation. These findings indicated that AtNUDX7 was a negative regulator of biotic stress responses, possibly by suppressing the excess accumulation of SA (Fig. ). However, the direct actions of these enzymes on such defense systems have not yet been established. Since chemical inhibition of the PAR reaction as well as KO of the AtNUDX7 gene impacted on responses to pathogenic attacks, it had been proposed that the PAR reaction and degradation of ADP-ribose removed from poly(ADP-ribose) may significantly contribute to appropriate plant defense responses (Fig. ).Citation54) Importantly, expression of the AtNUDX7 gene having the mutation of glutamate 154 to glutamine, which abolished hydrolase activity, under the control of the AtNUDX7 promoter could not complement the KO-nudx7 phenotype, demonstrating that the hydrolase activity of AtNUDX7 was required for its normal physiological function.Citation26)
The expression of AtNUDX6 was induced by avirulent pathogenic attacks and treatments with SA and H2O2.Citation50,51,53,55,57) Using KO-nudx6 and AtNUDX6-overexpressing plants, AtNUDX6 was shown to act on the hydrolysis of NADH as a physiological substrate, but barely contributed to that of ADP-ribose.Citation51,57) The expression of thioredoxin h5 (TRX-h5), which acts on the SA-induced activation of NPR1, as well as induction of SA-induced gene expression (PR1, WRKY70, NIMIN1, and NIMIN2), which depend on NPR1, was significantly suppressed and enhanced in SA-treated KO-nudx6 and AtNUDX6-overexpressing plants, respectively.Citation57) These findings indicated that AtNUDX6 was involved in the plant immune response as a positive regulator of NPR1-dependent SA signaling pathways by modulating NADH levels (Fig. ). Some pathogens may have developed a strategy to suppress plant immunity by secreting a NUDX enzyme exhibiting ADP-ribose/NADH pyrophosphohydrolase activity.Citation58)
A more recent study reported that Arabidopsis sensed the ammonium/nitrate ratio as an input signal to determine the amplitude of the EDS1-mediated immune defense response, possibly through the modulation of NO production, in which both AtNUDX6 and AtNUDX7 act as negative regulators.Citation55) Although SA levels were higher in KO-nudx7 plants than in wild-type and KO-nudx6 plants, double KO-nudx6/nudx7 plants accumulated markedly greater amounts of SA than KO-nudx7 plants and exhibited the dwarf phenotype caused by autoimmunity depending on EDS1 and SNC1, the Toll/Interleukin-1 receptor-nucleotide-binding leucine-rich repeat (TIR-NB-LRR)-type R gene. These findings suggested that AtNUDX6 may act synergistically on the EDS1-mediated plant immune response and accumulation of SA regulated by AtNUDX7.
Although it remains controversial whether AtNUDX6 and AtNUDX7 play different roles in defense systems against biotic and abiotic stresses, it is clear that the modulation of intracellular NADH levels is an important tuning point for accurate cellular responses. A comparison of the activities of NADH pyrophosphohydrolase among wild-type, KO-nudx6, and KO-nudx7 plants revealed that AtNUDX7 contributed more than AtNUDX6 to the total pyrophosphohydrolase activity toward NADH under normal conditions and oxidative stress, while AtNUDX6 accounted for the majority of total NADH pyrophosphohydrolase activity in plants treated with SA.Citation57) Plants are simultaneously exposed to abiotic and biotic hazards in nature, which suggests two possibilities (1) the metabolism of NADH can simultaneously regulate cellular responses to both biotic and abiotic stresses, and (2) changes in NADH levels at specific phases and/or in specific microcompartments in plant cells have different effects on cellular responses.
We recently identified the genes (NADH-responsive genes, NRG) whose expression levels were positively or negatively correlated with intracellular NADH levels using single and double KO plants of the AtNUDX6 and AtNUDX7 genes, in which NADH levels were higher in a stepwise manner than those in wild-type plants (Yoshimura et al., unpublished data). No or little overlap was observed between NRGs and the genes responsive to the well-known molecules affecting the cellular redox status, such as ascorbate, glutathione, O3, and H2O2,Citation59–62) suggesting the novel roles of intracellular NADH levels in redox signaling pathways.
On the other hand, a recent study demonstrated that AtNUDX6 and AtNUDX7 were not direct in vivo regulators of NADH.Citation55) The accumulation of NADH during pathogen infections was shown to be suppressed in both triple KO-nudx6/nudx7/eds1 plants and single KO-eds1 plants, suggesting the involvement of EDS1 in the accumulation of NADH. Thus, it is likely that either the existence of AtNUDX6 and AtNUDX7 themselves, but not as pyrophosphohydrolases, or an interaction between each protein and other factor(s) may be necessary for their regulatory roles including the modulation of intracellular NADH levels. The regulatory protein RACK1A, and AGG1 and AGG2 gamma subunits of the signal transducing heterotrimeric G protein were identified as AtNUDX7-interactors, suggesting the formation of regulatory proteins involved in signal transduction.Citation63)
4. Regulation of germination and growth
The growth and the appearance of KO-nudx6 and KO-nudx7 plants were almost the same as those of control plants throughout the growth period under normal conditions on Murashige and Skoog (MS) medium or soil in an isolated growth chamber.Citation49,57) However, growth retardation was observed in KO-nudx7 plants, but not KO-nudx6 plants grown on soil, and this was attributed to the hyperaccumulation of SA and H2O2 leading cell death.Citation55,56) Jambunathan et al.Citation52) indicated that the growth phenotypes of KO-nudx7 plants were due to differences in nutrient compositions; no phenotypic difference was observed between KO-nudx7 and wild-type plants in nutrient-rich medium. Zeng et al.Citation64) reported that KO-nudx7 plants accumulated high levels of ABA, resulting in a reduced seed germination phenotype. Therefore, the modulation of NADH levels by AtNUDX7 may be indispensable for normal seed germination, growth, and development.
5. Regulation of starch synthesis
Similar to its bacterial counterpart, AtNUDX14 (AtASPP) was found to catalyze the hydrolysis of ADP-ribose and ADP-glucose in vitro, with a preference for the former.Citation11,12) However, plants overexpressing AtNUDX14 in chloroplasts had significantly lower levels of both ADP-glucose and starch, indicating that AtNUDX14 hydrolyzed ADP-glucose linked to starch biosynthesis in chloroplasts.Citation11) Potato plants overexpressing the E. coli counterpart, EcASPP, in the cytosol also had markedly lower levels of both ADP-glucose and starch in the leaves and tubers.Citation11) In contrast, potato plants overexpressing potato ASPP, StASPP, in their chloroplasts had lower starch and normal ADP-glucose levels in both tissues. These findings suggested that the ADP-glucose pyrophosphohydrolase may play a role in connecting ADP-glucose metabolism with starch biosynthesis and other cellular processes in response to the physiological needs of the plant.
6. Regulation of flavin synthesis
AtNUDX23 has been shown to exhibit FAD pyrophosphohydrolase activity.Citation12) As predicted, AtNUDX23 was confirmed to be distributed in chloroplasts. The transcript levels of AtNUDX23 as well as genes involved in flavin metabolism (AtFADS, AtRibF1, AtRibF2, AtFMN/FHy, LS, and AtRibA) significantly increased under continuous light, leading to the accumulation of riboflavin (RF) and FAD.Citation65) Not only the overexpression, but also suppression of the AtNUDX23 gene caused decreases in the transcript levels of the flavin metabolic genes and intracellular levels of RF, FMN, and FAD. These findings suggested the negative feedback regulation of the metabolism of flavins, in which AtNUDX23 contributed to the fine-tuning of intracellular flavin levels by regulating the balance between FAD and FMN in plastids through the hydrolysis of FAD.
7. Metabolism of CoA and its derivatives
AtNUDX11, 15, and 15a exhibited pyrophosphohydrolase activities toward CoA and its derivatives.Citation10,12,17) AtNUDX11 and AtNUDX15 were shown to be distributed in the cytosol and mitochondria, respectively (Table ).Citation12,17,66) As described above, the alternatively spliced variant, AtNUDX15a, had both PTS1 and a mitochondrial transit peptide at the C- and N-terminus, respectively. Analyses by the subcellular distribution of AtNUDX15a fused to GFP at its internal regions and immunochemical detection of the protein in fractionated organelles from Arabidopsis cells indicated that the N-terminal mitochondrial transit peptide predominated and, hence, this protein was localized to mitochondria (Table ).Citation17) Given their localization and substrate specificities, the actions of AtNUDX11 and AtNUDX15(a) may impact not only on CoA biosynthesis, but also various metabolic pathways through the hydrolysis of malonyl-CoA, thereby contributing to fatty acid elongation and flavonoid biosynthesis in the cytosol, and succinyl-CoA, a key molecule in the TCA cycle, in mitochondria, respectively.Citation17) Plants overexpressing the AtNUDX11 gene continuously developed shoots until the late growth stage, resulting in the appearance of bigger and wider rosette leaves than those of wild-type plants.Citation66)
8. Metabolism of ppGpp
A hyperphosphorylated guanosine nucleotide, ppGpp, termed an alarmone, is a low-molecular weight effector that helps bacteria survive in limited environments.Citation67,68) ppGpp was found to be produced in plant chloroplasts, a semi-autonomous organelle that originates not only from an ancestral host cell, but also from a cyanobacterium-like photosynthetic prokaryote.Citation69–72) The chloroplast localization of AtNUDX26, a ppGpp pyrophosphohydrolase, was confirmed using GFP fusion proteins at its C-terminus (Table ).Citation24) Intracellular ppGpp levels and the expression levels of ppGpp metabolism-associated genes (AtRSH1, 2, and 3, and AtCRSH) were reported to increase in response to various types of stresses.Citation24,70) The expression of AtNUDX26 was induced by drought, but suppressed by salinity and treatments with PQ, suggesting a role for AtNUDX26 in the regulation of intracellular ppGpp levels in chloroplasts.
9. Regulation of mRNA turnover
mRNA decapping is an important process in eukaryotic mRNA turnover. AtDCP2, which can hydrolyze capped mRNAs, interacted with AtDCP1 and VARICOSE (VCS), Arabidopsis homologs of the proteins involved in forming decapping complex.Citation13,14) In addition to the Nudix motif as the active site, bioinformatic and biochemical analyses demonstrated that there were two additional functionally significant regions; (1) the PDZ domain-binding motif involved in protein stability and (2) the RNA-binding domain required for binding to the RNA moiety of the capped mRNA substrate.Citation15) Consistent with their roles in mRNA decay, AtDCP1, AtDCP2, and VCS colocalized in the cytosol (Table ). Null mutants of either AtDCP1, AtDCP2, or VCS accumulated capped mRNAs with a reduced degradation rate and showed a similar lethal phenotype at the seedling cotyledon stage, suggesting that mRNA turnover mediated by AtDCP2 is required for postembryonic development in Arabidopsis.Citation13,14)
10. AtNUDXs without hydrolase activity toward any substrates
The actions of the 11 remaining AtNUDXs, AtNUDX3–5, 8, 12, 16–18, 21, 22, and 24, have not yet been examined. The expression of AtNUDX5, which is homologous to AtNUDX6 and AtNUDX7, was shown to be markedly induced by avirulent pathogen infections.Citation54) A reporter assay using the −1387 bp 5′-flanking region of the AtNUDX5 gene fused to the GUS reporter showed that AtNUDX5 was weakly expressed in the vascular tissues of young plants and strongly in the axial roots.Citation73) The AtNUDX5 promoter was activated during infections by both avirulent and virulent pathogens, suggesting the involvement of this protein in immune responses. The AtNUDX8 gene was included in the genes whose expression is responsive to H2O2 specifically derived from chloroplasts.Citation60)
III. Conclusions and future perspectives
The findings obtained from the functional analyses of AtNUDXs could help to clarify much of the confusion related to these enzymes in other organisms. Recent studies on the molecular and enzymatic properties as well as physiological functions of AtNUDXs unambiguously uncovered their roles not only in housecleaning and the control of metabolic pathways, but also in the regulation of biotic and abiotic stress responses. Nevertheless, the essential functions of many AtNUDXs in cells remain unknown because of their broad substrate range. In addition, the availability of purified compounds to test often limits the identification of substrates for these enzymes. Although an analysis of Arabidopsis lines disrupted in a particular AtNUDX gene may provide novel insights into its physiological role, the functional redundancy among AtNUDXs with similar enzymatic properties often interferes with their specific roles of respective AtNUDXs; other AtNUDXs from the same subfamily may complement the role of the disrupted one. The determination of relevant substrates and true functions of individual AtNUDXs can be facilitated by combining genetic studies with metabolome analyses as demonstrated for T. thermophilus.Citation74)
Acknowledgments
We express our sincere gratitude to Prof. Fumihiko Horio (Nagoya University), Prof. Akiho Yokota (Nara Institute of Science and Technology), and Prof. Yoshihisa Nakano (Osaka Prefecture University) for their encouragement, support, and discussion. We are deeply grateful to Dr Takahisa Ogawa, Dr Kazuya Ishikawa, Dr Daisuke Ito, Dr Takanori Maruta, and former and current co-workers in our laboratory for their help and cooperation. Part of this work was supported by JSPS/MEXT KAKENHI.
Notes
This review was written in response to the author’s receipt of the JSBBA Award for Young Scientists in 2014.
Abbreviations: ApnA, diadenosine polyphosphates; CoA, coenzyme A; EDS1, ENHANCED DISEASE SUSCEPTIBILITY1; 8-oxo-dGTP, 8-oxo-7, 8-dihydro-2′-deoxy GTP; 8-oxo-G, 8-oxo-7, 8-dihydro-guanine; ppGpp, guanosine-3′,5′-tetraphosphate; IPP, isopentenyl diphosphate; NMNH, nicotinamide mononucleotide; NPR1, NONEXPRESSOR OF PATHOGENESISRELATED GENES1; PAR, poly(ADP-ribosyl)ation; PQ, paraquat; RF, riboflavin; ROS, reactive oxygen species; SA, salicylic acid; TRX, thioredoxin.
References
- Bessman MJ, Frick DN, O’Handley SF. The MutT proteins or “Nudix” hydrolases, a family of versatie, widely distributed “housecleaning” enzymes. J. Biol. Chem. 1996;271:25059–25062.10.1074/jbc.271.41.25059
- McLennan AG. The Nudix hydrolase superfamily. Cell Mol. Life Sci. 2006;63:123–143.10.1007/s00018-005-5386-7
- Taddei F, Hayakawa H, Bouton M, Cirinesi A, Matic I, Sekiguchi M, Radman M. Counteraction by MutT protein of transcriptional errors caused by oxidative damage. Science. 1997;278:128–130.10.1126/science.278.5335.128
- Fowler RG, White SJ, Koyama C, Moore SC, Dunn RL, Schaaper RM. Interactions among the Escherichia coli mutT, mutM, and mutY damage prevention pathways. DNA Repair. 2003;2:159–173.10.1016/S1568-7864(02)00193-3
- Nakabeppu Y, Sakumi K, Sakamoto K, Tsuchimoto D, Tsuzuki T, Nakatsu Y. Mutagenesis and carcinogenesis caused by the oxidation of nucleic acids. Biol. Chem. 2006;387:373–379.
- Kraszewska E. The plant Nudix hydrolase family. Acta Biochim. Pol. 2008;55:663–671.
- Gunawardana D, Likic VA, Gayler KR. A comprehensive bioinformatics analysis of the Nudix superfamily in Arabidopsis thaliana. Comp. Funct. Genomics. 2009;2009:820381.
- Xu W, Shen J, Dunn CA, Desai S, Bessman MJ. The Nudix hydrolases of Deinococcus radiodurans. Mol. Microbiol. 2001;39:286–290.10.1046/j.1365-2958.2001.02267.x
- Xu W, Dunn CA, Jones CR, D'Souza G, Bessman MJ. The 26 Nudix hydrolases of Bacillus cereus, a close relative of Bacillus anthracis. J. Biol. Chem. 2004;279:24861–24865.10.1074/jbc.M403272200
- Ogawa T, Ueda Y, Yoshimura K, Shigeoka S. Comprehensive analysis of cytosolic Nudix hydrolases in Arabidopsis thaliana. J. Biol. Chem. 2005;280:25277–25283.10.1074/jbc.M503536200
- Muñoz FJ, Baroja-Fernández E, Morán-Zorzano MT, Alonso-Casajús N, Pozueta-Romero J. Cloning, expression and characterization of Nudix hydrolase that catalyzes the hydrolytic breakdown of ADP-glucose linked to starch biosynthesis in Arabidopsis thaliana. Plant Cell Physiol. 2006;47:926–934.
- Ogawa T, Yoshimura K, Miyake H, Ishikawa K, Ito D, Tanabe N, Shigeoka S. Molecular characterization of organelle-type Nudix hydrolases in Arabidopsis. Plant Physiol. 2008;148:1412–1424.10.1104/pp.108.128413
- Xu J, Yang JY, Niu QW, Chua NH. Arabidopsis DCP2, DCP1, and varicose form a decapping complex required for postembryonic development. Plant Cell. 2006;18:3386–3398.10.1105/tpc.106.047605
- Iwasaki S, Takeda A, Motose H, Watanabe Y. Characterization of Arabidopsis decapping proteins AtDCP1 and AtDCP2, which are essential for post-embryonic development. FEBS Lett. 2007;581:2455–2459.10.1016/j.febslet.2007.04.051
- Gunawardana D, Cheng HC, Gayler KR. Identification of functional domains in Arabidopsis thaliana mRNA decapping enzyme (AtDcp2). Nucleic Acids Res. 2008;36:203–216.
- Yoshimura K, Ogawa T, Ueda Y, Shigeoka S. AtNUDX1, an 8-oxo-7,8-dihydro-20-deoxyguanosine 5′-triphosphate pyrophosphohydrolase, is responsible for eliminating oxidized nucleotides in Arabidopsis. Plant Cell Physiol. 2007;48:1438–1449.10.1093/pcp/pcm112
- Ito D, Yoshimura K, Ishikawa K, Ogawa T, Maruta T, Shigeoka S. A comparative analysis of the molecular characteristics of the Arabidopsis CoA pyrophosphohydrolases AtNUDX11, 15, and 15a. Biosci. Biotechnol. Biochem. 2012;76:139–147.10.1271/bbb.110636
- Dobrzanska M, Szurmak B, Wyslouch-Cieszynska A, Kraszewska E. Cloning and characterization of the first member of the Nudix family from Arabidopsis thaliana. J. Biol. Chem. 2002;277:50482–50486.10.1074/jbc.M205207200
- Klaus SMJ, Wegkamp A, Sybesma W, Hugenholtz J, Gregory JF, Hanson AD. A Nudix enzyme removes pyrophosphate from dihydroneopterin triphosphate in the folate synthesis pathway of bacteria and plants. J. Biol. Chem. 2005;280:5274–5280.10.1074/jbc.M413759200
- Olejnik K, Kraszewska E. Cloning and characterization of an Arabidopsis thaliana Nudix hydrolase homologous to the mammalian GFG protein. Biochim. Biophys. Acta. 2005;1752:133–141.10.1016/j.bbapap.2005.07.021
- Jambunathan N, Mahalingam R. Analysis of Arabidopsis growth factor gene 1 (AtGFG1) encoding a Nudix hydrolase during oxidative signaling. Planta. 2006;224:1–11.10.1007/s00425-005-0183-y
- Olejnik K, Płochocka D, Grynberg M, Goch G, Gruszecki WI, Basińska T, Kraszewska E. Mutational analysis of the AtNUDT7 Nudix hydrolase from Arabidopsis thaliana reveals residues required for protein quaternary structure formation and activity. Acta Biochim. Pol. 2009;56:291–300.
- Olejnik K, Murcha MW, Whelan J, Kraszewska E. Cloning and characterization of AtNUDT13, a novel mitochondrial Arabidopsis thaliana Nudix hydrolase specific for long-chain diadenosine polyphosphates. FEBS J. 2007;274:4877–4885.10.1111/j.1742-4658.2007.06009.x
- Ito D, Kato T, Maruta T, Tamoi M, Yoshimura K, Shigeoka S. Enzymatic and molecular characterization of Arabidopsis ppgpp pyrophosphohydrolase, AtNUDX26. Biosci. Biotechnol. Biochem. 2012;76:2236–2241.10.1271/bbb.120523
- Goyer A, Hasnain G, Frelin O, Ralat MA, Gregory JF, Hanson AD. A cross-kingdom Nudix enzyme that pre-empts damage in thiamin metabolism. Biochem. J. 2013;454:533–542.10.1042/BJ20130516
- Ge X, Li GJ, Wang SB, Zhu H, Zhu T, Wang X, Xia Y. AtNUDT7, a negative regulator of basal immunity in Arabidopsis, modulates two distinct defense response pathways and is involved in maintaining redox homeostasis. Plant Physiol. 2007;145:204–215.10.1104/pp.107.103374
- Hayakawa H, Hofer L, Thelander L, Kitajima Y, Cai Y, Oshiro S, Yakushiji H, Nakabeppu Y, Kuwano M, Sekiguchi M. Metabolic fate of oxidized guanine ribonucleotides in mammalian cells. Biochemistry. 1999;38:3610–3614.10.1021/bi982361l
- Haghdoost S, Sjölander L, Czene S, Harms-Ringdahl M. The nucleotide pool is a significant target for oxidative stress. Free Radical Biol. Med. 2006;41:620–626.10.1016/j.freeradbiomed.2006.05.003
- Ames BN, Gold LS. Endogenous mutagens and the causes of aging and cancer. Mutat. Res. 1991;250:3–16.10.1016/0027-5107(91)90157-J
- Sekiguchi M, Tsuzuki T. Oxidative nucleotide damage: consequences and prevention. Oncogene. 2002;21:8895–8904.10.1038/sj.onc.1206023
- Mo JY, Maki H, Sekiguchi M. Hydrolytic elimination of a mutagenic nucleotide, 8-oxodGTP, by human 18-kilodalton protein: sanitization of nucleotide pool. Proc. Natl Acad. Sci. USA. 1992;89:11021–11025.10.1073/pnas.89.22.11021
- Sakumi K, Furuichi M, Tsuzuki T, Kakuma T, Kawabata S, Maki H, Sekiguchi M. Cloning and expression of cDNA for a human enzyme that hydrolyzes 8-oxo-dGTP, a mutagenic substrate for DNA synthesis. J. Biol. Chem. 1993;268:23524–23530.
- Cai JP, Ishibashi T, Takagi Y, Hayakawa H, Sekiguchi M. Mouse MTH2 protein which prevents mutations caused by 8-oxoguanine nucleotides. Biochem. Biophys. Res. Commun. 2003;305:1073–1077.10.1016/S0006-291X(03)00864-7
- Ishibashi T, Hayakawa H, Sekiguchi M. A novel mechanism for preventing mutations caused by oxidation of guanine nucleotides. EMBO Rep. 2003;4:479–483.10.1038/sj.embor.embor838
- Ishibashi T, Hayakawa H, Ito R, Miyazawa M, Yamagata Y, Sekiguchi M. Mammalian enzymes for preventing transcriptional errors caused by oxidative damage. Nucleic Acids Res. 2005;33:3779–3784.10.1093/nar/gki682
- Takagi Y, Setoyama D, Ito R, Kamiya H, Yamagata Y, Sekiguchi M. Human MTH3 (NUDT18) protein hydrolyzes oxidized forms of guanosine and deoxyguanosine diphosphates: comparison with MTH1 AND MTH2. J. Biol. Chem. 2012;287:21541–21549.10.1074/jbc.M112.363010
- Yoshimura K, Ogawa T, Tsujimura M, Ishikawa K, Shigeoka S. Ectopic expression of the human MutT-Type Nudix hydrolase, hMTH1, confers enhanced tolerance to oxidative stress in Arabidopsis. Plant Cell Physiol. 2014;55:1534–1543.
- Huber A, Bai P, de Murcia JM, de Murcia G. PARP-1, PARP-2 and ATM in the DNA damage response: functional synergy in mouse development. DNA Repair. 2004;3:1103–1108.10.1016/j.dnarep.2004.06.002
- Qin XJ, Hudson LG, Liu W, Timmins GS, Liu KJ. Low concentration of arsenite exacerbates UVR-induced DNA strand breaks by inhibiting PARP-1 activity. Toxicol. Appl. Pharmacol. 2008;232:41–50.10.1016/j.taap.2008.05.019
- Berger F, Ramirez-Hernandez MH, Ziegler M. The new life of a centenarian: signalling functions of NAD(P). Trends Biochem. Sci. 2004;29:111–118.10.1016/j.tibs.2004.01.007
- Hunt L, Lerner F, Ziegler M. NAD–new roles in signalling and gene regulation in plants. New Phytol. 2004;163:31–44.10.1111/nph.2004.163.issue-1
- Ying W. NAD+ /NADH and NADP+ /NADPH in cellular functions and cell death: regulation and biological consequences. Antioxid. Redox Signal. 2008;10:179–206.10.1089/ars.2007.1672
- Hashida SN, Takahashi H, Uchimiya H. The role of NAD biosynthesis in plant development and stress responses. Ann. Bot. 2009;103:819–824.10.1093/aob/mcp019
- Jacobson EL, Cervantes-Laurean D, Jacobson MK. Glycation of proteins by ADP-ribose. Mol. Cell. Biochem. 1994;138:207–212.10.1007/BF00928463
- McDonald LJ, Moss J. Enzymatic and nonenzymatic ADP-ribosylation of cysteine. Mol. Cell. Biochem. 1994;138:221–226.10.1007/BF00928465
- Bektaş M, Akçakaya H, Aroymak A, Nurten R, Bermek E. Effect of oxidative stress on in vivo ADP-ribosylation of eukaryotic elongation factor 2. Int. J. Biochem. Cell Biol. 2005;37:91–99.10.1016/j.biocel.2004.05.016
- Wang H, Liang Q, Cao K, Ge X. Endogenous protein mono-ADP-ribosylation in Arabidopsis thaliana. Planta. 2011;233:1287–1292.10.1007/s00425-011-1415-y
- Ogawa T, Ishikawa K, Harada K, Fukusaki E, Yoshimura K, Shigeoka S. Overexpression of an ADP-ribose pyrophosphatase, AtNUDX2, confers enhanced tolerance to oxidative stress in Arabidopsis plants. Plant J. 2009;57:289–301.10.1111/tpj.2009.57.issue-2
- Ishikawa K, Ogawa T, Hirosue E, Nakayama Y, Harada K, Fukusaki E, Yoshimura K, Shigeoka S. Modulation of the Poly(ADP-ribosyl)ation reaction via the Arabidopsis ADP-ribose/NADH pyrophosphohydrolase, AtNUDX7, is involved in the response to oxidative stress. Plant Physiol. 2009;151:741–754.10.1104/pp.109.140442
- Davletova S, Rizhsky L, Liang H, Shengqiang Z, Oliver DJ, Coutu J, Shulaev V, Schlauch K, Mittler R. Cytosolic ascorbate peroxidase 1 is a central component of the reactive oxygen gene network of Arabidopsis. Plant Cell. 2005;17:268–281.10.1105/tpc.104.026971
- Ishikawa K, Yoshimura K, Ogawa T, Shigeoka S. Distinct regulation of Arabidopsis ADP-ribose/NADH pyrophosphohydrolases, AtNUDX6 and 7, in biotic and abiotic stress responses. Plant. Signal. Behav. 2010;5:839–841.10.4161/psb.5.7.11820
- Jambunathan N, Penaganti A, Tang Y, Mahalingam R. Modulation of redox homeostasis under suboptimal conditions by Arabidopsis Nudix hydrolase 7. BMC Plant Biol. 2010;10:173.10.1186/1471-2229-10-173
- Bartsch M, Gobbato E, Bednarek P, Debey S, Schultze JL, Bautor J, Parker JE. Salicylic acid-independent enhanced disease susceptibility1 signaling in Arabidopsis immunity and cell death is regulated by the monooxygenase FMO1 and the Nudix hydrolase NUDT7. Plant Cell. 2006;18:1038–1051.10.1105/tpc.105.039982
- Adams-Phillips L, Wan J, Tan X, Dunning FM, Meyers BC, Michelmore RW, Bent AF. Discovery of ADP-ribosylation and other plant defense pathway elements through expression profiling of four different Arabidopsis–Pseudomonas R-avr interactions. Mol Plant Microbe. Interact. 2008;21:646–657.10.1094/MPMI-21-5-0646
- Wang H, Lu Y, Liu P, Wen W, Zhang J, Ge X, Xia Y. The ammonium/nitrate ratio is an input signal in the temperature-modulated, SNC1-mediated and EDS1-dependent autoimmunity of nudt6-2 nudt7. Plant J. 2013;73:262–275.10.1111/tpj.2013.73.issue-2
- Straus MR, Rietz S, Ver Loren van TE, Bartsch M, Parker JE. Salicylic acid antagonism of EDS1-driven cell death is important for immune and oxidative stress responses in Arabidopsis. Plant J. 2010;62:628–640.
- Ishikawa K, Yoshimura K, Harada K, Fukusaki E, Ogawa T, Tamoi M, Shigeoka S. AtNUDX6, an ADP-Ribose/NADH pyrophosphohydrolase in Arabidopsis, positively regulates NPR1-Dependent salicylic acid signaling. Plant Physiol. 2010;152:2000–2012.10.1104/pp.110.153569
- Dong S, Yin W, Kong G, Yang X, Qutob D, Chen Q, Kale SD, Sui Y, Zhang Z, Dou D, Zheng X, Gijzen M, Tyler BM, Wang Y. Phytophthora sojae avirulence effector Avr3b is a secreted NADH and ADP-ribose pyrophosphorylase that modulates plant immunity. PLoS Pathog. 2011;7:e1002353.10.1371/journal.ppat.1002353
- Gao Y, Nishikawa H, Badejo AA, Shibata H, Sawa Y, Nakagawa T, Maruta T, Shigeoka S, Smirnoff N, Ishikawa T. Expression of aspartyl protease and C3HC4-type RING zinc finger genes are responsive to ascorbic acid in Arabidopsis thaliana. J. Exp. Bot. 2011;62:3647–3657.10.1093/jxb/err068
- Maruta T, Noshi M, Tanouchi A, Tamoi M, Yabuta Y, Yoshimura K, Ishikawa T, Shigeoka S. H2O2-triggered retrograde signaling from chloroplasts to nucleus plays specific role in response to stress. J. Biol. Chem. 2012;287:11717–11729.10.1074/jbc.M111.292847
- Queval G, Foyer CH. Redox regulation of photosynthetic gene expression. Philos. Trans. R. Soc. Lond. B. Biol. Sci. 2012;367:3475–3485.10.1098/rstb.2012.0068
- Short EF, North KA, Roberts MR, Hetherington AM, Shirras AD, McAinsh MR. A stress-specific calcium signature regulating an ozone-responsive gene expression network in Arabidopsis. Plant J. 2012;71:948–961.10.1111/tpj.2012.71.issue-6
- Olejnik K, Bucholc M, Anielska-Mazur A, Lipko A, Kujawa M, Modzelan M, Augustyn A, Kraszewska E. Arabidopsis thaliana Nudix hydrolase AtNUDT7 forms complexes with the regulatory RACK1A protein and Ggamma subunits of the signal transducing heterotrimeric G protein. Acta Biochim. Pol. 2011;58:609–616.
- Zeng X, Li YF, Mahalingam R. Arabidopsis Nudix hydrolase 7 plays a role in seed germination. Planta. 2014;239:1015–1025.10.1007/s00425-014-2035-0
- Maruta T, Yoshimoto T, Ito D, Ogawa T, Tamoi M, Yoshimura K, Shigeoka S. An Arabidopsis FAD pyrophosphohydrolase, AtNUDX23, is involved in flavin homeostasis. Plant Cell Physiol. 2012;53:1106–1116.10.1093/pcp/pcs054
- Kupke T, Caparrós-Martín JA, Malquichagua Salazar KJ, Culiáñez-Macià FA. Biochemical and physiological characterization of Arabidopsis thaliana AtCoAse: a Nudix CoA hydrolyzing protein that improves plant development. Physiol. Plant. 2009;135:365–378.10.1111/ppl.2009.135.issue-4
- Stephens JC, Artz SW, Ames BN. Guanosine 5'-diphosphate 3'-diphosphate (ppGpp): positive effector for histidine operon transcription and general signal for amino-acid deficiency. Proc. Natl. Acad. Sci. USA. 1975;72:4389–4393.10.1073/pnas.72.11.4389
- Magnusson LU, Farewell A, Nyström T. ppGpp: a global regulator in Escherichia coli. Trends Microbiol. 2005;13:236–242.10.1016/j.tim.2005.03.008
- Givens RM, Lin MH, Taylor DJ, Mechold U, Berry JO, Hernandez VJ. Inducible expression, enzymatic activity, and origin of higher plant homologues of bacterial RelA/SpoT Stress proteins in nicotiana tabacum. J. Biol. Chem. 2004;279:7495–7504.10.1074/jbc.M311573200
- Takahashi K, Kasai K, Ochi K. Identification of the bacterial alarmone guanosine 5′-diphosphate 3′-diphosphate (ppGpp) in plants. Proc. Natl. Acad. Sci. USA. 2004;101:4320–4324.10.1073/pnas.0308555101
- Kasai K, Usami S, Yamada T, Endo Y, Ochi K, Tozawa Y. A RelA-SpoT homolog (Cr-RSH) identified in Chlamydomonas reinhardtii generates stringent factor in vivo and localizes to chloroplasts in vitro. Nucleic Acids Res. 2002;30:4985–4992.10.1093/nar/gkf628
- Mizusawa K, Masuda S, Ohta H. Expression profiling of four RelA/SpoT-like proteins, homologues of bacterial stringent factors, in Arabidopsis thaliana. Planta. 2008;228:553–562.10.1007/s00425-008-0758-5
- Zhang XC, Li MY, Ruan MB, Xia YJ, Wu KX, Peng M. Isolation of AtNUDT5 gene promoter and characterization of its activity in transgenic Arabidopsis thaliana. Appl. Biochem. Biotechnol. 2013;169:1557–1565.10.1007/s12010-012-0071-4
- Ooga T, Ohashi Y, Kuramitsu S, Koyama Y, Tomita M, Soga T, Masui R. Degradation of ppGpp by Nudix pyrophosphatase modulates the transition of growth phase in the bacterium Thermus thermophilus. J. Biol. Chem. 2009;284:15549–15556.10.1074/jbc.M900582200
- Yang XN, Safrany ST, Shears SB. Site-directed mutagenesis of diphosphoinositol polyphosphate phosphohydrolase, a dual specificity NUDT enzyme that attacks diadenosine polyphosphate and diphosphoinositol polyphosphates. J. Biol. Chem. 1999;274:35434–35440.10.1074/jbc.274.50.35434
- Gasmi L, McLennan AG. The mouse Nudt7 gene encodes a peroxisomal Nudix hydrolase specific for coenzyme A and its derivatives. Biochem. J. 2001;357:33–38.10.1042/0264-6021:3570033
- Dunn CA, O'Handley SF, Frick DN, Bessman MJ. Studies on the ADP-ribose pyrophosphatase subfamily of the Nudix hydrolases and tentative identification of trgB, a gene associated with tellurite resistance. J. Biol. Chem. 1999;274:32318–32324.10.1074/jbc.274.45.32318
- Okada K, Kasahara H, Yamaguchi S, Kawaide H, Kamiya Y, Nojiri H, Yamane H. Genetic evidence for the role of isopentenyl diphosphate isomerases in the mevalonate pathway and plant development in Arabidopsis. Plant Cell Physiol. 2008;49:604–616.10.1093/pcp/pcn032
- Campbell M, Hahn FM, Poulter CD, Leustek T. Analysis of the isopentenyl diphosphate isomerase gene family from Arabidopsis thaliana. Plant Mol. Biol. 1997;32:323–328.
- Xu W, Dunn CA, O'Handley SF, Smith DL, Bessman MJ. Three new Nudix hydrolases from Escherichia coli. J Biol Chem. 2006;281:22794–22798.10.1074/jbc.M603407200
- Szurmak B, Wysłouch-Cieszyńska A, Wszelaka-Rylik M, Bal W, Dobrzańska M. A adenosine 5′,5′′-P1P4 tetraphosphate (Ap4A) hydrolase from Arabidopsis thaliana that is activated preferentially by Mn2+ ions. Acta Biochim. Pol. 2008;55:151–160.
- Rossi L, Denegri M, Torti M, Poirier GG, Scovassi AI. Poly(ADP-ribose) degradation by post-nuclear extracts from human cells. Biochimie. 2002;84:1227–1233.10.1016/S0300-9084(02)00017-2