Abstract
Various low-molecular-weight phytochemicals in green tea (Camellia sinensis L.), especially (–)-epigallocatechin-3-O-gallate (EGCG), are known to be involved in health promotion and disease risk reduction. However, the underlying mechanism has remained elusive because of the absence of an analytical technique that can easily detect the precise behavior of such a small molecule. Recently, we have identified a cell-surface EGCG-sensing receptor and the related signaling molecules that control the physiological functions of EGCG. We also developed a novel in situ label-free imaging technique for visualizing spatially resolved biotransformations based on simultaneous mapping of EGCG and its phase II metabolites. Furthermore, we established a chemometric method capable of evaluating the functionality of multicomponent green tea extracts by focusing on their compositional balances. This review highlights our proposed small molecule-sensing techniques for detecting the complex behavior of green tea components and linking such information to an enhanced understanding of green tea functionality.
Chemical biology and metabolomics-driven small molecule-sensing strategy and techniques for multifaceted evaluations and understanding of green tea functionality
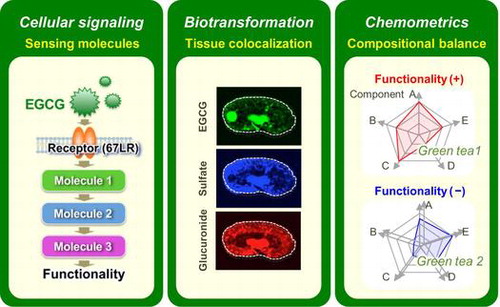
A large number of functionality-oriented nutraceuticals and foods have been developed for widespread consumption, although some have later been included in health hazard evaluation reports on the inadequate and excessive consumption of a specific functional food ingredient.Citation1,2) At present, reliable quantitative evaluation methods for predicting the efficacy, safety, and dosing of dietary components are limited. Detailed knowledge about the exact composition of a food product, the active or inactive ingredient(s), their metabolism and mechanism of action is frequently lacking.Citation3) This issue is due in part to limitations of analytical techniques for detecting complex behaviors of dietary factors in the body and food. To overcome this, at least in part, we must improve the detection sensitivity, accuracy, specificity, coverage, and spatiotemporal resolution of the methods. It is expected that (I) elucidation of specific cellular signaling pathways mediated through a food factor-sensing molecule and (II) the spatiotemporal dynamics of a dietary factor and its metabolites in the tissue micro-regions will lead to an enhanced understanding of its underlying mechanism of action and its precise pharmacokinetics (or nutrikinetics) (Fig. ).Citation4–6) Pharmacology of multicomponent nutraceuticals requires a “network” approach, in which multiple compounds interact with multiple targets in the body with interdependent activities to achieve an optimal effect. The traditional approach to understanding the pharmacology of a multicomponent nutraceutical is to study the effects of a single component on a single biological activity, and gradually assemble those effects into an integrated picture. However, assembling the results obtained from such a reductionistic approach to achieve a system understanding of a concerted pharmacological intervention has proved impractical.Citation7) Non-assemble methodology capable of simultaneously evaluating the relative contribution of multiple compounds present in a nutraceutical to its pharmacological activity is needed. (III) New technology capable of directly attributing not only a single-component behavior in a food product but coexisting multicomponent behaviors to a physiological function is indispensable for clarifying the exact food functionality under multicomponent systems (Fig. ).Citation8)
Fig. 1. Small molecule-sensing strategy and techniques for multifaceted evaluations of green tea functionality.
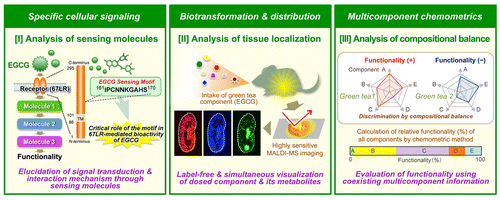
Green tea (Camellia sinensis L.) and its low-molecular-weight components, phytochemicals, have emerged over the past two decades as an important agricultural product and dietary factor for health maintenance and promotion, disease risk reduction, and chemotherapy.Citation9) Beneficial health effects from edible small molecules, phytochemicals, are now considered to be an inexpensive, readily applicable, acceptable, and accessible approach to disease control and management.Citation10) Tea is one of the most widely consumed beverages in the world after water. Green tea, black tea, and oolong tea are all derived from the dried leaves of the plant C. sinensis. Among all teas consumed in the world, green tea is often studied for its health benefits. It has been demonstrated that tea constituents exhibit various biological and pharmacological properties such as anti-carcinogenic, anti-oxidative, anti-allergic, anti-virus, anti-hypertensive, anti-atherosclerosis, anti-cardiovascular disease, and anti-hypercholesterolemic activities.Citation11–18) The principle components responsible for these activities were shown to be catechins, a group of polyphenols. The most abundant and bioactive green tea catechin is (–)-epigallocatechin-3-O-gallate (EGCG).
In the field of food science, chemical biology and metabolomics methods are gradually becoming recognized as powerful approaches for the precise and high-resolution analysis of small molecule behavior. This review describes three new small molecule behavior-sensing techniques suitable for analysis in the cell, tissue, and food (Fig. ) to obtain a better understanding of the functionality of green tea extract (GTE) and its major small molecule components such as EGCG.
I. Specific cellular mechanism(s) responsible for the actions of the green tea polyphenol EGCG
I.I. The discovery of a cell-surface EGCG-sensing receptor
Most of the effects of EGCG observed in cell culture systems and cell-free systems have been studied using considerably higher concentrations than observed in the plasma or tissues of animals or in human plasma after administration of GTE or EGCG.Citation19) Pharmacokinetic studies in humans indicate that the peak plasma concentration after a single dose of EGCG is <1.0 μM.Citation20) Furthermore, the intracellular level of EGCG is much lower than the observed extracellular concentration. Identification of high-affinity proteins that bind to EGCG is the first step to understanding the molecular and biochemical mechanisms of the physiological effects of tea polyphenols. Several proteins that can directly bind to EGCG have been identified from in vitro models. Using an EGCG-conjugated affinity column and two-dimensional electrophoresis, vimentin,Citation21) insulin-like growth factor 1 receptor,Citation22) Fyn,Citation23) glucose-regulated protein 78 kDa,Citation24), and ZAP70Citation25) have been identified as high-affinity EGCG-binding proteins. All of these proteins were demonstrated to be important for the inhibitory activity of EGCG in cell lines, but higher EGCG concentrations than Kd values were needed. For example, vimentin binds to EGCG with a Kd of 3.3 nM, while functional studies showed that EGCG inhibited the phosphorylation of vimentin at Ser55 and Ser50 by Cdc2 with an IC50 of 17 μM. The difference in effective concentrations is probably due to the non-specific binding of EGCG to other proteins, which compete with the target proteins. Therefore, it is not clear whether the activities observed at high EGCG concentrations in vitro will be observed in vivo and if the proposed EGCG-binding molecules are actually responsible for the in vivo physiological activities of EGCG.
To elucidate in detail the molecular basis responsible for the action of EGCG, it is necessary to identify the molecular target that triggers the specific signaling of EGCG. Studies of toll-like receptors (TLRs) teach us the principal role in the pathogen sensing and the need for identification of the specific receptor responsible as a signal initiator for generating cellular responses to understand the specific cellular signaling of foreign or functional substances. Recently, we found by surface plasmon resonance assay that all-trans-retinoic acid (ATRA) enhances the binding of EGCG to the cell surface of cancer cells.Citation26) To identify candidates through which EGCG inhibits cancerous cell growth, we used a subtraction cloning strategy involving cDNA libraries constructed from cells treated or untreated with ATRA. We isolated a single target that allows EGCG to bind to the cell surface. An analysis of the DNA sequence identified this unknown cell-surface candidate as the 67-kDa laminin receptor (67LR). In fact, the expression of this 67LR was enhanced by ATRA treatment.
Human lung cancer A549 cells were then used to assess how effectively the 67LR mediates EGCG-mediated growth inhibition. Cells transfected with empty vector and treated with EGCG showed no growth inhibition. However, cells transfected with the gene encoding 67LR and treated with EGCG demonstrated considerable inhibition as compared with the cells treated with H2O as a vehicle. We next tested whether the growth inhibitory activity of EGCG correlates with the binding affinity of EGCG to the cell surface. We found increased binding of EGCG to the cell surface of cells transfected with 67LR. EGCG binding to the 67LR-transfected cells was inhibited by treatment with an antibody to 67LR. The predicted Kd value for the binding of EGCG to the 67LR protein was 39.9 nM.Citation26) Most of the 67LR protein was found to exist in the fraction of the plasma membrane microdomain, lipid raft, rather than the non-raft fraction,Citation27) and this distribution pattern correlated well with the plasma membrane-associated EGCG level after treating the cells with EGCG.Citation28)
To investigate whether the 67LR can confer sensitivity to EGCG at physiologically relevant concentrations, we treated the 67LR-transfected A549 cells with two concentrations of EGCG (0.1 and 1.0 μM). These concentrations are similar to the amount of EGCG found in human plasma after drinking more than two or three cups of tea. The growth of the transfected cells was inhibited at both of these concentrations.Citation26) This growth suppressive effect was completely eliminated upon treatment with anti-67LR antibody before the addition of EGCG. Additionally, silencing of 67LR by stable RNAi resulted in the attenuation of the inhibitory effect of 1 μM EGCG on cell growth in 67LR-ablated mouse melanoma B16 cells in vitro.Citation29) In a C57BL/6 N mouse xenograft model subcutaneously challenged with 67LR-ablated B16 cells, the anti-tumor effect of an orally administered EGCG was also abrogated.Citation29) Together, these observations demonstrate that the cell-surface 67LR is an important receptor responsible for sensing and managing the anti-tumor action of EGCG at a physiologically relevant concentration. The discovery of the EGCG-sensing receptor 67LR has clarified some of the discrepancies observed between the in vitro and in vivo data on the cancer-preventing activity of EGCG.Citation4)
I.II. The 67-kDa laminin receptor
The 67LR is a non-integrin cell-surface receptor for laminin with high affinity.Citation30) This receptor appears to be very peculiar since only a full-length gene encoding a 37-kDa precursor protein of 295-amino acids has been isolated and the mechanism by which the precursor reaches the mature 67-kDa form is still unclear.Citation31) A hydrophobic segment (residues 86–101) has been hypothesized to act as a transmembrane domain, and the C-terminal domain of the receptor is thought to be involved in the interaction with laminin.Citation32) The evolutionary analysis of the sequence identified as the laminin-binding site in the human protein suggested that the acquisition of the laminin-binding capability is linked to the 173–178 region of the extracellular domain (residues 102–295), which appeared during evolution concomitantly with laminin.Citation33) Its role as a laminin receptor makes it an important molecule in cell adhesion to the basement membrane and in the metastasis of tumor cells.Citation34) Downregulation or inhibition of a 37-kDa/67-kDa laminin receptor impedes the invasion of fibrosarcoma cells, suggesting that the receptor plays a key role in tumor metastasis.Citation35) An increase in 67LR expression compared with the corresponding normal tissue has been found in a variety of common cancers, including breast, cervical, colon, lung, ovary, pancreatic, and prostate carcinomas.Citation36,37) Interestingly, the expression pattern of 67LR correlates well with EGCG susceptibility in both human normal cells and myeloma patient samples.Citation38) Thus, it was postulated that 67LR plays a significant role in tumor progression, and thus, studies conducted to define the function of 67LR could provide a new approach to cancer prevention.
In recent studies on the anti-cancer action of EGCG, a suggested mechanism of interaction between EGCG and its binding proteins was reported.Citation39) The 67LR interacts with many ligands including laminin, cellular pathogenic prion proteins, cytotoxic necrotizing factor 1 from Escherichia coli, Sindbis virus, Venezuelan equine encephalitis virus, Dengue virus, and adeno-associated virus, but the underlying interaction mechanism has not been elucidated.Citation30,31,40–45) The crystal structure of the partial domain of human 67LR was reported and should provide insights into its function and facilitate the design of novel therapeutics targeting 67LR.Citation46) However, a possible role of the interaction of EGCG with 67LR still remains unclear. Recently, we investigated the EGCG–67LR interaction responsible for the bioactivities of EGCG.Citation47) We synthesized various peptides deduced from the extracellular domain corresponding to the 102–295 region of human 67LR encoding a 295-amino acid. The neutralizing activity of these peptides toward EGCG cell-surface binding and inhibition of cancer cell growth was assayed. Both activities were inhibited by a peptide containing a 10-amino acid segment, IPCNNKGAHS, corresponding to residues 161–170. Furthermore, mass spectrometric analysis revealed the non-covalent formation of a EGCG-LR161-170 peptide complex without the formation of a covalent complex of EGCG chemically altered with the peptide. An amino acid deletion/replacement study of the peptide LR161–170 indicated that the 10-amino acid length and two basic amino acids, K166 and H169, have a critical role in neutralizing EGCG’s activities. Moreover, neutralizing activity against the anti-proliferation action of EGCG was observed in the presence of a recombinant protein of the extracellular domain of 67LR, and this effect was abrogated by a deletion of residues 161–170. These findings support that the 10-amino acid sequence, IPCNNKGAHS, might be the functional EGCG-sensing motif responsible for the anti-cancer activity of EGCG. Overall, these results highlight the nature of the EGCG–67LR interaction and provide novel structural insights into understanding 67LR-mediated functions of EGCG (EGCG-sensing cellular mechanism), and could aid in the development of potential anti-cancer compounds for chemopreventive or therapeutic uses that can mimic EGCG–67LR interactions.
I.III. Anti-allergic action of EGCG through the 67LR
Mast cells and basophils play a central role in immediate allergic reactions mediated by immunoglobulin E (IgE). Binding of multivalent allergens to specific IgE attached to the high-affinity IgE receptor (FcεRI) on the surface of mast cells or basophils leads to the release of both preformed and newly generated inflammatory mediators such as histamine. These mediators ultimately cause various symptoms including atopic dermatitis, bronchial asthma, and food allergy.Citation48,49) The early phase of cell activation of mast cells and basophils includes the phosphorylation and activation of protein tyrosine kinases and their substrates, generation of the second messengers such as inositol trisphosphate and diacylglycerol, and elevation of intracellular Ca2+ levels.Citation50,51) The late phase of the activation, which occurs after the influx of Ca2+, includes the fusion of secretory granules with the membrane and dramatic morphological changes due to remodeling of the actin cytoskeleton, which undergo extensive membrane ruffling.Citation52–54) We found that EGCG inhibited the calcium ionophore A23187-induced histamine release from human basophilic KU812 cells and did not inhibit the increase of the intracellular Ca2+ level after stimulation with A23187.Citation55) This result suggested that the effect of EGCG on histamine release occurs after the elevation of the intracellular Ca2+ concentration. Thr18/Ser19 phosphorylation of the myosin regulatory light chain (MRLC) has been reported to be temporally correlated with degranulation in rat basophilic RBL-2H3 cells, and the inhibition of MRLC phosphorylation has been shown to impair the degranulation.Citation56) Although the non-gallated type of catechin, epigallocatechin, with no ability to inhibit histamine release, showed no inhibitory effect toward MRLC phosphorylation, the gallated type of catechin, EGCG, clearly reduced the level of phosphorylated MRLC.Citation55) After treatment of KU812 cells with anti-67LR antibody, cells were incubated with EGCG and further challenged with A23187. The reductive effect of EGCG on the histamine release was almost completely inhibited in cells treated with the anti-67LR antibody. An experiment using such 67LR-downregulated cells revealed a significant abrogation of the inhibitory effect of EGCG on degranulation. Furthermore, the lowering effect of EGCG on the phosphorylation of MRLC was also inhibited by treatment with either the anti-67LR antibody or 67LR-knockdown. These findings indicate that the inhibitory effect of EGCG on degranulation was caused by a modification of the myosin cytoskeleton through the binding of EGCG to 67LR on the cell surface. When the basophilic cells were stimulated with A23187 in the presence of EGCG, membrane ruffling was inhibited and a biased F-actin accumulation was observed. Furthermore, this EGCG-induced actin remodeling was abolished in both anti-67LR antibody-treated cells and 67LR-knockdowned cells.Citation55) Our findings indicated that EGCG-induced actin remodeling is caused by lowering MRLC phosphorylation mediated through the binding of EGCG to the 67LR (Fig. ). Thus, these cytoskeletal modifications may play an important role in the inhibition of histamine release by EGCG.
Fig. 2. Possible EGCG signaling pathways for immunomodulatory actions through the EGCG-sensing receptor 67LR.
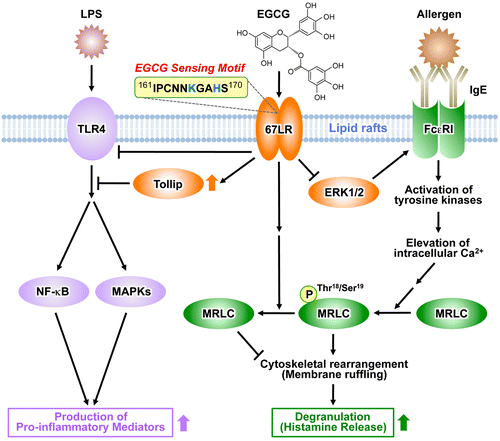
FcεRI plays a central role in the induction and maintenance of IgE-mediated allergic responses such as atopic dermatitis, bronchial asthma, and food allergy. Analysis of α chain-deficient mice demonstrated that IgE was unable to bind to the cell surface of mast cells, thereby abrogating the induction of degranulation through IgE binding.Citation57) Thus, it is expected that the downregulation of FcεRI expression in mast cells and basophils may lead to the attenuation of IgE-mediated allergic symptoms. We found that EGCG was able to decrease the cell-surface expression of FcεRI in human basophilic KU812 cells. Total cellular expression of the FcεRI α chain decreased upon treatment with EGCG. We also found that EGCG has an ability to inhibit the phosphorylation of the extracellular signal-regulated kinase 1/2 (ERK1/2), a family of mitogen-activated protein kinases (MAPKs).Citation28) This inhibition was involved in downregulation of FcεRI expression by EGCG. Moreover, the inhibitory effect elicited by EGCG on ERK1/2 was prevented by disruption of lipid rafts. Accordingly, the interaction between EGCG and the lipid rafts is important for EGCG’s ability to downregulate FcεRI expression, and ERK1/2 may be involved in this suppression signal. We also demonstrated that the suppressive effect of EGCG was inhibited by the knockdown of 67LR. Furthermore, the ability of EGCG to decrease the phosphorylation of ERK1/2 was reduced in the 67LR-knockeddown cells. These results indicate that the effect of EGCG on ERK1/2 phosphorylation correlates with the expression of 67LR, which implies that the 67LR is responsible for transducing the EGCG-induced downregulatory signaling of the FcεRI (Fig. ). The O-methylated derivatives of EGCG, (–)-epigallocatechin-3-O-(3″-O-methyl)-gallate (EGCG3″Me) and (–)-epigallocatechin-3-O-(4″-O-methyl)-gallate (EGCG4″Me), which can be isolated from tea leaves such as tong-ting oolong tea or cultivar “Benifuuki”, have been shown to inhibit allergic reactions in vitro.Citation58,59) The inhibitory effects of O-methylated EGCG on in vivo mouse type I and IV allergies more are potent than EGCG.Citation58,60) These catechins also strongly inhibited mast cell activation through the prevention of tyrosine phosphorylation (Lyn, Syk, and Btk) of cellular protein, histamine/leukotriene release, and interleukin (IL)-2 secretion after FcεRI cross-linking.Citation61) A double-blind clinical trial to treat allergic cedar pollinosis patients with “Benifuuki” green tea rich in EGCG3″Me showed promising results, such as significant relief of blowing the nose and itching eyes, when 1.5 g of tea powder mixed in water was consumed twice a day for 13 weeks.Citation62) We found that EGCG3″Me can inhibit histamine release and suppress FcεRI expression in human basophilic KU812 cells the same as EGCG.Citation59,63) RNAi-mediated knockdown of 67LR expression resulted in decreased activity of EGCG3″Me.Citation64) The suppression of MRLC phosphorylation through cell-surface binding to the 67LR contributed to the inhibitory effect of EGCG3″Me on the histamine release. The 67LR also mediated the EGCG3″Me-induced suppression of FcεRI expression by reducing ERK1/2 phosphorylation. EGCG is known to be unstable and is degraded easily in animal bodies. In contrast, EGCG3″Me and EGCG4″Me are absorbed efficiently and are more stable than EGCG in animal and human plasma, supporting the observation that the methylated derivatives of EGCG have potent inhibitory activities to allergies in vivo. EGCG has been reported to undergo methylation, (–)-4′-O-methyl-epigallocatechin-3-O-(4″-O-methyl) gallate (EGCG4′4″diMe) is one of the major metabolites of EGCG in plasma,Citation65) and EGCG4′4″diMe did not demonstrate a suppressive effect in KU812 cells.Citation66) Although our studies on methylated EGCGs have contributed to the elucidation of the physiological activities of EGCG in vivo, further investigation of the relationship between metabolites of EGCG and 67LR is necessary for a better understanding of the molecular basis of EGCG activities in vivo.
I.IV. Anti-inflammatory action of EGCG through the 67LR
TLRs are important in the activation of the innate immune response and are pathogen recognition proteins that have important roles in detecting microbes and initiating inflammatory responses.Citation67) Recognition of microbial components by TLRs plays a central role in the immune system’s decision to respond or not to a particular microbial infection. Lipopolysaccharide (LPS), a major component of the outer membrane of Gram-negative bacteria, is one of the most powerful activators of TLR4 signaling and is also well known to induce production of inflammatory mediators, such as tumor necrosis factor-α, IL-6, and nitric oxide, and activation of the MAPK signaling pathway and NF-κB, leading to death from endotoxic shock in animal models.Citation68–70) EGCG has been shown to rescue mice from LPS-induced lethal endotoxemia and downregulate inflammatory responses in macrophages.Citation71) Recently, we identified the molecular basis for the downregulation of TLR4 signal transduction by EGCG at 1 μM in macrophages.Citation72) Anti-67LR antibody treatment or RNAi-mediated silencing of 67LR resulted in abrogation of the inhibitory action of EGCG on LPS-induced activation of downstream signaling pathways and target gene expressions in murine macrophages. Additionally, we found that EGCG reduced the TLR4 expression through 67LR. Interestingly, EGCG induced a rapid upregulation of Tollip protein, a negative regulator of TLR signaling, and this EGCG action was prevented by 67LR silencing or anti-67LR antibody treatment. RNAi-mediated silencing of Tollip impaired the TLR4 signaling inhibitory activity of EGCG. Tollip was also involved in the inhibitory action of EGCG on TLR2 response, peptidoglycan-induced production of pro-inflammatory mediators, and activation of MAPKs.Citation73) Unlike the TLR4, EGCG did not inhibit the expression of TLR2 protein. Taken together, these findings demonstrate that 67LR plays a critical role in mediating the anti-inflammatory action of physiologically relevant EGCG and Tollip expression could be modulated through 67LR in macrophages (Fig. ). These results not only illuminate the mechanisms for the inflammation inhibitory activity of EGCG, but also should help in the design of new strategies to prevent inflammatory diseases. Therefore, strategies for upregulation of Tollip and downregulation of TLR4 expression via 67LR may prove effective in treating both chronic and acute inflammatory diseases.
II. Biotransformation and distribution of the green tea polyphenol EGCG
To elucidate the precise mechanism underlying the bioactivity of green tea polyphenols, its high-resolution spatiotemporal information is needed. Although some studies have visualized its tissue distribution by fluorescence imaging, cerium chloride staining, immunostaining, and radioactive labeling assays,Citation74–77) spatiotemporal information has been lacking because of the absence of an analytical technique that can easily detect the localization of the naïve polyphenol. Conventional molecular imaging generally requires labeling steps that are time-consuming, expensive, and labor-intensive. Additionally, the molecular discriminating powers of these techniques are insufficient for visualizing the target compound and its metabolites simultaneously. A label-free molecular imaging technique could overcome these issues, but the development of such a technique has been a challenge.
Mass spectrometry imaging (MSI) is a new technology capable of determining the naïve distribution of ionizable biological molecules in tissue sections on the basis of their specific mass-to-charge ratios without any labeling (Fig. ). This technique can theoretically detect target molecules and their metabolites simultaneously in a single analysis, and is now widely used for in situ imaging of endogenous and exogenous molecules such as proteins, lipids, drugs, and their metabolites.Citation78–80) It is a potential tool useful for pathological analysis and understanding diseases or pharmaceutical mechanisms. Matrix-assisted laser desorption/ionization (MALDI) is a commonly available ionization method used for MSI. MALDI-MSI, using traditional major matrices, such as 2,5-dihydroxy benzoic acid (DHB), sinapinic acid (SA), and α-cyano-4-hydroxycinnamic acid (CHCA), can visualize macromolecules such as lipids and proteins/peptides. However, small molecules are not easily detected by MALDI-MSI because many matrix and/or matrix-analyte cluster ion peaks are observed in the low mass range (m/z < 700). In contrast, we recently reported that MALDI-MS with 9-aminoacridine (9-AA) provided improved sensitivity for the detection of endogenous low-molecular-weight metabolites in negative ionization mode.Citation81–83) This technique was also useful to visualize both drastic and subtle changes in the spatiotemporal distributions of various cerebral metabolites in response to pathological perturbations. However, it is still unclear whether such highly sensitive MALDI-MSI techniques can visualize low-molecular-weight bioactive polyphenols. Major matrices such as DHB, CHCA, SA, and 9-AA are certainly effective for MALDI-MSI of limited drugs in tissue sections, but such matrices cannot be used to easily visualize the localization of many dosed dietary compounds (food factors), including phytochemicals and their metabolites, because of their low abundance in target tissue as well as interference with background peaks from the matrix and endogenous molecules.Citation84,85) For effective ionization of the analyte in MALDI-MS, the optimum matrix needs to be determined because there is often no direct correlation between the choice of matrix and its ability to ionize a bioactive small molecule of interest. We screened 41 chemicals as potential matrices for the representative bioactive green tea polyphenol, and EGCG.Citation5) EGCG peaks were not observed with DHB, CHCA, SA, or 9-AA. However, 1,5-diaminonaphthalene (1,5-DAN), harmane, norharmane, harmine, and ferulic acid all allowed for the detection of EGCG (m/z 457 [M–H]−) in negative ionization mode without any background peak interference.Citation5) Furthermore, among such candidate chemicals, only 1,5-DAN was useful to visualize the distribution of a single oral dose of EGCG (2,000 mg/kg b.w.) in mouse tissue sections. Provided that chemical screening data are available online, this information may be useful for matrix selection and development for highly sensitive detection of EGCG or its derivatives, and structure-based matrix screening for MSI of dietary polyphenolic compounds.
Understanding the metabolic fates of bioactive dietary polyphenols is indispensable for determining their in vivo molecular mechanisms.Citation39) Some studies have reported that green tea polyphenols are subjected to phase II biotransformation and predominantly undergo methylation, glucuronidation, and sulfation in the intestine, liver, and kidneys.Citation86) However, both the functions of the metabolites and their localizations in different tissue micro-regions were unclear.Citation87) In contrast, 1,5-DAN-MALDI-MSI was able to visualize a spatially resolved biotransformation based on simultaneous mapping of orally dosed EGCG and its phase II metabolites including its monosulfate (m/z 537) and monoglucuronide (m/z 633) forms (Fig. ).Citation5) Interestingly, unlike liver, the localization patterns in the kidney compartments (pelvis, medulla, and cortex) were clearly different among EGCG and its phase II metabolites. In the kidney tissue extract, EGCG and its major conjugates (methylated, sulfated, and glucuronidated forms) were observed. The peak abundance of these three conjugates was markedly lower than that of EGCG. Nevertheless, both sulfated and glucuronidated forms were detected in MALDI-MSI measurements, but there was no peak for the methylated form (m/z 471 [M–H]−). In negative ionization mode MALDI-MS, the phosphorylated compounds and carboxylic acids were efficiently ionized, indicating that compounds with leaving groups, including phosphate and carboxylic groups, readily undergo deprotonation.Citation81–83,88) Unlike methylation, sulfation or glucuronidation can introduce a leaving group (sulfate or carboxylic group, respectively) into EGCG. Therefore, in negative ionization mode MALDI-MS using 1,5-DAN, the introduction of such an ionizable group may contribute to preferable ionization, higher MALDI efficiency, of EGCG phase II conjugates in spite of their lowered tissue abundance compared with EGCG.Citation5) Although the bioavailability of EGCG is very low,Citation39,86) this sensing technology was able to visualize, for the first time, the in situ distribution of EGCG phase II metabolites in liver and kidney sections after oral dosing. The use of 1,5-DAN-MALDI-MSI will open new avenues for investigating the in situ metabolism of a bioactive dietary polyphenol subjected to phase II biotransformation, and may help to accelerate the highly effective and efficient design of plant-derived pharmaceuticals, multicomponent botanical drugs, dietary supplements, and functional foods. The advantages of this MALDI-MS methodology include label-free imaging and simultaneous detection of an orally dosed dietary polyphenol and its metabolites. It should help to overcome the drawbacks associated with conventional molecular imaging techniques.
Fig. 4. In situ label-free visualization of an orally dosed EGCG and its phase II metabolites in tissue micro-regions.
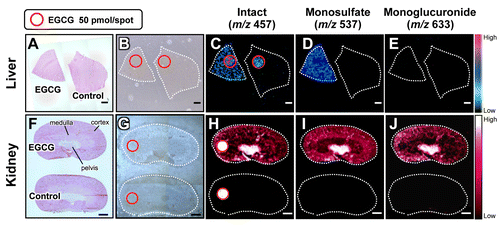
However, this technique could not be used to visualize EGCG and its phase II metabolites in both kidney and liver tissue sections after oral dosing at a normal intake level (20 mg/kg b.w.).Citation5) To overcome the limitation of the 1,5-DAN-MALDI-MSI technique and to ensure its practical use, further improvement of its detection sensitivity, such as employing a targeted selective reaction monitoring mode (SRM) approach using a triple quadrupole system (QqQ),Citation89) application of a Fourier transform ion cyclotron resonance-MS (FT-ICR-MS) instrument capable of accumulating continuously selected ions,Citation90) and on-tissue chemical derivatization approaches to increase the ionization efficiencyCitation91,92) as well as the improvement of MALDI efficiency based on matrix choice and development, may be required. Additionally, other studies including kinetic histochemistry,Citation80,84,93) microscopic analysis with high-spatial resolution,Citation94–96) 3D imaging,Citation97–99), and distribution of other metabolitesCitation86,99) will be required to unravel both the biological consequences of biotransformation of the dietary polyphenol and its mechanism(s) of action.
III. Evaluation of green tea functionality on the basis of its compositional balance
The chemical components of tea vary according to the species/cultivar, environment, growth, storage conditions, and leaf quality.Citation100) In most cases, the quality and bioactive functions (i.e. the health promotion effects in human and animal models) of tea are defined by their specific compositions. The functional biochemistry of plants is very diverse, the concentrations of many compounds vary widely, and metabolomic analyses are required to simultaneously determine a broad range of metabolites in plant extracts. Among many analytical platforms, MS is the most sensitive and selective technique, and thus, it is the method of choice for metabolomic research on plants.Citation101) LC–MS can be adapted to a wide range of molecules, such as secondary metabolites.Citation102) Metabolomic studies coupled with chemometric methods including principal component analysis (PCA) and partial least-squares (PLS) regression analysis have been used to explore the relationships between the metabolome of diverse plant species and their genotype, origin, vintage, quality, or other specific attributes.Citation103–108) Metabolic profiling techniques are often used to evaluate the nutraceutical (nutritional or physiological) value of a single plant cultivar for quality control and breeding. In the field of nutraceutical (functional food) research, such techniques have been used to identify subtle metabolic differences among individuals or different environmental conditions, for example, diet.Citation109,110) This chemometric approach, multivariate statistical analysis using multivariate data (e.g. MS datasets), can clarify similarities or differences among samples by compositional balance on the basis of the relative abundance of each metabolite to the total abundance of all metabolites. Additionally, such a technique enables us to theoretically calculate the relative contribution of all multicomponent factors detected in crude samples to the total functionality. Considering the principle of this methodology, it is expected that metabolic profiling may become an effective strategy for obtaining a comprehensive understanding of the attributes of crude samples, including multicomponent foods (agricultural products). In the functional food research field, various functionalities are evaluated by activity and abundance of a single particular component in foods, but simultaneous evaluation of coexisting multicomponent factors is required for elucidating real functionality in multicomponent systems. However, to date, there has been little research on the use of metabolic profiling to compare or predict the nutraceutical (bioactive) properties (the health promotion effects in human and animal models) of many plant cultivars. Therefore, elucidating the relationship between the metabolome and the bioactivity of diverse cultivars could be a novel strategy for identifying the nutraceutical potential of various plant cultivars for functional food design.
GTEs have various health-promoting activities, and these activities vary from cultivar to cultivar. We performed metabolomic analyses to explore the relationship between the metabolome and health-promoting attributes of diverse Japanese green tea cultivars.Citation8) We investigated the ability of leaf extracts from 43 Japanese green tea cultivars to inhibit thrombin-induced phosphorylation of MRLC in human umbilical vein endothelial cells (HUVECs). This thrombin-induced phosphorylation is a potential hallmark of vascular endothelial dysfunction. Among the tested cultivars, Cha Chuukanbohon Nou-6 and Sunrouge (SR) strongly inhibited MRLC phosphorylation. To evaluate the bioactivity of green tea cultivars using a metabolomics approach, metabolite profiles of all GTEs were determined by LC–MS. multivariate statistical analyses, PCA, and orthogonal PLS-discriminant analysis (OPLS-DA) revealed differences among green tea cultivars with respect to their ability to inhibit MRLC phosphorylation (Fig. ). The PCA score plot showed clear independent clusters, one consisting of cultivars with higher bioactivity (Nou-6 and SR, ranking: 1 and 2, respectively), and the other consisting of the remaining cultivars including the non-bioactive cultivar Yabukita (YB) (ranking: 32) and the less bioactive cultivar Benifuuki (BF) (ranking: 18) (Fig. (A)). YB is the most commonly consumed and widely distributed cultivar in Japan, accounting for 70–80% of all green tea consumed. BF has been reported biomedical activities in human models.Citation62,111) SR was generated by natural crossbreeding of Nou-6. Compared with Nou-6, this novel cultivar contains high levels of anthocyanins, is high yielding and easy to grow, and shows high resistance to anthracnose and gray blight. Both the bioactivity and metabolic profiles of SR were almost identical to that of Nou-6, but not to that of YB and BF (Fig. (A)–(C)). Polyphenols were associated with the unique metabolic profile and bioactivity of the SR cultivar. Interestingly, almost all of the metabolite peaks focused by multiple OPLS-DA (SR vs other cultivars) overlapped with those removed by treatment with polyvinylpolypyrrolidone (PVPP), a remover of polyphenolic compounds. Considering the significance of the PVPP test to indicate bioactive groups (polyphenols) in SR tea extract,Citation8) it may be reasonable to use OPLS-DA for simple and high-precision screening for bioactive factors without the need for additional fractionation, purification, and bioactivity experiments such as PVPP tests. These observations support the applicability of metabolic profiling with multivariate statistical analysis in nutraceutical research. Using PLS regression analysis, we also succeeded in constructing a reliable bioactivity-prediction model to predict the inhibitory effect of tea cultivars based on their metabolome (Fig. (D)). This model was based on certain identified metabolites that were associated with bioactivity. Intriguingly, when added to an extract from the non-bioactive cultivar YB, several metabolites enriched in SR were able to transform it into a bioactive extract.Citation8) Generally, most bioactive phytochemicals are isolated from crude extracts by fractionation and purification, during which low abundance but potentially interesting compounds may be lost. In contrast, our proposed chemometric approach allows for the identification of the promising compounds in a crude mixture containing many compounds at different concentrations in a single analysis without fractionation or purification steps prior to LC–MS as well as additional bioactivity assessment after LC–MS. This strategy may prove valuable for the isolation of additional bioactive compounds from GTEs.
Fig. 5. Multivariate statistical analyses of metabolic profiles of diverse green tea cultivars and their bioactivities.
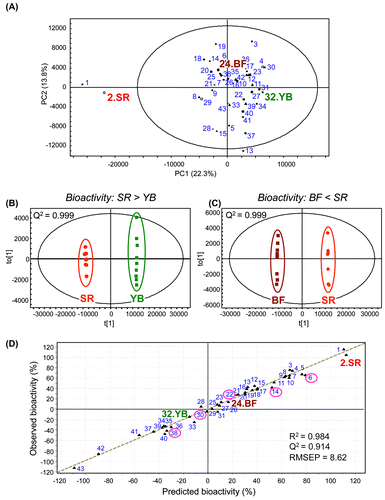
Recently, Ku and coworkers used metabolomic approaches to determine the effect of manufacturing type or cultivation method on the chemical composition of a single tea cultivar (green tea or pu-erh tea).Citation112,113) They suggested that several polyphenolic compounds were associated with a specific manufacturing type or cultivation method. In contrast, we investigated relationships between metabolomic data and health-promoting effects in 43 green tea cultivars.Citation8) In the cultivar SR, certain polyphenolic constituents (delphinidin-3-O-glucoside/galactoside, quercetin-3-O-glucoside/galactoside, cyanidin-3-O-(6-O-(E)-p-coumaroyl)-β-galactoside, and theogallin) were associated with bioactivity. Although polyphenols have many health-promoting effects, the relationship between these compounds and the inhibition of MRLC phosphorylation in human endothelial cells remain unclear. These polyphenols differed from those reported by Ku et al.Citation112,113) Additionally, such polyphenols, especially anthocyanins, were barely detected in the most consumed and distributed Japanese green tea cultivar. These facts support the potential value of our identified polyphenols and indicate that a metabolomic approach, an investigation of compositional balance in diverse crude samples with different bioactivities, is a useful tool for identifying unique bioactive factors. This information may be useful for the development of markers to produce new cultivars with greater bioactivity and to screen for bioactive tea cultivars.
A metabolic profiling approach enables us to obtain compositional information based on a correlation between relative component abundance and bioactivity (functionality), which is indispensable for a better understanding of green tea functionality under multicomponent systems. This chemometric methodology can easily calculate the relative functionality of all coexisting multicomponent factors detected in GTEs (Fig. ). This information may contribute to the creation of new scientific evidence useful for the development of green tea-based functional foods, multicomponent botanical drugs, and combinations of foods/beverages preferable for health promotion.
IV. Concluding remarks and future prospects
For specifically sensing complex behaviors of low-molecular-weight green tea components in the body or leaf infusion, we have proposed new concepts and techniques (Fig. ). (I) A green tea catechin EGCG-sensing cellular system based on the identification of the EGCG-sensing receptor 67LR and its downstream signaling pathways, (II) an in situ label-free MALDI-MS imaging technique for visualizing spatially resolved biotransformation based on simultaneous mapping of orally dosed EGCG and its phase II metabolites, and (III) a chemometrics approach capable of discriminating the functionality of multicomponent GTEs by their compositional balances and calculating the relative functionality of all components. These findings will provide significant insights into functionality-related interactions between green tea components and the body. This approach should overcome the drawbacks (low specificity, time-consuming, expensive, labor-intensive, and non-multicomponent properties) associated with conventional molecular-sensing techniques. More beneficial information on the usefulness of small molecule-sensing technology in green tea research will emerge from further biological applications such as disease model analysis and human intervention trials. We hope that this review will have wide-ranging applications to the studies of other foods and herbal medicines as well as their ingredients.
Acknowledgments
This research was conducted in the Laboratory of Food Chemistry, Faculty of Agriculture, Graduate School, and Innovation Center for Medical Redox Navigation (ICMRN), Kyushu University. I would like to thank the past and present members of our laboratory and all our collaborators for their considerable encouragement and generous cooperation. I also wish to express my deepest appreciation to Professor Koji Yamada and Professor Hirofumi Tachibana for their detailed guidance, continuing encouragement, and helpful suggestions in terms of food functionality and chemical biology research. I am very grateful to the former director of ICMRN, Professor Emeritus Hideo Utsumi, Professor Hiroyuki Wariishi, and Associate Professor Daisuke Miura for valuable guidance and considerable support in terms of metabolomics research. I am also grateful to Dr Mari Maeda-Yamamoto (National Agriculture and Food Research Organization) for valuable support of green tea preparation. These studies were supported in part by JSPS KAKENHI Grants, several research projects from the Ministry of Agriculture, Forestry and Fisheries of Japan, and the MEXT Funding – Project for Developing Innovation Systems – Creation of Innovation Centers for Advanced Interdisciplinary Research Areas Program in Japan. Finally, I would like to thank the Japan Society for Bioscience, Biotechnology, and Agrochemistry (JSBBA) for bestowing on me the JSBBA Award for the Encouragement of Young Scientists.
Notes
This review was written in response to the author’s receipt of the JSBBA Award for Young Scientists in 2014.
References
- Eussen SR, Verhagen H, Klungel OH, Garssen J, van Loveren H, van Kranen HJ, Rompelberg CJ. Functional foods and dietary supplements: products at the interface between pharma and nutrition. Eur. J. Pharmacol. 2011;668:S2–S9.10.1016/j.ejphar.2011.07.008
- Meoni P, Restani P, Mancama DT. Review of existing experimental approaches for the clinical evaluation of the benefits of plant food supplements on cardiovascular function. Food Funct. 2013;4:856–870.10.1039/c3fo30323c
- van Velzen EJ, Westerhuis JA, van Duynhoven JP, van Dorsten FA, Grün CH, Jacobs DM, Duchateau GS, Vis DJ, Smilde AK. Phenotyping tea consumers by nutrikinetic analysis of polyphenolic end-metabolites. J. Proteome Res. 2009;8:3317–3330.10.1021/pr801071p
- Tachibana H. Green tea polyphenol sensing. Proc. Jpn. Acad. Ser. B Phys. Biol. Sci. 2011;87:66–80.10.2183/pjab.87.66
- Kim YH, Fujimura Y, Hagihara T, Sasaki M, Yukihira D, Nagao T, Miura D, Yamaguchi S, Saito K, Tanaka H, Wariishi H, Yamada K, Tachibana H. In situ label-free imaging for visualizing the biotransformation of a bioactive polyphenol. Sci. Rep. 2013;3:2805.
- Castellino S. MALDI imaging MS analysis of drug distribution in tissue: the right time!(?). Bioanalysis. 2012;4:2549–2551.10.4155/bio.12.251
- Xue T, Roy R. Studying traditional Chinese medicine. Science. 2003;300:740–741.10.1126/science.300.5620.740
- Fujimura Y, Kurihara K, Ida M, Kosaka R, Miura D, Wariishi H, Maeda-Yamamoto M, Nesumi A, Saito T, Kanda T, Yamada K, Tachibana H. Metabolomics-driven nutraceutical evaluation of diverse green tea cultivars. PLoS One. 2011;6:e23426.10.1371/journal.pone.0023426
- Khan N, Mukhtar H. Tea and health: studies in humans. Curr. Pharm. Des. 2013;19:6141–6147.10.2174/1381612811319340008
- Surh YJ. Cancer chemoprevention with dietary phytochemicals. Nat. Rev. Cancer. 2003;3:768–780.10.1038/nrc1189
- Lambert JD, Yang CS. Cancer chemopreventive activity and bioavailability of tea and tea polyphenols. Mutat. Res. 2003;523–524:201–208.10.1016/S0027-5107(02)00336-6
- Chisaka T, Matsuda H, Kubomura Y, Mochizuki M, Yamahara J, Fujimura H. The effect of crude drugs on experimental hypercholesteremia: mode of action of (−)-epigallocatechin gallate in tea leaves. Chem. Pharm. Bull. 1988;36:227–233.10.1248/cpb.36.227
- Cao Y, Cao R. Angiogenesis inhibited by drinking tea. Nature. 1999;398:381.10.1038/18793
- Kuriyama S, Shimazu T, Ohmori K, Kikuchi N, Nakaya N, Nishino Y, Tsubono Y, Tsuji I. Green tea consumption and mortality due to cardiovascular disease, cancer, and all causes in Japan. JAMA. 2006;296:1255–1265.10.1001/jama.296.10.1255
- Sazuka M, Murakami S, Isemura M, Satoh K, Nukiwa T. Inhibitory effects of green tea infusion on in vitro invasion and in vivo metastasis of mouse lung carcinoma cells. Cancer Lett. 1995;98:27–31.10.1016/S0304-3835(06)80006-6
- Bors W, Saran M. Radical scavenging by flavonoid antioxidants. Free Radical Res. 1987;2:289–294.10.3109/10715768709065294
- Sano M, Takahashi Y, Yoshino K, Shimoi K, Nakamura Y, Tomita I, Oguni I, Konomoto H. Effect of tea (Camellia sinensis L.) on lipid peroxidation in rat liver and kidney: a comparison of green and black tea feeding. Biol. Pharm. Bull. 1995;18:1006–1008.10.1248/bpb.18.1006
- Hodgson JM, Puddey IB, Burke V, Beilin LJ, Jordan N. Effects on blood pressure of drinking green and black tea. J. Hypertens. 1999;17:457–463.10.1097/00004872-199917040-00002
- Yang CS, Maliakal P, Meng X. Inhibition of carcinogenesis by tea. Annu. Rev. Pharmacol. Toxicol. 2002;42:25–54.10.1146/annurev.pharmtox.42.082101.154309
- Lee MJ, Wang ZY, Li H, Chen L, Sun Y, Gobbo S, Balentine DA, Yang CS. Analysis of plasma and urinary tea polyphenols in human subjects. Cancer Epidemiol. Biomarkers Prev. 1995;4:393–399.
- Ermakova S, Choi BY, Choi HS, Kang BS, Bode AM, Dong Z. The intermediate filament protein vimentin is a new target for epigallocatechin gallate. J. Biol. Chem. 2005;280:16882–16890.10.1074/jbc.M414185200
- Li M, He Z, Ermakova S, Zheng D, Tang F, Cho YY, Zhu F, Ma WY, Sham Y, Rogozin EA, Bode AM, Cao Y, Dong Z. Direct inhibition of insulin-like growth factor-I receptor kinase activity by (−)-epigallocatechin-3-gallate regulates cell transformation. Cancer Epidemiol. Biomarkers Prev. 2007;16:598–605.10.1158/1055-9965.EPI-06-0892
- He Z, Tang F, Ermakova S, Li M, Zhao Q, Cho YY, Ma WY, Choi HS, Bode AM, Yang CS, Dong Z. Fyn is a novel target of (−)-epigallocatechin gallate in the inhibition of JB6 Cl41 cell transformation. Mol. Carcinog. 2008;47:172–183.10.1002/(ISSN)1098-2744
- Ermakova SP, Kang BS, Choi BY, Choi HS, Schuster TF, Ma WY, Bode AM, Dong Z. (−)-epigallocatechin gallate overcomes resistance to etoposide-induced cell death by targeting the molecular chaperone glucose-regulated protein 78. Cancer Res. 2006;66:9260–9269.10.1158/0008-5472.CAN-06-1586
- Shim JH, Choi HS, Pugliese A, Lee SY, Chae JI, Choi BY, Bode AM, Dong Z. (−)-epigallocatechin gallate regulates CD3-mediated T cell receptor signaling in leukemia through the inhibition of ZAP-70 kinase. J. Biol. Chem. 2008;283:28370–28379.10.1074/jbc.M802200200
- Tachibana H, Koga K, Fujimura Y, Yamada K. A receptor for green tea polyphenol EGCG. Nat. Struct. Mol. Biol. 2004;11:380–381.10.1038/nsmb743
- Fujimura Y, Yamada K, Tachibana H. A lipid raft-associated 67kDa laminin receptor mediates suppressive effect of epigallocatechin-3-O-gallate on FcεRI expression. Biochem. Biophys. Res. Commun. 2005;336:674–681.10.1016/j.bbrc.2005.08.146
- Fujimura Y, Tachibana H, Yamada K. Lipid raft-associated catechin suppresses the FcϵRI expression by inhibiting phosphorylation of the extracellular signal-regulated kinase1/2. FEBS Lett. 2004;556:204–210.10.1016/S0014-5793(03)01432-7
- Umeda D, Yano S, Yamada K, Tachibana H. Green tea polyphenol epigallocatechin-3-gallate signaling pathway through 67-kDa laminin receptor. J. Biol. Chem. 2008;283:3050–3058.10.1074/jbc.M707892200
- Ménard S, Castronovo V, Tagliabue E, Sobel ME. New insights into the metastasis-associated 67 kD laminin receptor. J. Cell. Biochem. 1997;67:155–165.10.1002/(ISSN)1097-4644
- Gauczynski S, Peyrin JM, Haik S, Leucht C, Hundt C, Rieger R, Krasemann S, Deslys JP, Dormont D, Lasmezas CI, Weiss S. The 37-kDa/67-kDa laminin receptor acts as the cell-surface receptor for the cellular prion protein. EMBO J. 2001;20:5863–5875.10.1093/emboj/20.21.5863
- Castronovo V, Taraboletti G, Sobel ME. Functional domains of the 67-kDa laminin receptor precursor. J. Biol. Chem. 1991;266:20440–20446.
- Ardini E, Pesole G, Tagliabue E, Magnifico A, Castronovo V, Sobel ME, Colnaghi MI, Menard S. The 67-kDa laminin receptor originated from a ribosomal protein that acquired a dual function during evolution. Mol. Biol. Evol. 1998;15:1017–1025.10.1093/oxfordjournals.molbev.a026000
- Tanaka M, Narumi K, Isemura M, Abe M, Sato Y, Abe T, Saijo Y, Nukiwa T, Satoh K. Expression of the 37-kDa laminin binding protein in murine lung tumor cell correlates with tumor angiogenesis. Cancer Lett. 2000;153:161–168.10.1016/S0304-3835(00)00365-7
- Zuber C, Knackmuss S, Zemora G, Reusch U, Vlasova E, Diehl D, Mick V, Hoffmann K, Nikles D, Fröhlich T, Arnold GJ, Brenig B, Wolf E, Lahm H, Little M, Weiss S. Invasion of tumorigenic HT1080 cells is impeded by blocking or downregulating the 37-kDa/67-kDa laminin receptor. J. Mol. Biol. 2008;378:530–539.10.1016/j.jmb.2008.02.004
- Martignone S, Menard S, Bufalino R, Cascinelli N, Pellegrini R, Tagliabue E, Andreola S, Rilke F, Colnaghi MI. Prognostic significance of the 67-kilodalton laminin receptor expression in human breast carcinomas. J. Natl. Cancer Inst. 1993;85:398–402.10.1093/jnci/85.5.398
- Tachibana H. Molecular basis for cancer chemoprevention by green tea polyphenol EGCG. Forum Nutr. 2009;61:156–169.10.1159/000212748
- Shammas MA, Neri P, Koley H, Batchu RB, Bertheau RC, Munshi V, Prabhala R, Fulciniti M, Tai YT, Treon SP, Goyal RK, Anderson KC, Munshi NC. Specific killing of multiple myeloma cells by (−)-epigallocatechin-3-gallate extracted from green tea: biologic activity and therapeutic implications. Blood. 2006;108:2804–2810.10.1182/blood-2006-05-022814
- Yang CS, Wang X, Lu G, Picinich SC. Cancer prevention by tea: animal studies, molecular mechanisms and human relevance. Nat. Rev. Cancer. 2009;9:429–439.10.1038/nrc2641
- Kim kJ, Chung JW, Kim KS. 67-kDa laminin receptor promotes internalization of cytotoxic necrotizing factor 1-expressing Escherichia coli K1 into human brain microvascular endothelial cells. J. Biol. Chem. 2005;280:1360–1368.10.1074/jbc.M410176200
- Wang KS, Kuhn RJ, Strauss EG, Ou S, Strauss JH. High-affinity laminin receptor is a receptor for Sindbis virus in mammalian cells. J. Virol. 1992;66:4992–5001.
- Ludwig GV, Kondig JP, Smith JF. A putative receptor for Venezuelan equine encephalitis virus from mosquito cells. J. Virol. 1996;70:5592–5599.
- Thepparit C, Smith DR. Serotype-specific entry of dengue virus into liver cells: identification of the 37-kilodalton/67-kilodalton high-affinity laminin receptor as a dengue virus serotype 1 receptor. J. Virol. 2004;78:12647–12656.10.1128/JVI.78.22.12647-12656.2004
- Akache B, Grimm D, Pandey K, Yant SR, Xu H, Kay MA. The 37/67-kilodalton laminin receptor is a receptor for adeno-associated virus serotypes 8, 2, 3, and 9. J. Virol. 2006;80:9831–9836.10.1128/JVI.00878-06
- Gauczynski S, Nikles D, El-Gogo S, Papy-Garcia D, Rey C, Alban S, Barritault D, Lasmézas CI, Weiss S. The 37‐kDa/67‐kDa laminin receptor acts as a receptor for infectious prions and is inhibited by polysulfated glycanes. J. Infect. Dis. 2006;194:702–709.10.1086/jid.2006.194.issue-5
- Jamieson kV, Wu J, Hubbard SR, Meruelo D. Crystal structure of the human laminin receptor precursor. J. Biol. Chem. 2008;283:3002–3005.10.1074/jbc.C700206200
- Fujimura Y, Sumida M, Sugihara K, Tsukamoto S, Yamada K, Tachibana H. Green tea polyphenol EGCG sensing motif on the 67-kDa laminin receptor. PLoS One. 2012;7:e37942.10.1371/journal.pone.0037942
- Metzger H. The receptor with high affinity for IgE. Immunol. Rev. 1992;125:37–48.10.1111/imr.1992.125.issue-1
- Ravetch JV, Kinet JP. Fc Receptors. Annu. Rev. Immunol. 1991;9:457–492.10.1146/annurev.iy.09.040191.002325
- Turner H, Kinet JP. Signalling through the high-affinity IgE receptor FcεRI. Nature. 1999;402:24–30.10.1038/35037021
- Rivera J. Molecular adapters in FcεRI signaling and the allergic response. Curr. Opin. Immunol. 2002;14:688–693.10.1016/S0952-7915(02)00396-5
- Choi OH, Adelstein RS, Beaven MA. Secretion from rat basophilic RBL-2H3 cells is associated with diphosphorylation of myosin light chains by myosin light chain kinase as well as phosphorylation by protein kinase C. J. Biol. Chem. 1994;269:536–541.
- Pfeiffer JR, Seagrave JC, Davis BH, Deanin GG, Oliver JM. Membrane and cytoskeletal changes associated with IgE-mediated serotonin release from rat basophilic leukemia cells. J. Cell Biol. 1985;101:2145–2155.10.1083/jcb.101.6.2145
- Edgar AJ, Bennett JP. Circular ruffle formation in rat basophilic leukemia cells in response to antigen stimulation. Eur. J. Cell Biol. 1997;73:132–140.
- Fujimura Y, Umeda D, Kiyohara Y, Sunada Y, Yamada K, Tachibana H. The involvement of the 67 kDa laminin receptor-mediated modulation of cytoskeleton in the degranulation inhibition induced by epigallocatechin-3-O-gallate. Biochem. Biophys. Res. Commun. 2006;348:524–531.10.1016/j.bbrc.2006.07.086
- Ludowyke RI, Peleg I, Beaven MA, Adelstein RS. Antigen-induced secretion of histamine and the phosphorylation of myosin by protein kinase C in rat basophilic leukemia cells. J. Biol. Chem. 1989;264:12492–12501.
- Dombrowicz D, Flamand V, Brigman KK, Koller BH, Kinet JP. Abolition of anaphylaxis by targeted disruption of the high affinity immunoglobulin E receptor α chain gene. Cell. 1993;75:969–976.10.1016/0092-8674(93)90540-7
- Sano M, Suzuki M, Miyase T, Yoshino K, Maeda-Yamamoto M. Novel antiallergic catechin derivatives isolated from oolong tea. J. Agric. Food Chem. 1999;47:1906–1910.10.1021/jf981114l
- Tachibana H, Sunada Y, Miyase T, Sano M, Maeda-Yamamoto M, Yamada K. Identification of a methylated tea catechin as an inhibitor of degranulation in human basophilic KU812 cells. Biosci. Biotechnol., Biochem. 2000;64:452–454.10.1271/bbb.64.452
- Suzuki M, Yoshino K, Maeda-Yamamoto M, Miyase T, Sano M. Inhibitory effects of tea catechins and O-methylated derivatives of (−)-epigallocatechin-3- O-gallate on mouse type IV allergy. J. Agric. Food Chem. 2000;48:5649–5653.10.1021/jf000313d
- Maeda-Yamamoto M, Inagaki N, Kitaura J, Chikumoto T, Kawahara H, Kawakami Y, Sano M, Miyase T, Tachibana H, Nagai H, Kawakami T. O-methylated catechins from tea leaves inhibit multiple protein kinases in mast cells. J. Immunol. 2004;172:4486–4492.10.4049/jimmunol.172.7.4486
- Maeda-Yamamoto M, Ema K, Shibuichi I. In vitro and in vivo anti-allergic effects of ‘benifuuki’ green tea containing O-methylated catechin and ginger extract enhancement. Cytotechnology. 2007;55:135–142.10.1007/s10616-007-9112-1
- Fujimura Y, Tachibana H, Maeda-Yamamoto M, Miyase T, Sano M, Yamada K. Antiallergic tea catechin, (−)-epigallocatechin-3-O-(3-O-methyl)-gallate, suppresses FcepsilonRI expression in human basophilic KU812 cells. J. Agric. Food Chem. 2002;50:5729–5734.10.1021/jf025680z
- Fujimura Y, Umeda D, Yano S, Maeda-Yamamoto M, Yamada K, Tachibana H. The 67 kDa laminin receptor as a primary determinant of anti-allergic effects of O-methylated EGCG. Biochem. Biophys. Res. Commun. 2007;364:79–85.10.1016/j.bbrc.2007.09.095
- Lambert JD, Yang CS. Mechanisms of cancer prevention by tea constituents. J. Nutr. 2003;133:3262S–3267S.
- Yano S, Fujimura Y, Umeda D, Miyase T, Yamada K, Tachibana H. Relationship between the biological activities of methylated derivatives of (−)-epigallocatechin-3-O-gallate (EGCG) and their cell surface binding activities. J. Agric. Food Chem. 2007;55:7144–7148.10.1021/jf071176o
- Takeda K, Akira S. Toll-like receptors in innate immunity. Int. Immunol. 2005;17:1–14.
- Takeuchi O, Akira S. Toll-like receptors; their physiological role and signal transduction system. Int. J. Immunopharmacol. 2001;1:625–635.10.1016/S1567-5769(01)00010-8
- Cohen J. The immunopathogenesis of sepsis. Nature. 2002;420:885–891.10.1038/nature01326
- Fujihara M, Muroi M, Tanamoto K, Suzuki T, Azuma H, Ikeda H. Molecular mechanisms of macrophage activation and deactivation by lipopolysaccharide: roles of the receptor complex. Pharmacol. Ther. 2003;100:171–194.10.1016/j.pharmthera.2003.08.003
- Li W, Ashok M, Li J, Yang H, Sama AE, Wang H. A major ingredient of green tea rescues mice from lethal sepsis partly by inhibiting HMGB1. PLoS One. 2007;2:e1153.10.1371/journal.pone.0001153
- Hong Byun E, Fujimura Y, Yamada K, Tachibana H. TLR4 signaling inhibitory pathway induced by green tea polyphenol epigallocatechin-3-gallate through 67-kDa laminin receptor. J Immunol. 2010;185:33–45.10.4049/jimmunol.0903742
- Byun EH, Omura T, Yamada K, Tachibana H. Green tea polyphenol epigallocatechin-3-gallate inhibits TLR2 signaling induced by peptidoglycan through the polyphenol sensing molecule 67-kDa laminin receptor. FEBS Lett. 2011;585:814–820.10.1016/j.febslet.2011.02.010
- Suganuma M, Okabe S, Oniyama M, Tada Y, Ito H, Fujiki H. Wide distribution of [3H](−)-epigallocatechin gallate, a cancer preventive tea polyphenol, in mouse tissue. Carcinogenesis. 1998;19:1771–1776.10.1093/carcin/19.10.1771
- Yoshida A, Hirooka Y, Sugata Y, Nitta M, Manabe T, Ido S, Murakami K, Saha RK, Suzuki T, Ohshima M, Yoshida A, Itoh K, Shimizu K, Oku N, Furuta T, Asakawa T, Wakimoto T, Kan T. Concise synthesis of catechin probes enabling analysis and imaging of EGCg. Chem. Commun. 2011;47:1794–1796.10.1039/c0cc03676e
- Kawai Y. Immunochemical detection of food-derived polyphenols in the aorta: macrophages as a major target underlying the anti-atherosclerotic activity of polyphenols. Biosci. Biotechnol., Biochem. 2011;75:609–617.10.1271/bbb.100785
- Nakayama M, Shigemune N, Tsugukuni T, Tokuda H, Miyamoto T. Difference of EGCg adhesion on cell surface between Staphylococcus aureus and Escherichia coli visualized by electron microscopy after novel indirect staining with cerium chloride. J. Microbiol. Methods. 2011;86:97–103.10.1016/j.mimet.2011.04.010
- Stoeckli M, Chaurand P, Hallahan DE, Caprioli RM. Imaging mass spectrometry: a new technology for the analysis of protein expression in mammalian tissues. Nat. Med. 2001;7:493–496.10.1038/86573
- Shimma S, Sugiura Y, Hayasaka T, Zaima N, Matsumoto M, Setou M. Mass imaging and identification of biomolecules with MALDI-QIT-TOF-based system. Anal. Chem. 2008;80:878–885.10.1021/ac071301v
- Khatib-Shahidi S, Andersson M, Herman JL, Gillespie TA, Caprioli RM. Direct molecular analysis of whole-body animal tissue sections by imaging MALDI mass spectrometry. Anal. Chem. 2006;78:6448–6456.10.1021/ac060788p
- Miura D, Fujimura Y, Tachibana H, Wariishi H. Highly sensitive matrix-assisted laser desorption ionization–mass spectrometry for high-throughput metabolic profiling. Anal. Chem. 2010;82:498–504.10.1021/ac901083a
- Miura D, Fujimura Y, Yamato M, Hyodo F, Utsumi H, Tachibana H, Wariishi H. Ultrahighly sensitive in situ metabolomic imaging for visualizing spatiotemporal metabolic behaviors. Anal. Chem. 2010;82:9789–9796.10.1021/ac101998z
- Yukihira D, Miura D, Saito K, Takahashi K, Wariishi H. MALDI−MS-based high-throughput metabolite analysis for intracellular metabolic dynamics. Anal. Chem. 2010;82:4278–4282.10.1021/ac100024w
- Prideaux B, Stoeckli M. Mass spectrometry imaging for drug distribution studies. J. Proteomics. 2012;75:4999–5013.10.1016/j.jprot.2012.07.028
- Matros A, Mock HP. Mass spectrometry based imaging techniques for spatially resolved analysis of molecules. Front. Plant Sci. 2013;4:89.
- Sang S, Lambert JD, Ho CT, Yang CS. The chemistry and biotransformation of tea constituents. Pharmacol. Res. 2011;64:87–99.10.1016/j.phrs.2011.02.007
- Lambert JD, Sang S, Yang CS. Biotransformation of green tea polyphenols and the biological activities of those metabolites. Mol. Pharmacol. 2007;4:819–825.10.1021/mp700075m
- Yukihira D, Miura D, Fujimura Y, Umemura Y, Yamaguchi S, Funatsu S, Yamazaki M, Ohta T, Inoue H, Shindo M, Wariishi H. MALDI efficiency of metabolites quantitatively associated with their structural properties: a quantitative structure–property relationship (QSPR) approach. J. Am. Soc. Mass. Spectrom. 2014;25:1–5.10.1007/s13361-013-0772-0
- Prideaux B, Dartois V, Staab D, Weiner DM, Goh A, Via LE, Barry III CE, Stoeckli M. High-sensitivity MALDI-MRM-MS imaging of moxifloxacin distribution in tuberculosis-infected rabbit lungs and granulomatous lesions. Anal. Chem. 2011;83:2112–2118.10.1021/ac1029049
- Norris JL, Caprioli RM. Analysis of tissue specimens by matrix-assisted laser desorption/ionization imaging mass spectrometry in biological and clinical research. Chem. Rev. 2013;113:2309–2342.10.1021/cr3004295
- Chacon A, Zagol-Ikapitte I, Amarnath V, Reyzer ML, Oates JA, Caprioli RM, Boutaud O. On-tissue chemical derivatization of 3-methoxysalicylamine for MALDI-imaging mass spectrometry. J. Mass Spectrom. 2011;46:840–846.10.1002/jms.1958
- Manier ML, Reyzer ML, Goh A, Dartois V, Via LE, Barry 3rd CE, Caprioli RM. Reagent precoated targets for rapid in-tissue derivatization of the anti-tuberculosis drug isoniazid followed by MALDI imaging mass spectrometry. J. Am. Soc. Mass. Spectrom. 2011;22:1409–1419.10.1007/s13361-011-0150-8
- Louie KB, Bowen BP, McAlhany S, Huang Y, Price JC, Mao JH, Hellerstein M, Northen TR. Mass spectrometry imaging for in situ kinetic histochemistry. Sci. Rep. 2013;3:1656.
- Harada T, Yuba-Kubo A, Sugiura Y, Zaima N, Hayasaka T, Goto-Inoue N, Wakui M, Suematsu M, Takeshita K, Ogawa K, Yoshida Y, Setou M. Visualization of volatile substances in different organelles with an atmospheric-pressure mass microscope. Anal. Chem. 2009;81:9153–9157.10.1021/ac901872n
- Zavalin A, Todd EM, Rawhouser PD, Yang J, Norris JL, Caprioli RM. Direct imaging of single cells and tissue at sub-cellular spatial resolution using transmission geometry MALDI MS. J. Mass Spectrom. 2012;47:1473–1481.10.1002/jms.3108
- Yasunaga M, Furuta M, Ogata K, Koga Y, Yamamoto Y, Takigahira M, Matsumura Y. The significance of microscopic mass spectrometry with high resolution in the visualisation of drug distribution. Sci. Rep. 2013;3:3050.
- Cerruti CD, Benabdellah F, Laprévote O, Touboul D, Brunelle A. MALDI imaging and structural analysis of rat brain lipid negative ions with 9-aminoacridine matrix. Anal. Chem. 2012;84:2164–2171.10.1021/ac2025317
- Seeley EH, Caprioli RM. 3D imaging by mass spectrometry: a new frontier. Anal. Chem. 2012;84:2105–2110.10.1021/ac2032707
- Liu X, Ide JL, Norton I, Marchionni MA, Ebling MC, Wang LY, Davis E, Sauvageot CM, Kesari S, Kellersberger KA, Easterling ML, Santagata S, Stuart DD, Alberta J, Agar JN, Stiles CD, Agar NY. Molecular imaging of drug transit through the blood–brain barrier with MALDI mass spectrometry imaging. Sci. Rep. 2013;3:2859.
- Le Gall G, Colquhoun IJ, Defernez M. Metabolite profiling using 1H NMR spectroscopy for quality assessment of green tea, Camellia sinensis (L.). J. Agric. Food. Chem. 2004;52:692–700.10.1021/jf034828r
- Roessner U, Luedemann A, Brust D, Fiehn O, Linke T, Willmitzer L, Fernie A. Metabolic profiling allows comprehensive phenotyping of genetically or environmentally modified plant systems. Plant Cell. 2001;13:11–29.10.1105/tpc.13.1.11
- Fernie AR, Trethewey RN, Krotzky AJ, Willmitzer L. Innovation: metabolite profiling: from diagnostics to systems biology. Nat. Rev. Mol. Cell Biol. 2004;5:763–769.10.1038/nrm1451
- Luthria DL, Lin LZ, Robbins RJ, Finley JW, Banuelos GS, Harnly JM. Discriminating between cultivars and treatments of broccoli using mass spectral fingerprinting and analysis of variance principal component analysis. J. Agric. Food. Chem. 2008;56:9819–9827.10.1021/jf801606x
- Okazaki K, Oka N, Shinano T, Osaki M, Takebe M. Differences in the metabolite profiles of spinach (Spinacia oleracea L.) leaf in different concentrations of nitrate in the culture solution. Plant Cell Physiol. 2008;49:170–177.
- Heuberger AL, Lewis MR, Chen MH, Brick MA, Leach JE, Ryan EP. Metabolomic and functional genomic analyses reveal varietal differences in bioactive compounds of cooked rice. PLoS One. 2010;5:e12915.10.1371/journal.pone.0012915
- Cuadros-Inostroza A, Giavalisco P, Hummel J, Eckardt A, Willmitzer L, Peña-Cortés H. Discrimination of wine attributes by metabolome analysis. Anal. Chem. 2010;82:3573–3580.10.1021/ac902678t
- Kim SH, Cho SK, Hyun SH, Park HE, Kim YS, Choi HK. Metabolic profiling and predicting the free radical scavenging activity of guava (Psidium guajava L.) leaves according to harvest time by 1H-nuclear magnetic resonance spectroscopy. Biosci. Biotechnol., Biochem. 2011;75:1090–1097.10.1271/bbb.100908
- Park SY, Park WT, Park YC, Ju JI, Park SU, Kim JK. Metabolomics for the quality assessment of lycium Chinense fruits. Biosci. Biotechnol., Biochem. 2012;76:2188–2194.10.1271/bbb.120453
- Raskin I, Ribnicky DM, Komarnytsky S, Ilic N, Poulev A, Borisjuk N, Brinker A, Moreno DA, Ripoll C, Yakoby N, O’Neal JM, Cornwell T, Pastor I, Fridlender B. Plants and human health in the twenty-first century. Trends Biotechnol. 2002;20:522–531.10.1016/S0167-7799(02)02080-2
- Scalbert A, Brennan L, Fiehn O, Hankemeier T, Kristal BS, van Ommen B, Pujos-Guillot E, Verheij E, Wishart D, Wopereis S. Mass-spectrometry-based metabolomics: limitations and recommendations for future progress with particular focus on nutrition research. Metabolomics. 2009;5:435–458.10.1007/s11306-009-0168-0
- Kurita I, Maeda-Yamamoto M, Tachibana H, Kamei M. Antihypertensive effect of benifuuki tea containing O-methylated EGCG. J. Agric. Food Chem. 2010;58:1903–1908.10.1021/jf904335g
- Ku KM, Choi JN, Kim J, Kim JK, Yoo LG, Lee SJ, Hong YS, Lee CH. Metabolomics analysis reveals the compositional differences of shade grown tea (Camellia sinensis L.). J. Agric. Food Chem. 2010;58:418–426.10.1021/jf902929h
- Ku KM, Kim J, Park HJ, Liu KH, Lee CH. Application of metabolomics in the analysis of manufacturing type of pu-erh tea and composition changes with different postfermentation year. J. Agric. Food Chem. 2010;58:345–352.10.1021/jf902818c