Abstract
In this study, the antidiabetic effects of a hot water extract of edible Chrysanthemum morifolium Ramat. (HW-ECM) were investigated in type 2 diabetic mice. HW-ECM improved blood glucose levels and insulin resistance and increased adiponectin mRNA expression in adipose tissues and protein concentrations in the plasma. Moreover, it increased adipose mRNA and protein expressions of peroxisome proliferator-activated receptor γ (PPARγ), a regulator of adiponectin transcription, and mRNA expression of its downstream target genes. It also reduced the adipose cell size and attenuated the mRNA expression of pro-inflammatory adipocytokines in adipose tissues. These data presumably indicate a hypoglycemic mechanism of HW-ECM, involving increased PPARγ expression, decreased the adipocyte sizes, and suppression of chronic inflammation in adipose tissues. Finally, elevated adiponectin levels lead to amelioration of insulin resistance and the corresponding hypoglycemic effects. Therefore, HW-ECM indicates its potential as a functional food for type 2 diabetes.
The possible mechanism of the antidiabetic effects of HW-ECM in obese diabetic KK-Ay mice.

Diabetes mellitus is a serious and increasing public health concern globally. In particular, type 2 diabetes is caused by insulin resistance of target tissues and will likely affect more than 366 million people by the year 2030.Citation1) It has been demonstrated that insulin sensitivity is inversely correlated with adipocyte size, and its sensitivity to insulin decreases with increasing the adipocyte size.Citation2) Peroxisome proliferator-activated receptor γ (PPARγ) is a transcription factor that plays a central role in adipocyte differentiation, and its activation leads to miniaturization of adipocytes.Citation2,3) Moreover, PPARγ regulates the transcription of the adipocytokine adiponectin,Citation4) which attenuates whole-body insulin resistance.Citation3) Secretion of adiponectin from adipocytes is inversely correlated with adipocyte cell size.Citation5,6) In addition, plasma adiponectin levels are lower in patients with type 2 diabetes than in healthy subjects, and low adiponectin concentrations are a strong independent predictor of future type 2 diabetes.Citation7) Accordingly, increased adiponectin secretion and miniaturization of adipocytes improve type 2 diabetes by attenuating insulin resistance.
Recent studies indicated that whole-body insulin resistance is caused by obesity-induced chronic inflammation in adipose tissues.Citation8) Chronic inflammation is characterized by upregulation of pro-inflammatory adipocytokines such as monocyte chemoattractant protein-1 (MCP-1), which induces macrophage infiltration into adipose tissues, and tumor necrosis factor α (TNF-α), which cause insulin resistance.Citation9,10) TNF-α also inhibits the expression of adiponectin.Citation10−12) Thus, the suppression of chronic inflammation contributes to improvements in type 2 diabetes by ameliorating insulin resistance in adipose tissues.
Various drugs have been developed to modulate adipose tissue function and to improve insulin sensitivity and glucose homeostasis. Among these, thiazolidinediones (TZD) are a principal class of drugs that improve insulin sensitivity in obese diabetic subjects and act by promoting adipocyte differentiation and insulin sensitivity and by suppressing adipose tissue inflammation through the activation of PPARγ.Citation13,14) However, TZD therapy reportedly causes several side effects.Citation15,16) Accordingly, numerous recent studies have focused on dietary constituents that may be beneficial for the prevention and treatment of diabetes.
The chrysanthemum (Chrysanthemum morifolium Ramat.) plant, which has been bred for more than 2,000 years, is one of the most important ornamental flowers in the world. In Japan, C. morifolium Ramat. has been widely cultivated in the northeastern part of the Honshu Island as a traditional edible flower, with various advantageous biological properties. For example, C. morifolium Ramat. showed anti-inflammatory activity in ear edema, and triterpene was reportedly the key functional component.Citation20) The C. morifolium Ramat. constituent acacetin-7-o-β-d-galactopyranoside also has anti-HIV activity.Citation21) However, few studies have assessed the effects of edible C. morifolium Ramat. (ECM) on type 2 diabetes mellitus.
In this study, we investigated the antidiabetic effects of HW-ECM on type 2 diabetic mice. The present data indicate hypoglycemic activity, reduced obesity-related insulin resistance, and enhanced adiponectin production in HW-ECM-fed mice.
Materials and methods
Preparation of HW-ECM. Edible C. morifolium Ramat
‘‘Kotobuki’’ was purchased from JA Yamagata (Yamagata, Japan). ECM (10 kg) was homogenized in 10 volumes of deionized water and then boiled at 90 °C for 15 min. The extract was then filtered through ADVANTEC No. 2 filter paper (Toyo Roshi Kaisha, Ltd., Tokyo, Japan) before lyophilizing with a freeze dryer (LABCONCO FZ-12, Kansas City, MO). About 400 g of the HW-ECM was collected and stored at −20 °C until further use.
Animals and diets
Nine-week-old male KK-Ay mice (CLEA Japan, Inc., Tokyo, Japan) were individually housed in stainless steel cages in an animal room at 23–25 °C and 50–56% humidity under a 12 h light/12 h dark cycle (lights on 8:00–20:00) with ad libitum access to food and drinking water. After acclimatization to a normal AIN-93G-based dietCitation22) for one week, animals were divided into three groups of 10 individuals. The control (Con) and HW-ECM groups were pair-fed an AIN-93 M normal diet and an AIN-93 M diet containing 1 or 5% HW-ECM, respectively, for five weeks. Diets for the HW-ECM groups were prepared by replacing cornstarch and cellulose with HW-ECM to maintain dietary fiber contents (Table ).
Table 1. Composition of experimental diets.
Prior to weekly blood glucose analysis, mice were fasted for 2 h and blood was collected from the tail vein. Blood glucose levels were determined using an automatic blood glucose meter (LIFE CHECK, EIDIA Co., Ltd., Tokyo, Japan). Following the feeding period and an 8 h fasting period, animals were dissected under anesthesia with sodium pentobarbital. Plasma was collected and glucose, insulin, adiponectin, aspartate aminotransferase (AST), and alanine aminotransferase (ALT) levels were determined. Epididymal white adipose tissues were collected for mRNA expression and histochemical analysis. Animal experiments were performed in accordance with the guidelines for maintenance and handling of experimental animals established by the Tokyo University of Agriculture Ethics Committee.
Insulin tolerance tests (ITTs)
ITTs were performed at four weeks after the start of feeding according to the method of Sasaki et al.Citation23) Mice were fasted for 14 h and then received intraperitoneal injections of 0.67 U/kg insulin (Humulin R, Eli Lilly and Company, Indianapolis, IN). Blood samples were collected from the tail vein at 0, 30, 60, 90, 120, and 150 min after insulin injection. Blood glucose levels were determined as described above. The area under the curve (AUC) was calculated from glucose levels.
Blood analysis
Plasma glucose levels were measured using a Glucose CII-test (Wako Pure Chemical Industries Ltd., Osaka, Japan). Plasma insulin levels were measured using a Mouse Insulin ELISA kit (AKRIN-011T, Shibayagi Co. Ltd., Gunma, Japan). Plasma glucose and insulin levels were used to calculate the homeostasis model assessment of insulin resistance index (HOMA-IR), an indicator of systemic insulin resistance, using the following formula: HOMA-IR = fasting blood glucose (mg/dL) × fasting blood insulin (μU/mL)/405. Plasma adiponectin levels were measured using a mouse/rat adiponectin ELISA kit (Otsuka Pharmaceutical Co., Ltd., Tokyo, Japan) according to the manufacturer’s instructions. Plasma AST and ALT levels were measured using a Transaminase CII-test (Wako Pure Chemical Industries Ltd.).
Histochemical analysis
Epididymal white adipose tissue was fixed in 10% formaldehyde. Fixed samples were then embedded in paraffin wax and cut into 3 μm thick sections. Sections were mounted on glass slides and stained with hematoxylin and eosin. To determine the average cell size, number of adipocytes, and size distributions of adipocytes, adipocyte diameters were measured in three fields on slides from each mouse using an image analysis software (ImageJ vs. 1.42, National Institutes of Health, Bethesda, MD).
Real-time polymerase chain reaction
Total RNA was isolated from epididymal white adipose tissue specimens using the Isogene II reagent (NIPPON GENE Co., Ltd., Tokyo, Japan) according to the manufacturer’s instructions. After treatment with DNase (Promega KK, Tokyo, Japan), total RNA (1.0 μg) was reverse transcribed to cDNA in a final reaction volume of 20 μL using a High Capacity cDNA Reverse Transcription Kit (Thermo Fisher Scientific Inc., Waltham, MA). Amplification was performed in a final volume of 25 μL containing cDNA, optimized specific primers, and probes (TaqMan Gene Expression Assays, Thermo Fisher Scientific Inc.) using a real-time PCR system (Applied Biosystems 7300, Thermo Fisher Scientific Inc.). The assay IDs of the targets were as follows: β-actin, NM_007393.1; PPARγ, Mm01184322_m1; adiponectin, Mm00456425_m1; adipocyte fatty acid-binding protein 4 (aP2), Mm00445878_m1; glucose transporter type 4 (GLUT4), Mm01245502_m1; lipoprotein lipase (LPL), Mm00434764_m1; TNF-α, Mm00443260_m1; MCP-1, Mm00441242_m1; F4/80, Mm00802529. Data were expressed as fold-increases relative to the control after normalization to β-actin mRNA levels.
Immunoblot analysis
Protein in epididymal white adipose tissues was extracted with an RIPA buffer and quantified using an RC DC protein assay reagent (Bio-Rad Laboratories, Inc., Hercules, CA). Equal amounts of protein lysates were loaded and electrophoresed on an 8% (w/v) Tris–glycine polyacrylamide gel in sodium dodecyl sulfate. Following electrophoresis, the proteins were transferred onto a polyvinylidene difluoride (PVDF) membrane using a transfer buffer. The membrane was subsequently blocked with PVDF Blocking Reagent for Can Get Signal® (TOYOBO CO., Ltd., Osaka, Japan) for 1 h. After washing two times with Tris-buffered saline containing 0.5% (v/v) Tween 20 (TBST), the membrane was incubated with an anti-PPARγ antibody (Cell Signaling Technology, Inc., Danvers, MA) diluted 1,000-fold with Can Get Signal® (TOYOBO CO., Ltd.,) solution 1 at 4 °C overnight. After washing five times with TBST, the membrane was incubated with an anti-rabbit IgG-horseradish peroxidase (Cell Signaling Technology, Inc.) diluted 2,500-fold with Can Get Signal® Solution 2 for 1 h. After washing 5 times with TBST, immunoreactive bands were visualized using an Amersham ECL Advance Western Blotting Detection Kit (GE Healthcare UK., Ltd., Buckingham, England).
Statistical analysis
Statistical analysis was performed using the SPSS 15.0J software. The significance of differences of the means among the groups was assessed by one-way analysis of variance (ANOVA), followed by Tukey’s multiple comparison tests. Data were expressed as means ± standard errors (SE).
Results
Effect of HW-ECM on body weight, food intake, fat mass, liver weight, and toxicological parameters
Table shows body weight, food intake, fat mass, liver weight, and plasma ALT and AST levels after feeding HW-ECM for five weeks. No significant changes in these parameters were observed between control and HW-ECM groups.
Table 2. Effect of HW-ECM on body weight, food intake, fat mass, liver weight, and toxicological parameters.
HW-ECM improved blood glucose level and ameliorated insulin resistance
Fig. (A) shows the time course of blood glucose levels of type 2 diabetic KK-Ay mice that were fed diets containing HW-ECM. HW-ECM supplementations reduced blood glucose levels in a dose-dependent manner. In comparison with the control group, 1% HW-ECM tended to decrease blood glucose levels during the feeding period, whereas 5% HW-ECM significantly decreased blood glucose levels at weeks 2, 3, and 5. In ITTs (Fig. (B)), blood glucose levels tended to reduce in the 1% HW-ECM group and were significantly reduced in the 5% HW-ECM group at 30 min after insulin injections. At 60 min after the insulin treatment, both HW-ECM groups showed significantly lower glucose levels compared with those in the control group. The glucose-lowering effect of the 5% HW-ECM group was significantly greater than that of the 1% HW-ECM group. In addition, dietary HW-ECM reduced AUC in a dose-dependent manner with a significant lower AUC in the 5% HW-ECM group than in the control group (Fig. (C)). The HW-ECM intake also reduced HOMA-IR in a dose-dependent manner (Fig. (D)), with significant decrease in the 5% HW-ECM group.
Fig. 1. Effect of HW-ECM on blood glucose levels and insulin resistance in KK-Ay mice.
Notes: (A) Blood glucose levels during the feeding period; (B) insulin tolerance tests (ITTs); (C) area under the curve (AUC) in ITTs; and (D) homeostasis model analysis of insulin resistance (HOMA-IR) in HW-ECM-fed KK-Ay mice. Blood was collected from the tail vein every week, and blood glucose levels were determined using an automatic blood glucose meter. ITTs were performed at four weeks after the feeding period, and blood samples were collected from tail tips at 0, 30, 60, 90, 120, and 150 min after insulin injections. AUC was calculated from glucose levels during ITTs. HOMA-IR was calculated from fasting plasma glucose levels and fasting plasma insulin levels. Bars and values indicate means ± SE (n = 10). Bars and values without common letters indicate significant differences (p < 0.05).
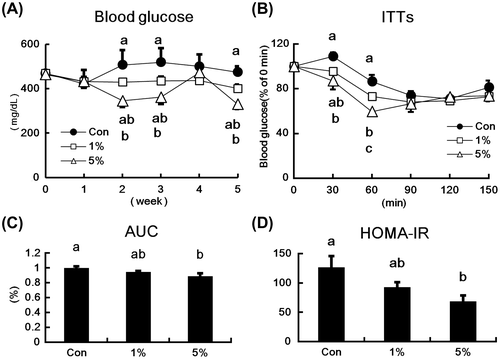
Effects of HW-ECM on the levels of plasma adiponectin and adiponectin mRNA in epididymal white adipose tissues
Plasma adiponectin levels and adiponectin mRNA expression in the adipose tissues of HW-ECM-fed mice are shown in Fig. . Plasma adiponectin concentrations (Fig. (A)) tended to increase in the 1% HW-ECM group, and they were significantly higher in the 5% HW-ECM group compared with control mice. Similarly, adiponectin mRNA expression in adipose tissues tended to increase in the 1% HW-ECM group and was significantly induced in the 5% HW-ECM group (Fig. (B)).
Fig. 2. Effects of HW-ECM on plasma concentrations and mRNA level of adiponectin in adipose tissues.
Notes: (A) Plasma adiponectin protein levels were determined using an enzyme-linked immunosorbent assay (ELISA) and (B) mRNA expression in epididymal white adipose tissues was measured using real-time PCR and expressed relative to that of β-actin. Bars indicate the mean ± SE (n = 10). Bars without common letters represent significant differences (p < 0.05).
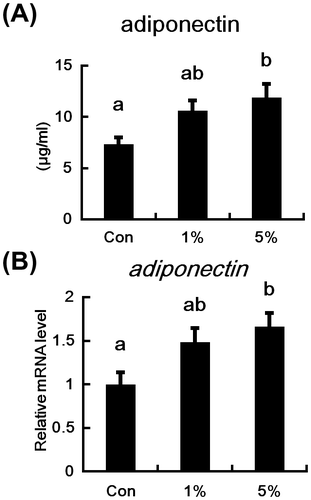
Effects of HW-ECM on mRNA and protein expressions of PPARγ and mRNA expression of downstream target genes of PPARγ in epididymal white adipose tissue
The effects of dietary HW-ECM on the expressions of PPARγ, aP2, GLUT4, and LPL mRNA in epididymal white adipose tissues are shown in Fig. . PPARγ mRNA levels tended to increase in the 1% HW-ECM group and were significantly induced in the 5% HW-ECM group (Fig. (A)). The protein level of PPARγ was also increased in the HW-ECM groups, in particular in the 5% HW-ECM group (Fig. (B)). Additionally, the PPARγ downstream target genes aP2, GLUT4, and LPL were significantly induced in both the HW-ECM groups (Fig. (C)–(E)).
Fig. 3. Effects of HW-ECM on the mRNA and protein expressions of PPARγ and mRNA expression of its downstream target genes in epididymal white adipose tissues.
Notes: The mRNA levels (A) PPARγ; (C) aP2; (D) GLUT4; and (E) LPL in epididymal white adipose tissues were determined using real-time PCR and are expressed relative to those of β-actin. The protein levels of PPARγ (B) in epididymal white adipose tissues were evaluated using an immunoblot analysis. The representative data from each group are shown. Bars indicate the mean ± SE (n = 10). Bars without common letters represent significant differences (p < 0.05).
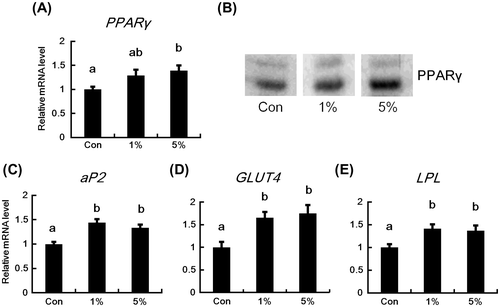
HW-ECM reduced the adipocyte size and changed the size distribution of adipocytes
The adipocyte size in epididymal adipose tissues of mice fed HW-ECM were assessed using histochemical analyses (Fig. ), which showed reductions in both the HW-ECM groups (Fig. (A)). The average adipocyte cell size of the HW-ECM groups was significantly smaller than those of control mice (Fig. (B)). The average number of adipocytes in three fields on slides from each mouse was also significantly increased in both the HW-ECM groups (273.9 ± 12.7 (Con), 302.6 ± 10.6 (1%), 321.5 ± 16.8 (5%) cells). Moreover, in size distributions of adipocytes (Fig. (C)), HW-ECM feeding dose-dependently decreased the proportion of large adipocytes (10,000–20,000 μm2) and increased the proportion of adipocytes <6,000 μm2 compared with those of control mice. In particular, the ratio of adipocytes <4,000 μm2 was more than two times higher in the 5% HW-ECM group than in the control group.
Fig. 4. Effect of HW-ECM on adipocyte size in epididymal white adipose tissues.
Notes: (A) Histological analyses of epididymal white adipose tissues of KK-Ay mice; (B) the average cell size of adipocytes; (C) adipocyte size distributions. Tissues were fixed in 10% formaldehyde before cutting into 3-μm thick sections. Sections were then mounted on glass slides and stained with hematoxylin and eosin. Scale bars indicate 200 μm. To determine the average cell size and size distributions of adipocytes, the diameter of adipocytes in three fields of slides from each mouse was measured using the ImageJ software. Bars indicate the mean ± SE (n = 10). Bars without common letters represent significant differences (p < 0.05).
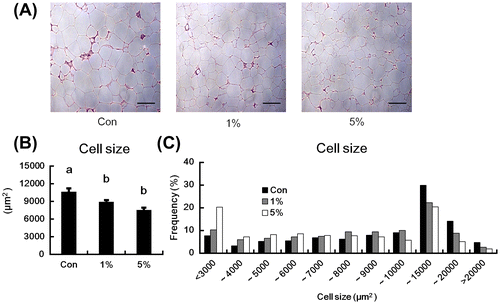
Effect of HW-ECM on mRNA expression of inflammatory genes in epididymal white adipose tissues
Relative mRNA levels of the pro-inflammatory adipocytokines MCP-1 and TNF-α were significantly lower in the HW-ECM groups than in control mice (Fig. (A) and (B)). In addition, the expression of the macrophage marker F4/80 was significantly reduced in both the HW-ECM groups (Fig. (C)).
Fig. 5. Effects of HW-ECM on the mRNA expressions of genes related to chronic inflammation in epididymal white adipose tissues.
Notes: The mRNA levels of (A) MCP-1; (B) TNF-α; and (C) F4/80 in epididymal white adipose tissues were measured using real-time PCR. Target mRNA levels are expressed relative to those of β-actin. Bars indicate the mean ± SE (n = 10). Bars without common letters represent significant differences (p < 0.05).
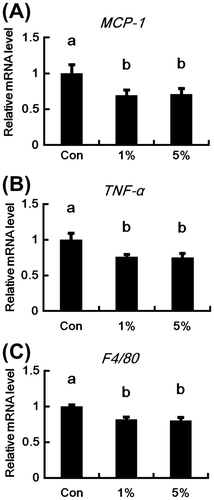
Discussion
Type 2 diabetes results from insulin resistance, which is characterized by the ineffective utilization of insulin in the body. Thus, the improvement of insulin resistance is an important target of treatments for type 2 diabetes. Although several studies have demonstrated advantageous biological effects of ECM,Citation17−19) few have examined these effects in the context of type 2 diabetes. Thus, to investigate the antidiabetic potential, we examined the effects of dietary HW-ECM in obese diabetic KK-Ay mice.
In initial experiments, 1 and 5% HW-ECM had no effects on body weights, liver mass, or plasma AST and ALT levels of mice (Table ), indicating negligible HW-ECM toxicity at the present concentrations.
In further experiments, HW-ECM treatments dose-dependently decreased blood glucose levels after week 2 but did not at week 4 (Fig. (A)). In agreement, ITTs and HOMA-IR analyses, which indicate insulin efficacy,Citation24) clearly showed improved insulin resistance in type 2 diabetic mice that were fed HW-ECM (Fig. (B)–(D)), suggesting that HW-ECM has antidiabetic potential.
Subsequent experiments were designed to determine the effects of HW-ECM supplementation on plasma adiponectin levels and adipose adiponectin mRNA expression. Adiponectin is an adipocytokine derived from adipocytes, and it has been shown to attenuate whole-body insulin resistance.Citation25) Moreover, plasma adiponectin levels are lower in patients with type 2 diabetes than in healthy subjects.Citation7) Therefore, increased adiponectin levels are associated with improvements of type 2 diabetes. In this study, HW-ECM treatments, in particular the 5% HW-ECM treatment, elevated plasma concentrations of adiponectin in accordance with increased mRNA expression in adipose tissues (Fig. (A) and (B)). Thus, HW-ECM improves insulin resistance by inducing adiponectin.
Expression studies on PPARγ and its downstream target genes, and histochemical analyses of the adipocyte size confirmed the benefits of dietary HW-ECM. Specifically, mRNA and protein expressions of PPARγ and mRNA expression of its downstream targets aP2, GLUT4, and LPL were significantly enhanced in the HW-ECM-fed mice (Fig. (A)–(E)). PPARγ is the master regulator of adipocyte differentiation, the target of insulin-sensitizing drugs, and the regulator of adiponectin transcription.Citation3,4,13) Thus, the present data suggest that HW-ECM increases plasma concentrations and adipose mRNA levels of adiponectin by upregulating PPARγ in adipose tissues. Moreover, HW-ECM treatments significantly reduced the size of adipocytes (Fig. (A) and (B)), decreased the proportion of large adipocytes, and increased the proportion of small adipocytes (Fig. (C)). Additionally, adiponectin secretion from adipocytes is inversely related to adipocyte size.Citation5) Thus, HW-ECM-induced PPARγ expression in adipose tissues stimulates the production of adiponectin by increasing the transcription of adiponectin and miniaturizing adipocytes. Recent studies have identified natural compounds that modulate PPAR, including polyphenols such as naringenin, kaempferol, and genistein, which activate PPARγ.Citation26) Accordingly, polyphenols are potential as therapeutic agents for diabetes mellitus. Because HW-ECM was prepared using hot water extraction, the present extracts likely contained polyphenols and corresponding glycosides that may be associated with increased PPARγ expression. However, further studies are warranted to elucidate the mechanisms by which HW-ECM increases PPARγ expression.
Interestingly, HW-ECM miniaturizes adipocytes (Fig. ), but not changes the weights of white adipose tissues (Table ). We have previously reported that HW-ECM promoted adipocyte differentiation in 3T3-L1 cells.Citation27) In this study, the HW-ECM feeding enhanced the expression of PPARγ and its downstream targets, decreased the proportion of large adipocytes, and increased the proportion of small adipocytes. Similarly, insulin-sensitizing drug TZD, a PPARγ agonist, increases the number of newly differentiated small adipocytes by enhancing adipocyte differentiation and decreases the number of large adipocytes by inducing apoptosis, without changing fat mass.Citation2,6,28) HW-ECM might decrease the size of adipocytes without altering fat weights by promoting adipocyte differentiation and inducing apoptosis.
Obesity aggravates insulin resistance by contributing to macrophage-induced chronic inflammation in adipose tissues.Citation29,30) MCP-1 plays a crucial role in inflammatory responses of adipose tissues by inducing the infiltration of macrophages into adipose tissues.Citation9) Subsequently, a disadvantageous cycle is established in adipocytes, involving a paracrine loop of TNF-α and free fatty acids, the augmentation of inflammatory changes and insulin resistance, and the suppression of adiponectin expression in adipose tissues.Citation10,29,31) Thus, the suppression of obesity-related inflammation by decreasing the expression of MCP-1 in adipose tissues may ameliorate insulin resistance. In this study, HW-ECM treatments significantly decreased MCP-1, TNF-α, and F4/80 (Fig. (A)–(C)) mRNA expressions in adipose tissues. These data suggest that HW-ECM suppresses chronic inflammation in adipose tissues of obese diabetic KK-Ay mice. Because MCP-1 expression was enhanced in hypertrophied adipocytes,Citation32) miniaturization of adipocytes following supplementation with HW-ECM (Fig. (A)–(C)) may decrease MCP-1 expression. Furthermore, reductions in MCP-1 expression likely inhibit macrophage infiltration into adipose tissues and may decrease TNF-α and F4/80 mRNA expressions in adipose tissues. Taken together, these data suggest that HW-ECM improves chronic inflammation in adipose tissues by reducing MCP-1 expression through miniaturizing the size of adipocytes. In addition, the improved inflammation partly leads to the amelioration of insulin resistance and the increase in adiponectin because HW-ECM reduced the mRNA levels of TNF-α that inhibits insulin signaling and adiponectin expression.
Chrysanthemum flowers contain biologically active substances including various polyphenols that have well characterized effects.Citation18,33) The main polyphenols of chrysanthemum flowers are chlorogenic acid, luteolin, apigenin, and their glycosides.Citation34) Although these polyphenols have reported antidiabetic activities,Citation35−37) chlorogenic acid and its metabolite caffeic acid failed to promote adipocyte differentiation in a previous study.Citation38) Moreover, the antidiabetic effects of apigenin have not been assessed in type 2 diabetic models. Luteolin showed antidiabetic activity but it decreased body weight and fat mass in obese C57/BL6 mice fed a high-fat diet.Citation36) In contrast, although HW-ECM had antidiabetic effects in type 2 diabetic KK-Ay mice and enhanced adipocyte differentiation,Citation27) no reductions in body weight or fat mass were observed (Table ), suggesting the presence of additional antidiabetic HW-ECM constituents.
In conclusion, the present data demonstrate for the first time that HW-ECM ameliorates insulin resistance and has antidiabetic effects in type 2 diabetic KK-Ay mice. Type 2 diabetes is a chronic metabolic disorder of increasing global prevalence, and insulin resistance is central to the ensuing pathologies. Therefore, HW-ECM has potential as a functional food for the prevention and improvement of type 2 diabetes. The possible mechanism of the antidiabetic effects of HW-ECM is as follows: HW-ECM increases PPARγ expression in white adipose tissues, leading to the direct induction of adiponectin and miniaturization of adipocytes. The miniaturized adipocytes increase adiponectin production and inhibit MCP-1 production. The inhibited MCP-1 production improves chronic inflammation by suppressing macrophage infiltration into adipose tissues. The improvement of inflammation also contributes to the increased adiponectin production in adipose tissues. Consequently, the increased adiponectin production induces the amelioration of insulin resistance and subsequently the hypoglycemic effect of HW-ECM on type 2 diabetic status. However, HW-ECM is an extract and presumably contains anti-inflammatory substances such as chlorogenic acid and luteolin.Citation39,40) Thus, HW-ECM may directly suppress chronic inflammation without increasing PPARγ expression. However, further studies are warranted to elucidate the detailed hypoglycemic mechanisms of HW-ECM and to identify antidiabetic components.
Notes
Abbreviations: ALT, alanine aminotransferase; aP2, adipocyte fatty acid-binding protein 4; AST, aspartate aminotransferase; AUC, area under the curve; C/EBPα, CCAAT/enhancer-binding protein alpha; ECM, edible Chrysanthemum morifolium Ramat.; GLUT4, glucose transporter type 4; HW-ECM, hot water extract of edible Chrysanthemum morifolium Ramat.; ITTs, insulin tolerance tests; LPL, lipoprotein lipase; MCP-1, monocyte chemoattractant protein-1; PPAR γ, peroxisome proliferator-activated receptor γ; PVDF, polyvinylidene difluoride; TBST, Tris-buffered saline containing 0.5% (v/v) Tween 20; TG, triglyceride; TNF-α, tumor necrosis factor α; TZD, thiazolidinedione.
References
- Smyth S, Heron A. Diabetes and obesity: the twin epidemics. Nat. Med. 2006;12:75–80.10.1038/nm0106-75
- Okuno A, Tamemoto H, Tobe K, Ueki K, Mori Y, Iwamoto K, Umesono K, Akanuma Y, Fujiwara T, Horikoshi H, Yazaki Y, Kadowaki T. Troglitazone increases the number of small adipocytes without the change of white adipose tissue mass in obese Zucker rats. J. Clin. Invest. 1998;101:1354–1361.10.1172/JCI1235
- Rosen ED. The transcriptional basis of adipocyte development. Prostaglandins Leukot Essent. Fatty Acids 2005;73:31–34.10.1016/j.plefa.2005.04.004
- Iwaki M, Matsuda M, Maeda N, Funahashi T, Matsuzawa Y, Makishima M, Shimomura I. Induction of adiponectin, a fat-derived antidiabetic and antiatherogenic factor, by nuclear receptors. Diabetes. 2003;52:1655–1663.10.2337/diabetes.52.7.1655
- Kadowaki T. Insights into insulin resistance and type 2 diabetes from knockout mouse models. J. Clin. Invest. 2000;106:459–465.10.1172/JCI10830
- Yamauchi T, Kamon J, Waki H, Terauchi Y, Kubota N, Hara K, Mori Y, Ide T, Murakami K, Tsuboyama-Kasaoka N, Ezaki O, Akanuma Y, Gavrilova O, Vinson C, Reitman ML, Kagechika H, Shudo K, Yoda M, Nakano Y, Tobe K, Nagai R, Kimura S, Tomita M, Froguel P, Kadowaki T. The fat-derived hormone adiponectin reverses insulin resistance associated with both lipoatrophy and obesity. Nat. Med. 2001;7:941–946.10.1038/90984
- Spranger J, Kroke A, Möhlig M, Bergmann MM, Ristow M, Boeing H, Pfeiffer AFH. Adiponectin and protection against type 2 diabetes mellitus. Lancet. 2003;361:226–228.10.1016/S0140-6736(03)12255-6
- Suganami T, Nishida J, Ogawa Y. A paracrine loop between adipocytes and macrophages aggravates inflammatory changes: role of free fatty acids and tumor necrosis factor alpha. Arterioscler. Thromb. Vasc. Biol. 2005;25:2062–2068.10.1161/01.ATV.0000183883.72263.13
- Hirai S, Takahashi N, Goto T, Lin S, Uemura T, Yu R, Kawada T. Functional food targeting the regulation of obesity-induced inflammatory responses and pathologies. Mediators Inflamm. 2010;2010. Article ID 367838, 8 pages.
- Gustafson B. Adipose tissue, inflammation and atherosclerosis. J. Atheroscler. Thromb. 2010;17:332–341.10.5551/jat.3939
- Yamauchi T, Kadowaki T. Physiological and pathophysiological roles of adiponectin and adiponectin receptors in the integrated regulation of metabolic and cardiovascular diseases. Int. J. Obes. (Lond). 2008;32:S13–18.10.1038/ijo.2008.233
- Maeda N, Takahashi M, Funahashi T, Kihara S, Nishizawa H, Kishida K, Nagaretani H, Matsuda M, Komuro R, Ouchi N, Kuriyama H, Hotta K, Nakamura T, Shimomura I, Matsuzawa Y. PPARgamma ligands increase expression and plasma concentrations of adiponectin, an adipose-derived protein. Diabetes. 2001;50:2094–2099.10.2337/diabetes.50.9.2094
- Evans RM, Barish GD, Wang Y-X. PPARs and the complex journey to obesity. Nat. Med. 2004;10:355–361.10.1038/nm1025
- Bodles AM, Varma V, Yao-Borengasser A, Phanavanh B, Peterson CA, McGehee RE, Rasouli N, Wabitsch M, Kern PA. Pioglitazone induces apoptosis of macrophages in human adipose tissue. J. Lipid Res. 2006;47:2080–2088.10.1194/jlr.M600235-JLR200
- Scheen AJ. Thiazolidinediones and liver toxicity. Diabetes Metab. 2001;27:305–313.
- Shah P, Mudaliar S. Pioglitazone: side effect and safety profile. Expert Opin. Drug Saf. 2010;9:347–354.10.1517/14740331003623218
- Kim HJ, Lee YS. Identification of new dicaffeoylquinic acids from Chrysanthemum morifolium and their antioxidant activities. Planta Med. 2005;71:871–876.10.1055/s-2005-873115
- Ukiya M, Akihisa T, Yasukawa K, Kasahara Y, Kimura Y, Koike K, Nikaido T, Takido M. Constituents of compositae plants. 2. triterpene diols, triols, and their 3- o -fatty acid esters from edible chrysanthemum flower extract and their anti-inflammatory effects. J. Agric. Food. Chem. 2001;49:3187–3197.10.1021/jf010164e
- Miyazawa M, Hisama M. Antimutagenic activity of flavonoids from Chrysanthemum morifolium. Biosci. Biotechnol. Biochem. 2003;67:2091–2099.10.1271/bbb.67.2091
- Akihisa T, Yasukawa K, Oinuma H, Kasahara Y, Yamanouchi S, Takido M, Kumaki K, Tamura T. Triterpene alcohols from the flowers of compositae and their anti-inflammatory effects. Phytochemistry. 1996;43:1255–1260.10.1016/S0031-9422(96)00343-3
- Hu CQ, Chen K, Shi Q, Kilkuskie RE, Cheng YC, Lee KH. Anti-AIDS agents, 10. Acacetin-7-O-beta-D-galactopyranoside, an anti-HIV principle from Chrysanthemum morifolium and a structure-activity correlation with some related flavonoids. J. Nat. Prod. 1994;57:42–51.10.1021/np50103a006
- Reeves PG, Nielsen FH, Fahey GC. AIN-93 purified diets for laboratory rodents: final report of the American Institute of Nutrition ad hoc writing committee on the reformulation of the AIN-76A rodent diet. J. Nutr. 1993;123:1939–1951.
- Sasaki R, Nishimura N, Hoshino H, Isa Y, Kadowaki M, Ichi T, Tanaka A, Nishiumi S, Fukuda I, Ashida H, Horio F, Tsuda T. Cyanidin 3-glucoside ameliorates hyperglycemia and insulin sensitivity due to downregulation of retinol binding protein 4 expression in diabetic mice. Biochem. Pharmacol. 2007;74:1619–1627.10.1016/j.bcp.2007.08.008
- Matthews DR, Hosker JP, Rudenski AS, Naylor BA, Treacher DF, Turner RC. Homeostasis model assessment: insulin resistance and beta-cell function from fasting plasma glucose and insulin concentrations in man. Diabetologia. 1985;28:412–419.10.1007/BF00280883
- Kadowaki T, Yamauchi T. Adiponectin and adiponectin receptors. Endocr. Rev. 2005;26:439–451.10.1210/er.2005-0005
- Goto T, Kim Y-I, Takahashi N, Kawada T. Natural compounds regulate energy metabolism by the modulating the activity of lipid-sensing nuclear receptors. Mol. Nutr. Food Res. 2013;57:20–33.10.1002/mnfr.v57.1
- Yamamoto J, Yamane T, Oishi Y, Shimizu M, Tadaishi M, Kobayashi-Hattori K. Chrysanthemum extract promotes adipocyte differentiation, adiponectin secretion, and glucose uptake. Am. J. Chin. Med. Forthcoming.
- Yamauchi T, Kamon J, Waki H, Murakami K, Motojima K, Komeda K, Ide T, Kubota N, Terauchi Y, Tobe K, Miki H, Tsuchida A, Akanuma Y, Nagai R, Kimura S, Kadowaki T. The mechanisms by which both heterozygous peroxisome proliferator-activated receptor gamma (PPARgamma) deficiency and PPARgamma agonist improve insulin resistance. J. Biol. Chem. 2001;276:41245–41254.10.1074/jbc.M103241200
- Xu H, Barnes GT, Yang Q, Tan G, Yang D, Chou CJ, Sole J, Nichols A, Ross JS, Tartaglia LA, Chen H. Chronic inflammation in fat plays a crucial role in the development of obesity-related insulin resistance. J. Clin. Invest. 2003;112:1821–1830.10.1172/JCI200319451
- Weisberg SP, McCann D, Desai M, Rosenbaum M, Leibel RL, Ferrante AW. Obesity is associated with macrophage accumulation in adipose tissue. J. Clin. Invest. 2003;112:1796–1808.10.1172/JCI200319246
- Kawada T, Goto T, Hirai S, Kang M-S, Uemura T, Yu R, Takahashi N. Dietary regulation of nuclear receptors in obesity-related metabolic syndrome. Asia Pac. J. Clin. Nutr. 2008;17(Suppl 1):126–130.
- Ito A, Suganami T, Miyamoto Y, Yoshimasa Y, Takeya M, Kamei Y, Ogawa Y. Role of MAPK phosphatase-1 in the induction of monocyte chemoattractant protein-1 during the course of adipocyte hypertrophy. J. Biol. Chem. 2007;282:25445–25452.10.1074/jbc.M701549200
- Lee JS, Kim HJ, Lee YS. A new anti-HIV flavonoid glucuronide from Chrysanthemum morifolium. Planta Med. 2003;69:859–861.
- Sugawara T, Igarashi K. Cultivar variation in flavonoid components and radical scavenging activity of polyphenol fractions among edible chrysanthemum flowers. Nippon Shokuhin Kagaku Kogaku Kaishi. 2009;56:600–604.10.3136/nskkk.56.600
- Meng S, Cao J, Feng Q, Peng J, Hu Y. Roles of chlorogenic acid on regulating glucose and lipids metabolism: a review. Evid. Based. Complement. Alternat. Med. 2013;2013:801457.
- Xu N, Zhang L, Dong J, Zhang X, Chen Y-G, Bao B, Liu J. Low-dose diet supplement of a natural flavonoid, luteolin, ameliorates diet-induced obesity and insulin resistance in mice. Mol. Nutr. Food Res. 2014;58:1258–1268.10.1002/mnfr.v58.6
- Panda S, Kar A. Apigenin (4’,5,7-trihydroxyflavone) regulates hyperglycaemia, thyroid dysfunction and lipid peroxidation in alloxan-induced diabetic mice. J. Pharm. Pharmacol. 2007;59:1543–1548.10.1211/jpp.59.11.0012
- Hsu C-L, Huang S-L, Yen G-C. Inhibitory effect of phenolic acids on the proliferation of 3T3-L1 preadipocytes in relation to their antioxidant activity. J. Agric. Food. Chem. 2006;54:4191–4197.10.1021/jf0609882
- Seelinger G, Merfort I, Schempp CM. Anti-oxidant, anti-inflammatory and anti-allergic activities of luteolin. Planta Med. 2008;74:667–677.
- Upadhyay R, Mohan Rao LJ. An outlook on chlorogenic acids-occurrence, chemistry, technology, and biological activities. Crit. Rev. Food Sci. Nutr. 2013;53:968–984.10.1080/10408398.2011.576319