Abstract
The transcriptional regulation of the MAPK mpkA and cell wall-related genes in Aspergillus nidulans differs from that of their counterparts in Saccharomyces cerevisiae. The A. nidulans MAPK MpkB is putatively orthologous to the yeast MAPKs Kss1p and Fus3p. To investigate MpkB and its contribution to cell wall integrity in A. nidulans, we constructed mpkB-disruptant (mpkB∆) strains. We previously showed that mpkA∆ strains exhibited reduced colony growth and increased sensitivity to the β-1,3-glucan synthase inhibitor micafungin. Like mpkA∆ strains, mpkB∆ strains exhibited slight growth retardation and increased sensitivity to micafungin. Although MpkB-dependent signaling modulated the transcription of some cell wall-related genes, the sugar composition of cell wall fractions was similar among wild-type, mpkA∆, and mpkB∆ strains. To elucidate the relationship between MpkA and MpkB pathways, we compared conditional mutants of mpkB with those with mpkA deletion. Sensitivity testing suggested that MpkA and MpkB additively contribute to micafungin activity in A. nidulans.
Graphical Abstract
Phenotypic analyses of the A. nidulans mpkA∆, mpkB∆, and conditional-mpkB with mpkA∆ strains revealed that the MpkA and MpkB additively contribute to micafungin activity. (24 words, 147 characters)
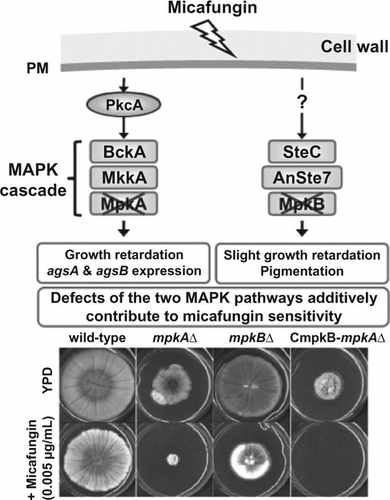
The fungal cell wall is a complex structure that is essential for the maintenance of cellular shape and integrity, prevention of cell lysis, and protection against adverse environmental conditions. Fungal cells constantly remodel the rigid structure of their cell walls during growth and development. Cell wall biogenesis and its regulation by cell signaling have been well characterized in the yeast Saccharomyces cerevisiae.Citation1,2) The activation of the cell wall integrity signaling (CWIS) pathway leads to activation of the downstream mitogen-activated protein kinase (MAPK) cascade via Pkc1p and the small G protein Rho1p, which acts on Pkc1p.Citation2,3) The MAPK Mpk1 protein activates the transcription factor Rlm1p, which regulates the transcription of at least 25 genes involved in cell wall biogenesis, including β-1,3-glucan synthase genes and chitin synthase genes.Citation2,4)
In the model filamentous fungus Aspergillus nidulans, disruptants of mpkA, rlmA (orthologs of S. cerevisiae MPK1 and RLM1, respectively), and Answi4 and Answi6 (orthologs of S. cerevisiae SWI4 and SWI6, which together encode the Mpk1p-activating transcription factor Swi4p–Swi6p complex) have been constructed.Citation5,6) Treatment of these disruptants with the β-1,3-glucan synthase inhibitor micafungin transiently up-regulates the transcription of most cell wall-related genes, including the β-1,3-glucan synthase gene fksA and several chitin synthase genes.Citation6) Therefore, transcription of fksA and of several chitin synthase genes is independent of RlmA and AnSwi4/AnSwi6, suggesting their transcriptional regulation by a non-MpkA pathway in A. nidulans. In the rice blast fungus Magnaporthe grisea, α-1,3-glucan was undetectable in the cell wall of the deletion mutant of Mps1p MAPK, which is an ortholog of Mpk1p/Slt2p in S. cerevisiae.Citation7) In addition, the activation of Mps1p was required for α-1,3-glucan accumulation in response to a plant wax derivative, 1,16-hexadecanediol.Citation7) Conversely, several cell wall polysaccharides, such as β-1,3-glucan and chitin, were detected regardless of the presence of 1,16-hexadecanediol.Citation7) These results suggest that the α-1,3-glucan synthase gene of M. grisea fully depends on Mps1p,Citation7) as occurs in A. nidulans.Citation6) Therefore, the regulation of β-1,3-glucan and chitin biosynthesis by the CWIS pathway is likely conserved in filamentous fungi, and the regulation of fungal cell wall-related genes via CWIS markedly differs from that in S. cerevisiae.Citation6) Despite the many studies addressing the CWIS pathway in filamentous fungi,Citation8–11) the mechanism underlying the transcriptional regulation of the β-1,3-glucan synthase and chitin synthase genes remains largely unknown.
Filamentous fungi generally contain three conserved MAPK pathways, called the HOG, MpkA, and MpkB pathways in A. nidulans.Citation5,6,12–15) The A. nidulans HOG pathway is involved in adaptation to high osmolarity,Citation12) the MpkA pathway regulates the cell wall α-1,3-glucan synthase gene,Citation6) and the MpkB pathway regulates sexual development and secondary metabolism.Citation13,14) In several phytopathogenic fungi, MAPK genes orthologous to yeast Fus3/Kss1 have been characterized and appear to be well conserved in the regulation of plant infection processes.Citation16–20) For example, Chk1, an ortholog of yeast Fus3/Kss1 in the southern corn leaf blight fungus Cochliobolus heterostrophus, regulates not only sexual–asexual development and pathogenicity but also adaptation to oxidative and heavy-metal stresses.Citation20)
MAPK genes orthologous to yeast Fus3/Kss1 apparently are well conserved in regard to their involvement in sexual development in filamentous fungi. However, the role of mpkB in cell wall biogenesis in A. nidulans remains unclear. In addition, although the phosphorylation motif of MpkB is the same as that of MpkA (Thr-Glu-Tyr),Citation21) the relationship between MpkA and MpkB has not been characterized in Aspergillus species, including A. nidulans.
In the present study, we functionally analyzed the A. nidulans mpkB gene in the context of cell wall biogenesis. To this end, we constructed a deletion mutant of mpkB (mpkB∆), which we used to analyze the transcription of various cell wall-related genes. We also placed the mpkB gene under the control of the alcA promoter to create a strain in which the expression of MpkB was conditional (CmpkB). We then deleted mpkA from the CmpkB strain to elucidate the relationship between mpkA and mpkB. We examined susceptibility of these strains to cell wall stress-inducing compounds. These analyses revealed that despite its contribution to sensitivity to micafungin, mpkB is not directly involved in the CWIS pathway. Moreover, we deduced that MpkA and MpkB have additive effects on radial growth and micafungin sensitivity. Considering our combined results, we discuss the CWIS pathway in A. nidulans.
Materials and methods
Strains and growth media
Throughout this study, A. nidulans ABPU1 (biA1, pyrG89, wA3, argB2, pyroA4) with ligD∆ (ligD∆::ptrA) was used as the control strain and for all genetic manipulations. A. oryzae RIB40 was used for DNA extraction to amplify the A. oryzae wild-type argB gene, which was used as a selectable marker in A. nidulans. For the A. nidulans mpkA and mpkB deletion studies, ABPU1 cells were transformed with the gene-replacement constructs described in the following subsection. Czapek–Dox (CD) and YPD media were used for standard culture.Citation6) CD medium containing 0.1 M threonine and 0.1% fructose instead of 1% glucose as a carbon source (designated CDTF) was used for up-regulation of alcA promoter-driven mpkB.Citation22) To investigate gene expression, conidia (105 mL−1) were inoculated into liquid medium (CD, YPD, or CDTF) and cultured at 37 °C for 24 h, after which mycelia were harvested. DNA and RNA extraction, reverse transcriptase reactions, and transformation analysis were all performed as described previously.Citation23)
Construction of gene-replacement cassettes
The gene-replacement cassettes for the mpkA and mpkB deletion mutants and the alcA promoter-driven mpkB (CmpkB) strain were constructed using a previously reported PCR-based fusion strategy.Citation24) The sequences of all primers used in this study are listed in Table S1. To disrupt a gene in A. nidulans, we replaced it with A. oryzae argB or A. nidulans pyrG as a selectable marker. To disrupt the mpkB gene, in the first round of PCR, the 5′ non-coding region (amplicon 1; primers mpkB-5′F and argBmpkB-5′R) and 3′ non-coding region (amplicon 2; primers argBmpkB-3′F and mpkB-3′R) of mpkB were amplified from A. nidulans ABPU1 genomic DNA, and the argB gene (amplicon 3; primers argB-F and argB-R) was amplified from A. oryzae genomic DNA. The primers argBmpkB-5′R and argBmpkB-3′F were chimeric oligonucleotides that contained reverse complementary sequences for fusion of the PCR products. The three resulting PCR products were gel-purified and used as substrates for a second round of PCR, which used the primers mpkB-5′F and mpkB-3′R and fused the three first-round fragments into a disruption cassette. All PCR reactions were performed in a GeneAmp PCR System 9700 (Applied Biosystems, Foster City, CA, USA) using PrimeSTAR HS DNA polymerase (Takara, Tokyo, Japan). The PCR-generated disruption cassette was gel-purified and used to transform the ABPU1 strain.
To construct the gene-replacement cassette for the alcA promoter-driven mpkB (CmpkB) strain, a fragment containing the 5′ non-coding region of mpkB and one including the open reading frame of mpkB were PCR-amplified using A. nidulans ABPU1 genomic DNA as a template and primers listed in Table S1. In addition, the pyrG-alcA(p) cassette,Citation24) which contains the A. nidulans pyrG marker and the alcA promoter (originally derived from A. nidulans FGSC A89 strain), was amplified by PCR using the primers pyrG-F and PalcA-R (Table S1). These three PCR-amplified fragments were used as substrates for the second-round PCR fusion (Fig. S2). The resulting major PCR product was gel-purified and used to transform the ABPU1 strain.
To construct the disruption cassette for mpkA in the ABPU1 and the CmpkB strains, the same PCR fusion strategy was used. In the first round of PCR, the primers mpkA-5′F and argBmpkA-5′R (for amplicon 1), argBmpkA-3′F and mpkA-3′R (for amplicon 2), and argB-F and argB-R (for amplicon 3) were used (Table S1). The primers mpkA-5′F and mpkA-3′R were used for the second round of PCR amplification. The resulting major PCR product was gel-purified and used to transform the CmpkB or the ABPU1 strains. Fungal transformation experiments were performed as described previously.Citation6)
Quantitative RT-PCR
The methods for real-time RT-PCR have been described previously.Citation24) Primer sets for quantifying cell wall-related gene expressionCitation6,24) are listed in Table S1. The gene for histone H2B was used as a normalization reference (internal control) for target gene expression ratios. A sample from unmanipulated ABPU1 cells cultured in CD liquid medium was set as a calibrator in each experiment. Statistical analyses were based on Welch’s t-test, and significance was defined as a p value of <0.01.
Growth inhibition testing of mutants
The sensitivity of strains to the cell wall stress-inducing compounds micafungin and calcofluor white (CFW) was evaluated by plotting the dose response for colony growth using the plate-dilution method. The chemicals were added from 1000-fold concentrated stock solutions in water (micafungin) or 0.1 N NaOH (CFW). Conidial suspensions of each mutant (1 × 103 cells) were spotted on the centers of plates containing micafungin (0.005, 0.01, 0.05, or 0.1 μg/mL) or CFW (1, 5, or 10 μg/mL). To test the sensitivity of the strains to oxidative and heavy-metal stresses, CD plates containing 2–4 mM hydrogen peroxide, 0.8–1.6 mM CuSO4, or 4.0–4.8 mM FeCl3 were used. The dose response was determined 5 d after incubation at 37 °C by plotting the sizes of the colonies on treated media as percentages of those on control medium. At least three replicates were performed for each experiment.
Fractionation of cell walls by alkali treatment
The steps used for cell wall fractionation have been described previously.Citation24) Mycelia cultivated in liquid CD medium at 37 °C for 24 h were collected and freeze-dried. According to their solubility in alkali, the freeze-dried mycelia were fractionated into four fractions: hot-water-soluble (HW); alkali-soluble 1 (AS1), which contained the water-soluble components after dialysis of the alkali-soluble fraction; alkali-soluble 2 (AS2), which contained the water-insoluble components after dialysis of the alkali-soluble fraction; and alkali-insoluble (AI). All fractions were freeze-dried, and each fraction was weighed.
Quantitative determination of the carbohydrate composition of the cell wall fractions
The procedure for quantification of the carbohydrate composition of the cell wall fractions has been described previously.Citation24) Each freeze-dried cell wall fraction (10 mg dry weight) was hydrolyzed in sulfuric acid, and the carbohydrate composition was determined using high-performance chromatography with a pulsed electrochemical detector and an anion-exchange column (Carbo PAC PA-1, 4 × 25 mm; Dionex, Sunnyvale, CA, USA).
Results
Isolation and characterization of an A. nidulans mpkB deletion strain (mpkBΔ)
To investigate the roles of the mpkB gene in A. nidulans, we generated mutant strains from which mpkB had been deleted (mpkB∆) using the targeted replacement method and selecting double-crossover events after homologous recombination (Fig. S1A). Correct integration of the deletion cassettes and gene disruption were confirmed by PCR (Fig. S1B). The color of mpkB∆ colonies was altered (Fig. (A)), and the colony diameter of the strains lacking mpkB was approximately 70% of that of the wild-type strain (Fig. (B)). No differences in hyphal morphology or conidiation on CD plates were noted between the mpkB∆ and wild-type strains (data not shown).
Fig. 1. Phenotype of the mpkB∆ strain of A. nidulans.
Notes: (A) Colony growth of the wild-type (left) and mpkB∆ strains (right) on CD plates. (B) Colony diameter of the wild-type and mpkB∆ strains on CD plates.
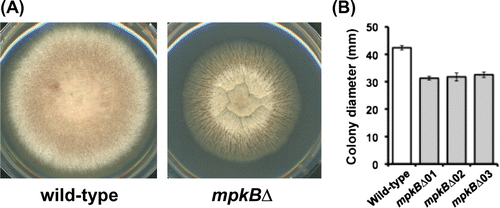
To investigate the functions of mpkB in adaptation to oxidative and heavy-metal stresses, we tested the sensitivities of the mpkB∆ strain to H2O2, CuSO4, and FeCl3. No significant differences in growth rate on CD plates containing H2O2, CuSO4, and FeCl3 were noted between the mpkB∆ and wild-type strains (Fig. S2).
To investigate the functions of mpkB in CWIS, we tested the sensitivities of the mpkB∆ strain to cell wall stress-inducing compounds. The sensitivity of the mpkB∆ strain to micafungin, an inhibitor of β-1,3-glucan synthase, was greater than that of the control strain (Fig. (A)). Specifically, mpkB∆ strain was unable to grow on CD plates containing 0.05 μg/mL micafungin, whereas the growth rate of the wild-type strain on CD plates containing 0.05 μg/mL micafungin was still approximately 15% of that of control cells on drug-free CD plates (Fig. (A)). In contrast to the inhibitory effect of micafungin, the mpkB∆ strain was insensitive to CFW, which is a chitin-binding agent (Fig. (B)).
Fig. 2. Sensitivities of the wild-type and mpkB∆ strains to micafungin and CFW.
Notes: (A) Growth rate after 5 d on CD medium containing micafungin was calculated relative to that of each strain on inhibitor-free CD medium. (B) Growth rate after 5 d on CD medium containing CFW was calculated relative to that of each strain on CFW-free CD medium. Error bars represent the standard error of the mean (n = 3).
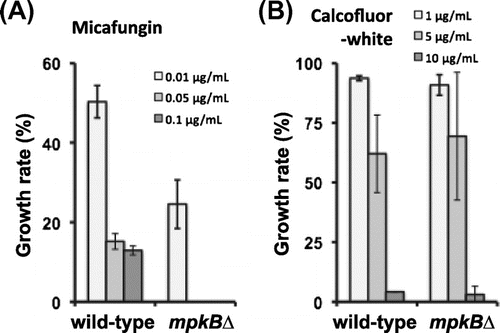
Transcription profiles of genes involved in cell wall biosynthesis in the mpkB∆ strain
We then tested whether deletion of the mpkB gene affected the transcription of genes associated with cell wall biogenesis. The wild-type and mpkB∆ strains were treated with 0.1 μg/mL of micafungin for 0, 30, 60, or 120 min, after which the transcript levels of agsA and agsB (two α-1,3-glucan synthase genes); fksA (a β-1,3-glucan synthase gene); gelA and gelB (two β-1,3-glucanosyl transferase genes); chsA, chsB, chsC, chsD, csmA, and csmB (chitin synthase genes); and gfaA (a glutamine–fructose-6-phosphate amidotransferase gene) were analyzed using quantitative RT-PCR.
The transcript levels of all genes analyzed, except fksA, gelA, and gfaA, were similar between the wild-type and mpkB∆ strains under normal growth conditions (no micafungin treatment) (Fig. , the 0-min time point). In contrast, the transcript levels of fksA, gelA, and gfaA were significantly but slightly (<2-fold) increased in the mpkB∆ strain compared with the wild-type strain under normal growth conditions (Fig. , the 0-min time point). These results suggest that a cell wall component such as β-1,3-glucan or chitin was altered in the mpkB∆ strain, and our experiments that address this possibility are presented in a later section.
Fig. 3. Transcription of cell wall-related genes in the wild-type and mpkB∆ strains after treatment with 0.1 μg/mL of micafungin.
Notes: Levels of transcription of the indicated genes were determined by quantitative RT-PCR using total RNA and the gene-specific primers reported previously by Fujioka et al.Citation5) (Table S1). The transcription of histone H2B gene tended to be reduced in the mpkB∆ strain even in the absence of micafungin (approximately 80% of that of the wild-type strain). According to the differences of the expression levels of histone H2B gene in each strain, the transcription of each gene was normalized. The value shown is the normalized expression level relative to that of the histone H2B gene in each strain. Error bars represent the standard deviations (n ≥ 3). *, Value significantly (p < 0.01) different from that of the wild-type strain.
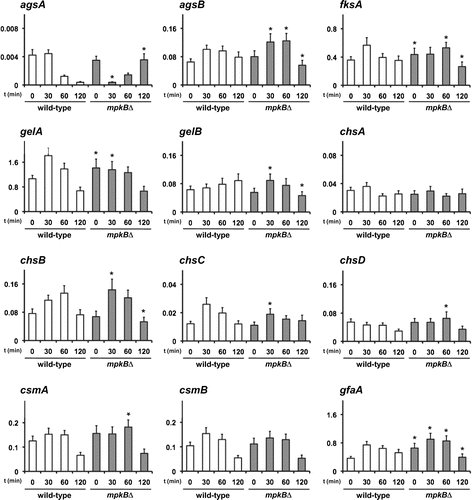
Next, we examined the transcription patterns of cell wall-related genes after treatment with micafungin (Fig. ). The transcription patterns of all genes analyzed, except agsA, gelA, and gelB, were similar between the wild-type and mpkB∆ strains, although the transcript levels of some genes showed significant but slight (<2-fold) differences over time (Fig. ). Transcription of these genes tended to be up-regulated at 30–60 min of micafungin treatment in both the wild-type and mpkB∆ strains (Fig. ). In addition, gelA transcripts were transiently up-regulated at 30 or 60 min of micafungin treatment in the wild-type strain but were relatively unchanged in the mpkB∆ strain during the same period (Fig. ). The level of gelA transcripts in the mpkB∆ strain was higher than that in the wild-type strain under normal growth conditions (Fig. ) but was similar between the wild-type and mpkB∆ strains at 120 min of micafungin treatment (Fig. ). The gelB transcript level in the wild-type strain continued to increase at least until 120 min of micafungin treatment but had returned to baseline levels by this time point in the mpkB∆ strain (Fig. ). These results suggested that the transcription of gelA and gelB was not fully dependent on MpkB. Transcript levels of agsA were similar between the wild-type and mpkB∆ strains under normal growth conditions (Fig. ) but differed after treatment with micafungin (Fig. ). Specifically, the levels of the agsA transcript in the mpkB∆ strain decreased transiently but subsequently recovered after 120-min treatment with micafungin, whereas agsA transcripts in wild-type cells remained at low levels. These results suggest that the transcription of agsA, gelA, and gelB is partially dependent on MpkB, whereas that of other cell wall-related genes is independent of the MpkB pathway.
Construction of conditional-mpkB strains with mpkA deletion in A. nidulans
To analyze the relationships of the MAP kinases MpkA and MpkB in A. nidulans, we tried to generate double-deletion mutants of mpkA and mpkB genes but remained unsuccessful after many attempts. This result suggested that the simultaneous deletion of mpkA and mpkB is lethal. We therefore decided to generate a strain conditionally mutant for mpkB from an mpkA deletion strain (CmpkB-mpkA∆), in which the transcription of mpkB was regulated under the control of the A. nidulans alcA promoter in the context of an mpkA-null genetic background.
We first generated several CmpkB strains. The replacement cassette, which enabled the replacement of the native mpkB promoter (−1000 bp from the start codon) by the alcA promoter and contained the pyrG selectable marker, was constructed and introduced into the wild-type strain (Fig. S3A). The genomic structure of the resulting conditional-mpkB strains was confirmed by PCR (Fig. S3B). Three independent mutant lines were established. To confirm the regulation of mpkB transcription in the CmpkB strains, we performed transcriptional analysis by quantitative RT-PCR after their growth in three different media. The alcA promoter activates gene expression in the presence of carbon sources such as ethanol, glycerol, and threonine and represses it in the presence of glucose.Citation25) Accordingly, after 24 h of culture in YPD liquid medium (1% glucose), mpkB transcript levels were lower in the CmpkB strains than in the wild-type strain (Fig. S4, left). The CmpkB strains also showed low mpkB expression in CD (1% glucose) medium, albeit somewhat higher than that in YPD medium (Fig. S4, center). Culture in CDTF medium (0.1 M threonine, 0.1% fructose) led to considerably higher mpkB transcription in the CmpkB strains than in the control strain (Fig. S4, right). These results showed that the alcA promoter in the CmpkB strains successfully regulated mpkB expression in response to the carbon sources in the medium.
We then compared the phenotypes of the mpkB∆ and CmpkB strains under mpkB-repressing and -inducing conditions. Colony growth and sensitivity to micafungin did not differ appreciably between the mpkB∆ and the CmpkB strains on YPD (repressing condition) (Figs. and ). In addition, colony growth on CDTF (inducing condition) plates did not differ between the wild-type and CmpkB strains (Fig. ). According to these results, we decided to use the CmpkB strain as a parental strain for an mpkA deletion (CmpkB-mpkA∆). The disruption cassettes, which replaced the coding region of mpkA with the A. oryzae argB gene as a selectable marker in A. nidulans, were introduced into the CmpkB strain (Fig. S5A). Correct integration of the cassettes and gene disruption were confirmed by PCR (Fig. S5B). In addition, we generated mpkA deletion strains (mpkA∆) from the wild-type strain (Fig. S5B) to compare phenotypic differences among mpkA∆, mpkB∆, and the CmpkB-mpkA∆ strains.
Fig. 4. Colony growth of the mpkA∆, mpkB∆, CmpkB (conditional-mpkB), and CmpkB-mpkA∆ (CmpkB with mpkA∆) strains on YPD medium (mpkB-repressing condition for CmpkB and CmpkB-mpkA∆ strains) for 5 d.
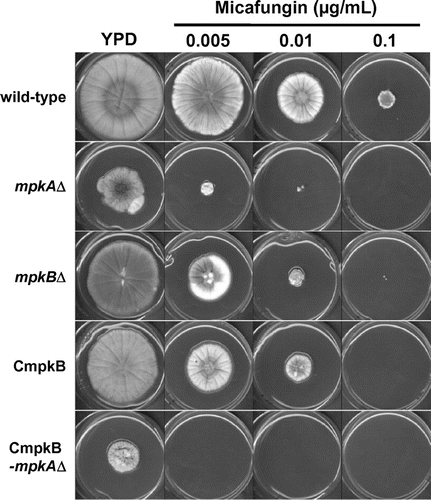
Fig. 5. Colony diameter of the mpkA∆, mpkB∆, CmpkB (conditional-mpkB), and CmpkB-mpkA∆ (CmpkB with mpkA∆) strains on CDTF medium (mpkB-inducing condition for CmpkB and CmpkB-mpkA∆ strains) and YPD medium (mpkB-repressing condition for CmpkB and CmpkB-mpkA∆ strains) for 5 d.
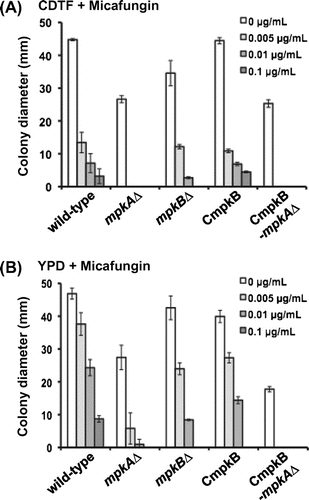
The growth of the mpkA∆ colonies on YPD medium was greatly decreased compared with that of the wild-type strain (Figs. and ). Specifically, the colony diameter of the mpkA∆ strain on YPD medium was approximately 60% of that of the wild-type strain (Fig. ). In addition, the growth rate of the mpkA∆ strain on YPD medium containing 0.005 μg/mL micafungin was approximately 20% of that on YPD medium only (Fig. ); in comparison, the growth rate of the wild-type strain on 0.005 μg/mL micafungin in YPD was approximately 80%. The mpkB∆ and CmpkB strains showed slightly reduced colonial growth on YPD medium and increased sensitivity to micafungin (Figs. and ). The growth rates of the mpkB∆ strain on YPD medium containing 0.005 and 0.01 μg/mL micafungin were approximately 55% and 20%, respectively, of that in YPD medium only (Fig. ), whereas those of the wild-type strain were approximately 80% and 50%, respectively.
Growth on YPD medium was further retarded in the CmpkB-mpkA∆ strain compared with both the mpkA∆ strain and the mpkB∆ strain. Specifically, the colony diameter of the CmpkB-mpkA∆ strain on YPD medium was approximately 40% of that of the wild-type strain (Fig. ). In addition, the CmpkB-mpkA∆ strain failed to grow on YPD medium containing 0.005 μg/mL micafungin. These findings suggest that MpkA and MpkB had additive effects on colony growth and sensitivity to micafungin.
Comparison of cell wall components in the mpkA∆ and mpkB∆ strains
Cell wall components of the wild-type, mpkA∆, and mpkB∆ strains were fractionated according to their solubility in alkali. We previously reported that the alkali-soluble fraction of A. nidulans is composed mainly of α-1,3-glucan and that the alkali-insoluble fraction comprises primarily chitin and β-1,3-glucan.Citation24) The cell wall components from the A. nidulans wild-type, mpkA∆, and mpkB∆ strains were separated into four fractions, and the weight ratios of the fractions were obtained (Table S2). The total recovery rate of the four fractions during the fractionation was 50–63% of the total cell dry weight in each strain. Next, we determined the sugar compositions in the four fractions for each strain (Fig. ). The HW fraction contained glucose, galactose, and mannose, which together accounted for less than 5% of the HW fraction (by weight) in each strain (Fig. (A)). The AS1 fraction, which contained the water-soluble components after dialysis of the alkali-soluble fraction,Citation24) was composed of glucose, galactose, and mannose (Fig. (B)). However, these sugars together accounted for less than 1% of the total AS1 fraction weight in each strain. Glucose again was the predominant sugar in the AS2 fraction, the weight of which did not differ between strains (Fig. (C)).
Fig. 6. Composition of carbohydrate of each cell wall fraction.
Notes: The wild-type, mpkA∆, and mpkB∆ strains were cultured in CD liquid medium at 37 °C for 24 h. Monosaccharide compositions of the (A) hot-water-soluble (HW), (B) alkali-soluble 1 (AS1), (C) alkali-soluble 2 (AS2), and (D) alkali-insoluble (AI) cell wall fractions are expressed as percentages of the total dry weight of each fraction. Error bars represent the standard deviations (n ≥ 3). Glc, glucose; Gal, galactose; Man, mannose; GlcN, glucosamine.
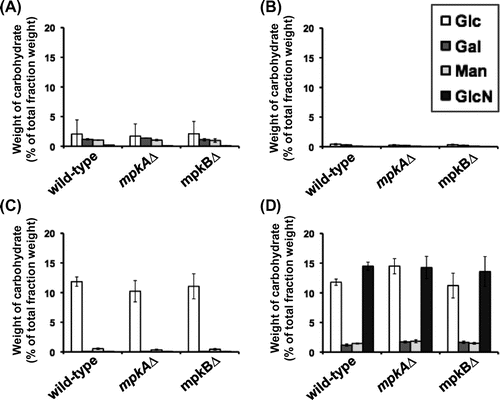
The AI fraction contained glucose, galactose, mannose, and glucosamine in all strains (Fig. D). In each strain, the glucose and glucosamine contents of the AI fraction each accounted for about 10–15% of the dry weight of the AI fraction, and the galactose and mannose contents each accounted for less than 2%. The sugar compositions of all fractions did not differ notably among the wild-type, mpkA∆, and mpkB∆ strains (Fig. ). The combined results from the fractionation and the quantification of sugar contents revealed that the cell wall composition of the mpkA∆ and mpkB∆ strains did not differ greatly from that of the wild-type strain.
Because the CmpkB-mpkA∆ strain exhibited severe growth retardation in liquid culture, biochemical analysis of cell wall components could not perform in the CmpkB-mpkA∆ strain.
Discussion
In the present study, we constructed deletion mutants of the mpkB gene in A. nidulans. The mpkB∆ strain of A. nidulans showed slight radial growth retardation, altered colony color, and increased sensitivity to micafungin, compared with the wild-type strain. Other researchers have reported phenotypic changes in mpkB deletion mutants in A. nidulans.Citation13–15) Jun et al.Citation15) demonstrated that the colony growth rate of their mpkB deletion mutant was slower than that of the wild-type strain, as was the case for our mpkB∆ strain, although they did not report a change in colony color.Citation15) Jun et al. Citation15) also demonstrated that the phenotypes of the mpkB deletion mutant resembled those of the steC deletion mutant of A. nidulans.Citation15,26) Deletion of A. nidulans steC, an ortholog of the yeast Ste11 MAPKK kinase gene, not only inhibited heterokaryon formation and blocked cleistothecium development but also decreased hyphal growth and altered colony color.Citation26) Together with these observations, our results suggest that hyphal development and pigmentation are regulated by the SteC MAPKK kinase pathway via MpkB in A. nidulans. In addition, Atoui et al.Citation14) demonstrated that the mpkB of A. nidulans regulated secondary metabolism.Citation14) The mpkB∆ strain of A. nidulans produced low levels of sterigmatocystin and penicillin compared with the levels produced by the control strain.Citation14) Levels of transcripts of aflR, which is a known sterigmatocystin transcriptional regulator gene, and laeA, a global regulator of secondary metabolic gene clusters in Aspergillus, including the sterigmatocystin and penicillin gene clusters, were dramatically reduced in the mpkB∆ strain of A. nidulans.Citation14) Although the transcript levels of aflR and laeA were not altered in our mpkB∆ strain (data not shown), the hyphal pigmentation observed in our A. nidulans mpkB∆ strain might be attributable to alterations of secondary metabolism.
Several orthologs of Fus3/Kss1 have been characterized in filamentous fungi.Citation16–20,27) In particular, Fus3/Kss1 orthologs in plant pathogens such as M. grisea and Colletotrichum lagenarium are involved in the formation of infection structures (appresorila) and in infectious processes.Citation18,27) The Fus3/Kss1 ortholog Chk1 in C. heterostrophus is involved not only in virulence but also in responses against oxidative and heavy-metal stresses.Citation20) However, the responses of the A. nidulans mpkB∆ strain to oxidative and heavy-metal stress were similar to those of the wild-type strain (Fig. S2). In A. nidulans,Citation14) Neurospora crassa,Citation28) and C. heterostrophus,Citation20) losing the function of a Fus3/Kss1 ortholog caused defects in the formation of sexual organs. Therefore, whereas some functions of Fus3/Kss1 orthologs differ among filamentous fungi, others partly overlap.
We previously demonstrated that MpkA regulates the transcription of the agsA and agsB genes but not most other cell wall-related genes.Citation6) In the present study, the transcript levels of the fksA, gelA, and gfaA genes were slightly increased in A. nidulans mpkB∆ compared with those in the wild-type strain. However, no noteworthy differences between the wild-type and mpkB∆ strains were apparent in the β-1,3-glucan and chitin contents of mycelia under normal growth conditions (Fig. ). Apparently, the slightly increased transcription (<2-fold) of fksA, gelA, and gfaA did not affect the composition of the cell wall in the mpkB∆ strain. In addition, the levels of fksA, gelA, and gfaA transcripts in the mpkB∆ strain recovered after 120 min of micafungin treatment comparable to their basal levels in the wild-type strain (Fig. ). Therefore, MpkB seems not to be directly involved in the transcriptional regulation of fksA, gelA, and gfaA. In response to micafungin, the transcription patterns of most cell wall-related genes were similar between the wild-type and mpkB∆ strains. Transcription of the genes tended to be up-regulated at 30–60 min of micafungin treatment and had returned to baseline at 120 min of micafungin treatment. Except for those of gelB, chsD, and csmA, these patterns are consistent with our previous observations.Citation6)
Transcription of gelB, chsD, and csmA was transiently up-regulated after micafungin treatment in the A89 strain we used previously.Citation6) In contrast, micafungin treatment did not alter chsD and csmA transcript levels markedly in the ABPU1 strain used in the present study, whereas the gelB transcript level continued to increase until at least the 120-min time point (Fig. ). The minor differences in transcript levels of these genes between the previous and present studies may reflect differences in the parental strains used. Despite these differences, the transcript levels of most cell wall-related genes did not differ between the mpkB∆ and wild-type strains under normal growth conditions in the present study. In addition, the phenotype of the mpkB∆, mpkA∆, and CmpkB-mpkA∆ strains yielded no evidence of crosstalk between the MpkA and MpkB pathways. Although the reasons for the micafungin sensitivities of the mpkA∆ and mpkB∆ strains remain unclear, the micafungin sensitivity of the CmpkB-mpkA∆ strain suggests that the inhibitory effects due to the transcriptional down-regulation of mpkB and mpkA are additive and depend on processes that are independent of each other.
Biochemical analysis of cell wall components revealed no noteworthy differences between the wild-type and mpkB∆ strains (Fig. ). This result suggests that MpkB pathway is not involved directly in CWIS under normal growth conditions. In contrast, our analysis of the transcriptional responses of the cell wall-related genes disclosed differences in agsA transcript levels in the mpkB∆ strain compared with the wild-type strain on exposure to micafungin. However, no noteworthy differences between the wild-type and mpkB∆ strains were noted in α-1,3-glucan content of mycelia after treatment with micafungin (data not shown). The overall levels of agsA transcription in both the wild-type and mpkB∆ strains were very low (Fig. ). These results suggest that the low level of agsA gene expression did not affect the composition of the cell wall in the mpkB∆ strain.
In addition, cell wall composition did not differ notably between the wild-type and mpkA∆ strains (Fig. ). This result was inconsistent with our previous observations. The transcription of A. nidulans agsB, the major α-1,3-glucan synthase gene,Citation24) is fully dependent on the MpkA pathway.Citation6) Transcription of agsB was maintained at a low level in the previous mpkA∆ strain, which was derived from A89 strain.Citation6) We therefore analyzed the transcript levels of agsB gene in the newly created mpkA∆ strain, which was derived from the ABPU1 strain. Although the level of transcription of the agsB gene was reduced by up to 50% in this strain compared with the parental ABPU1 strain, agsB gene expression was not fully dependent on the MpkA pathway in this strain (data not shown). The apparent differences in the dependence of agsB transcription on the MpkA pathway reflect differences between the parental strains. In addition, the HOG pathway may be involved in the regulation of cell wall-related genes, including chitin and β-1,3-glucan synthase, in Trichoderma reesei.Citation29) However, the detailed mechanism for the maintenance of the cell wall and the transcription factors related to cell wall biogenesis remain unclear in filamentous fungi. Additional studies, including analysis of the crosstalk among the three MPAK pathways, are needed to elucidate the mechanism underlying cell wall biogenesis in filamentous fungi.
Supplemental material
The supplemental material for this paper is available at http://dx.doi.org/10.1080/09168451.2014.998619.
Supplemental Materials
Download MS Power Point (1.4 MB)References
- Levin DE. Cell wall integrity signaling in Saccharomyces cerevisiae. Microbiol. Mol. Biol. Rev. 2005;69:262–291.10.1128/MMBR.69.2.262-291.2005
- Levin DE. Regulation of cell wall biogenesis in Saccharomyces cerevisiae: the cell wall integrity signaling pathway. Genetics. 2011;189:1145–1175.10.1534/genetics.111.128264
- Levin DE, Fields FO, Kunisawa R, Bishop JM, Thorner J. A candidate protein kinase C gene, PKC1, is required for the S. cerevisiae cell cycle. Cell. 1990;62:213–224.10.1016/0092-8674(90)90360-Q
- Jung US, Levin DE. Genome-wide analysis of gene expression regulated by the yeast cell wall integrity signalling pathway. Mol. Microbiol. 1999;34:1049–1057.10.1046/j.1365-2958.1999.01667.x
- Bussink HJ, Osmani SA. A mitogen-activated protein kinase (MPKA) is involved in polarized growth in the filamentous fungus, Aspergillus nidulans. FEMS Microbiol. Lett. 1999;173:117–125.10.1016/S0378-1097(99)00060-9
- Fujioka T, Mizutani O, Furukawa K, Sato N, Yoshimi A, Yamagata Y, Nakajima T, Abe K. MpkA-dependent and -independent cell wall integrity signaling in Aspergillus nidulans. Eukaryot. Cell. 2007;6:1497–1510.10.1128/EC.00281-06
- Fujikawa T, Kuga Y, Yano S, Yoshimi A, Tachiki T, Abe K, Nishimura M. Dynamics of cell wall components of Magnaporthe grisea during infectious structure development. Mol. Microbiol. 2009;73:553–570.10.1111/mmi.2009.73.issue-4
- Damveld RA, Arentshorst M, Franken A, VanKuyk PA, Klis FM, Van Den Hondel CAMJJ, Ram AFJ. The Aspergillus niger MADS-box transcription factor RlmA is required for cell wall reinforcement in response to cell wall stress. Mol. Microbiol. 2005;58:305–319.10.1111/j.1365-2958.2005.04827.x
- Valiante V, Heinekamp T, Jain R, Härtl A, Brakhage AA. The mitogen-activated protein kinase MpkA of Aspergillus fumigatus regulates cell wall signaling and oxidative stress response. Fung. Genet. Biol. 2008;45:618–627.10.1016/j.fgb.2007.09.006
- Jain R, Valiante V, Remme N, Docimo T, Heinekamp T, Hertweck C, Gershenzon J, Haas H, Brakhage AA. The MAP kinase MpkA controls cell wall integrity, oxidative stress response, gliotoxin production and iron adaptation in Aspergillus fumigatus. Mol. Microbiol. 2011;82:39–53.10.1111/mmi.2011.82.issue-1
- Kovács Z, Szarka M, Kovács S, Boczonádi I, Emri T, Abe K, Pócsi I, Pusztahelyi T. Effect of cell wall integrity stress and RlmA transcription factor on asexual development and autolysis in Aspergillus nidulans. Fung. Genet. Biol. 2013;54:1–14.10.1016/j.fgb.2013.02.004
- Furukawa K, Hoshi Y, Maeda T, Nakajima T, Abe K. Aspergillus nidulans HOG pathway is activated only by two-component signaling pathway in response to osmotic stress. Mol. Microbiol. 2005;56:1246–1261.10.1111/j.1365-2958.2005.04605.x
- Paoletti M, Seymour FA, Alcocer MJC, Kaur N, Calvo AM, Archer DB, Dyer PS. Mating type and the genetic basis of self-fertility in the model fungus Aspergillus nidulans. Curr. Biol. 2007;17:1384–1389.10.1016/j.cub.2007.07.012
- Atoui A, Bao D, Kaur N, Grayburn WS, Calvo AM. Aspergillus nidulans natural product biosynthesis is regulated by MpkB, a putative pheromon response mitogen-activated protein kinase. Appl. Environ. Microbiol. 2008;74:3596–3600.10.1128/AEM.02842-07
- Jun S-C, Lee S-J, Park H-J, Kang J-Y, Leem Y-E, Yang T-H, Chang M-H, Kim J-M, Jang S-H, Kim H-G, Han D-M, Chae K-S, Jahng K-Y. The MpkB MAP kinase plays a role in post-karyogamy processes as well as in hyphal anastomosis during sexual development in Aspergillus nidulans. J. Microbiol. 2011;49:418–430.10.1007/s12275-011-0193-3
- Lev S, Sharon A, Hadar R, Ma H, Horwitz BA. A mitogen activated protein kinase of the corn-leaf pathogen Cochliobolus heterostrophus is involved in conidiation, appressorium formation, and pathogenicity: diverse roles for mitogen-activated protein kinase homologs in foliar pathogens. Proc. Nat. Acad. Sci. USA. 1999;96:13542–13547.10.1073/pnas.96.23.13542
- Kim YK, Kawano T, Li DX, Kolattukudy PE. A mitogen-activated protein kinase kinase required for induction of cytokinesis and appressorium formation by host signals in the conidia of Colletotrichum gloeosporioides. Plant Cell. 2000;12:1331–1344.10.1105/tpc.12.8.1331
- Takano Y, Kikuchi T, Kubo Y, Hamer JE, Mise K, Furusawa I. The Colletotrichum lagenarium MAP kinase gene CMK1 regulates diverse aspects of fungal pathogenesis. Mol. Plant Microb. Interact. 2000;13:374–383.10.1094/MPMI.2000.13.4.374
- Ruiz-Roldán MC, Maier FJ, Schäfer W. PTK1, a mitogen-activated-protein kinase gene, is required for conidiation, appressorium formation, and pathogenicity of Pyrenophora teres on barley. Mol. Plant Microb. Interact. 2001;14:116–125.10.1094/MPMI.2001.14.2.116
- Izumitsu K, Yoshimi A, Kubo D, Morita A, Saitoh Y, Tanaka C. The MAPKK kinase ChSte11 regulates sexual/asexual development, melanization, pathogenicity, and adaptation to oxidative stress in Cochliobolus heterostrophus. Curr. Genet. 2009;55:439–448.10.1007/s00294-009-0257-7
- Kobayashi T, Abe K, Asai K, Gomi K, Juvvadi PR, Kato M, Kitamoto K, Takeuchi M, Machida M. Genomics of Aspergillus oryzae. Biosci. Biotechnol. Biochem. 2007;71:646–670.10.1271/bbb.60550
- Ichinomiya M, Uchida H, Koshi Y, Ohta A, Horiuchi H. A protein kinase C-encoding gene, pkcA, is essential to the viability of the filamentous fungus Aspergillus nidulans. Biosci. Biotechnol. Biochem. 2007;71:2787–2799.10.1271/bbb.70409
- Hagiwara D, Kondo A, Fujioka T, Abe K. Functional analysis of C2H2 zinc finger transcription factor CrzA involved in calcium signaling in Aspergillus nidulans. Curr. Genet. 2008;54:325–338.10.1007/s00294-008-0220-z
- Yoshimi A, Sano M, Inaba A, Kokubun Y, Fujioka T, Mizutani O, Hagiwara D, Fujikawa T, Nishimura M, Yano S, Kasahara S, Shimizu K, Yamaguchi M, Kawakami K, Abe K. Functional analysis of the α-1,3-glucan synthase genes agsA and agsB in Aspergillus nidulans: AgsB is the major α-1,3-glucan synthase in this fungus. PLoS ONE. 2013;8:e54893.10.1371/journal.pone.0054893
- Gwynne DI, Buxton FP, Sibley S, Davies RW, Lockington RA, Scazzocchio C, Sealy-Lewis HM. Comparison of the cis-acting control regions of two coordinately controlled genes involved in ethanol utilization in Aspergillus nidulans. Gene. 1987;51:205–216.10.1016/0378-1119(87)90309-X
- Wei H, Requena N, Fischer R. The MAPKK kinase SteC regulates conidiophore morphology and is essential for heterokaryon formation and sexual development in the homothallic fungus Aspergillus nidulans. Mol. Microbiol. 2003;47:1577–1588.10.1046/j.1365-2958.2003.03405.x
- Xu JR, Hamer JE. MAP kinase and cAMP signaling regulate infection structure formation and pathogenic growth in the rice blast fungus Magnaporthe grisea. Genes Dev. 1996;10:2696–2706.10.1101/gad.10.21.2696
- Li D, Bobrowicz P, Wilkinson HH, Ebbole DJ. A mitogen-activated protein kinase pathway essential for mating and contributing to vegetative growth in Neurospora crassa. Genetics. 2005;170:1091–1104.10.1534/genetics.104.036772
- Wang M, Zhao Q, Yang J, Jiang B, Wang F, Liu K, Fang X. A mitogen-activated protein kinase Tmk3 participates in high osmolarity resistance, cell wall integrity maintenance and cellulase production regulation in Trichoderma reesei. PLoS ONE. 2013;8:e72189.10.1371/journal.pone.0072189