Abstract
β-Thujaplicin, one of the major constituents in Chamaecyparis obtusa, has been demonstrated to exert different health beneficial efficacy, but the role of β-thujaplicin in regulating mammary tumorigenesis has not been investigated. In this study, we found that β-thujaplicin significantly suppressed the proliferation through arresting the cell cycle transition from G1 to S phase as well as inhibited the expression of cell cycle-related proteins, cyclin D1, and cyclin-dependent kinase 4 (CDK4) in MCF-7 and T47D luminal subtype breast cancer cells. In addition, estrogen receptor α (ER-α) was down-regulated by β-thujaplicin via enhanced proteolysis by ubiquitination, which led to cell growth inhibition. These results suggest that β-thujaplicin may be considered as a potent agent regulating the hormone sensitive mammary tumorigenesis.
Graphical Abstract
β-Thujaplicin from Chamaecyparis obtus inhibits proliferation (G0/G1 arrest) of human breast cancer cells via modulating the estrogen receptor α, cyclin D1, and CDK4.

β-Thujaplicin (Hinokitiol, Fig. (A)) identified from Chamaecyparis obtusa and Thuja plicata is a natural tropolone-based monoterpenoid and considered as the major constituent in the wood of Chamaecyparis obtuse.Citation1,2) In addition to its well-known anti-bacterial activity,Citation3,4) there are accumulating evidences demonstrating the health beneficial efficacies of β-thujaplicin including neuroprotective,Citation5) anti-inflammatory,Citation6,7) and especially cancer suppressive activities.Citation8–11) For example, β-thujaplicin induced G1 arrest by regulating p27, Skp2, and cyclin-dependent kinase 2 (CDK2) functions in human malignant melanoma cells and suppressed cell proliferation by S phase arrest and apoptosis induction in human colon cancer cells and xenograft animal model.Citation8,9) Recently, β-thujaplicin has also been reported to inhibit tumor cell proliferation through activating the autophagic pathway in lung and colon cancer cells.Citation10,11) However, there are few studies evaluating the role of β-thujaplicin in regulating the mammary tumorigenesis.
Fig. 1. Effect of β-thujaplicin on cell proliferation and cell cycle in MCF-7 cells.
Notes: (A) The structure of β-thujaplicin. (B) The cells (5000 cells/well in a 96-well plate) were treated with β-thujaplicin at the indicated concentrations (5–50 μM) for 72 h, and cell viability (%) was determined by MTT and BrdU assay. (C) The cells (1 × 106 cells) were treated with β-thujaplicin at the indicated concentrations (10–50 μM) for 24 h, and the cellular distribution was determined with flow cytometry. Values represent means ± SD. A–E and a–e, different letters indicate statistical differences at p < 0.05.
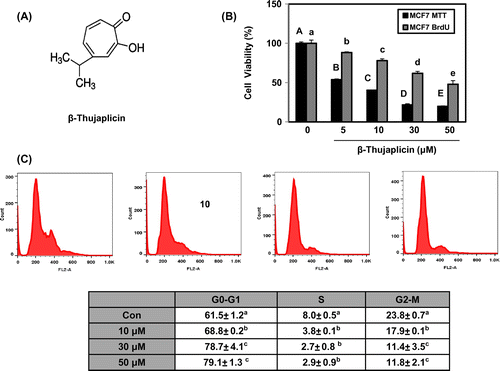
Breast cancer is the most commonly diagnosed cancer and one of the leading causes of cancer death in women worldwide.Citation12) Microarray-based molecular profiling has identified the subtypes of breast tumors into five groups, luminal A, luminal B, Her2-positive, basal-like, and normal breast-like tumors,Citation13,14) suggesting that breast cancer is a highly heterogeneous disease. Estrogen receptor (ER) is one of the key molecular markers in luminal subtypes constituting approximately 60–80% of breast tumors.Citation15) To take care of ER-positive breast cancer, different selective ER modulators have been developed, and tamoxifen, acting as a partial ER agonist, was approved by food and drug administration for reducing mammary tumor incidence in women. However, it is unfortunate that the resistance to tamoxifen treatment in an initially responsive mammary tumor has been reported,Citation16) which suggests the necessity of investigating the novel agents modulating the estrogen signaling in breast cancer.
In this study, we investigated the role of β-thujaplicin in regulating the proliferation in MCF-7 and T47D human breast cancer cells, characterized as luminal subtype of molecular profiling (ER-positive, progesterone receptor (PR)-positive, and Her2 negative),Citation17) and modulating efficacy in ER signaling.
Materials and methods
General experimental procedure and cell culture
β-Thujaplicin was purchased from Sigma (Cat. #: 469521, purity >98.5%, ST. Louis, MO, USA). MCF-7 and T47D cells were obtained from American Type Culture Collection (ATCC, Manassas, VA, USA) and cultured in Dulbecco’s modified Eagle’s medium (DMEM) and Roswell Park Memorial Institute medium (RPMI) 1640 media, respectively, supplemented with 10% fetal bovine serum (FBS), 1% penicillin/streptomycin at 37 °C, 5% CO2.
Cell proliferation assay
Cells were seeded in 96-well plate and treated with β-thujaplicin at different conditions. Four hours before cell harvest, 3-(4,5-dimethulthiazol-2-yl)-2,5-diphenyltetrazolium bromide (MTT, Sigma) solution was added to each well. The dark blue formazan crystals formed were dissolved in DMSO, and the absorbance at 570 nm was measured.
BrdU assay
Cells were seeded 5000 cells/well in 96-well plate and then incubated for overnight. After incubation, cells were treated with β-thujaplicin at indicated conditions for 72 h. According to the manufacturer’s instructions of BrdU kit (Millipore, Billerica, MA, USA), 12 h before cell harvest, BrdU was added to each well. At the end of treatment, the supernatant was removed, and the anti-BrdU antibody, its secondary antibody, and substrate were added to each well consecutively. The absorbance at 570 nm was measured, and the inhibition of DNA synthesis was described compared to the control.
Western blot analysis
Cells were lysed in radioimmunoprecipitation assay (RIPA) buffer (10 mM Tris-HCl, 5 mM EDTA, 150 mM NaCl, 1% Triton X-100, 1% sodium deoxycholate, 0.1% SDS, 0.1 mM Na3VO4, 1% phenylmethylsulfonyl fluoride, 1% protease inhibitor). The proteins were separated by 10% SDS-PAGE and transferred to polyvinylidene fluoride (PVDF) membranes (Milipore, Billerica, MA, USA). The primary antibodies against ER-α, Cyclin D1, Cyclin B, CDK4, CDK2, and ubiquitin (Santa Cruz Biotechnology Inc., Santa Cruz, CA, USA) and secondary antibodies (Santa Cruz Biotechnology Inc., Santa Cruz, CA, USA) were used. Protein expression levels were visualized by chemiluminescence reagent (GE healthcare, Little Chalfont, UK). The blots were quantified using EZ west Lumi plus (ATTO, Tokyo, Japan).
Cell cycle analysis
After the treatment of β-thujaplicin for 24 h in the cells, DNA was stained with propidium iodide and then analyzed with FACSCalibur flow cytometer (BD Biosciences, Franklin Lakes, NJ, USA). Data were interpreted using FlowJo software (Ashland, OR, USA).
Statistical analysis
Statistical differences were determined using one-way ANOVA or Student’s t-test. Differences were considered statistically significant at p < 0.05.
Results
β-Thujaplicin significantly suppressed the cellular proliferation and induced G0/G1 cell cycle arrest in MCF-7 cells
Here, we first found β-thujaplicin exerted the significant suppression of MCF-7 cell growth at the concentration from 5 to 50 μM where the IC50 was 8 μM using MTT assay and inhibited DNA synthesis in a dose-dependent manner using BrdU assay (Fig. (B)). To investigate the effect of β-thujaplicin on cell cycle, the flow cytometry analysis was performed after treatment of β-thujaplicin at 10, 30, and 50 μM in MCF-7 cells for 24 h. The cell population at G0/G1 phase was found to be increased from 61.5, 68.8, 78.7, and 79.1%, respectively, whereas the populations at S and G2/M phase were reduced in a dose-dependent manner (Fig. (C)). However, there were no significant changes in sub-G1 contents and poly (ADP-ribose) polymerase expression by β-thujaplicin treatment, indicating that apoptosis is not induced in MCF-7 cells (data not shown). These results suggest that β-thujaplicin may induce cell cycle arrest at G0/G1 phase, which resulted in inhibiting the cell proliferation.
β-Thujaplicin inhibited the cell cycle modulators, cyclin D1 and CDK4, and ER-α without affecting the expression of ER-β in MCF-7 cells
Generally, the cell cycle is regulated with the critical mediators including cyclin-dependent kinases (CDKs) and cyclins, where CDK 4/6 are involved in regulating G1 phase, CDK2 is in S phase, and CDK 2/1 are in M phase. In case of cyclins, cyclin D and E regulate G1 phase, cyclin E and A are in S phase, and cyclin A and B are in M phase.Citation18) Here, β-thujaplicin was found to exert the significant suppression of cyclin D1 and CDK4 expression from 10 μM without affecting cyclin B or CDK2 (Fig. (A)), which led to arrest the transition of cells from G1 to S phase (Fig. (B)). ER-α has been considered as a critical molecular target for prevention and/or treatment of hormone sensitive breast cancer. As shown in Fig. (B), β-thujaplicin suppressed the protein level of ER-α in a dose-dependent manner without affecting the expression of ER-β. In a time-dependent experiment, β-thujaplicin began to show inhibiting activity in ER-α expression from 3 h treatment (Fig. (B)). These results demonstrate the strong regulation of ER-α expression by β-thujaplicin in hormone sensitive human breast cancer cells.
Fig. 2. Effect of β-thujaplicin on the expression of cell cycle-related proteins and critical biomarkers in breast cancer ERs in MCF-7 cells.
Notes: The cells (5 × 105 cells/well in a 6-well plate) were treated with β-thujaplicin at different concentrations (10–50 μM) for 24 h or different time points (30 min to 24 h) at 50 μM. (A) Immunoblotting against cyclin D1, cyclin B, CDK4, CDK2, and β-actin was performed. (B) Immunoblotting against ER-α and ER-β was performed. After the quantification, the protein expressions were expressed as means ± SD. *p < 0.05, **p < 0.01 compared to the control group.
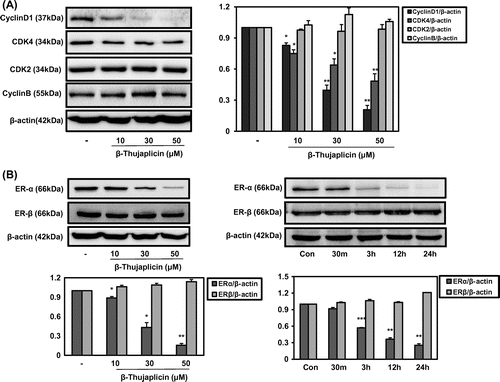
β-Thujaplicin induced ER-α degradation resulting in cyclin D1 degradation and the proliferation of MCF-7 cells
To determine whether the down-regulation of ER-α by β-thujaplicin was mediated via regulating the protein degradation pathway, a well-known 26S proteasome inhibitor MG132 was treated together with β-thujaplicin at 50 μM and confirmed that it strongly reduced the protein expression of ER-α, cyclin D1, and CDK4, where it was significantly recovered in the presence of MG132 at 10 μM (Fig. (A)). Furthermore, MG132 treatment dramatically enhanced the accumulation of ubiquitinated proteins in the cells through proteasome inhibition, and co-treatment with β-thujaplicin increased those levels (Fig. (B)), indicating the stimulation of ubiquitination process by β-thujaplicin in MCF-7 cells. In the absence of MG132, β-thujaplicin slightly reduced the level of ubiquitinated proteins, which might be from activating the protein degradation pathway (Fig. (B)). In addition, the treatment of MG132 together with β-thujaplicin significantly abolished the reduced cell viability from 25.1% by β-thujaplicin to 53.5% by co-treatment (Fig. (C)). These findings support that β-thujaplicin may enhance the proteolysis of ER-α and inhibit cyclin D1 and CDK4 expression, leading to suppression of the proliferation of MCF-7 cells.
Fig. 3. Effect of β-thujaplicin on the recovery of ER-α, cyclin D1, CDK4, and the reversal of the cellular growth inhibition after inhibition of proteasome by MG132 in MCF-7 cells.
Notes: (A) The cells (5 × 105 cells/well in a 6-well plate) were treated with β-thujaplicin (50 μM) and/or MG132 (10 μM) for 24 h, and immunoblotting against ER-α, cyclin D1, CDK4 was performed. After the quantification, the protein expressions were expressed as means ± SD. *p < 0.05 compared to β-thujaplicin treated group. (B) The cells (5 × 105 cells/well in a 6-well plate) were treated with β-thujaplicin (50 μM) and/or MG132 (10 μM) for 24 h, and immunoblotting against ubiquitin was performed. (C) The cells (5000 cells/well in a 96-well plate) were treated with β-thujaplicin (50 μM) and/or MG132 (1 μM) for 72 h, and cell viability (%) was determined by MTT assay. Values represent means ± SD. ***p < 0.001 compared to β-thujaplicin treated group.
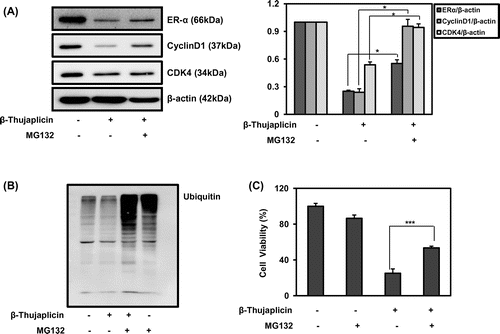
β-Thujaplicin inhibited the cell proliferation through regulating ER-α, cyclin D1, and CDK4 in T47D cells
T47D human breast cancer cell line is another widely used hormone sensitive cell line categorized as luminal subtype forming invasive ductal carcinoma.Citation17) Using T47D cells, the experiments for elucidating the effect of β-thujaplicin on inhibiting the cell proliferation and regulating the expression of cell cycle mediators and ER-α were performed. As shown in Fig. (A), β-thujaplicin (5–50 μM) exerted dose-dependent suppression of cell proliferation, where IC50 was 29 μM and inhibition of DNA synthesis (Fig. (A)). The expression of ER-α, cyclin D1, and CDK4 was also significantly inhibited by β-thujaplicin at different concentration, 10, 30, and 50 μM (Fig. (B)). In addition, the proteasome inhibition by MG132 was repeated to reverse the down-regulated levels of ER-α, cyclin D1, and CDK4 by β-thujaplicin in T47D cells (Fig. (C)). In the ubiquitination experiment, the treatment of β-thujaplicin together with MG132 increased the level on ubiquitinated proteins, where β-thujaplicin alone reduced it (Fig. (D)). These results strongly support that β-thujaplicin shared the same molecular targets for regulating the cell proliferation in ER-positive luminal breast cancer cells.
Fig. 4. Effect of β-thujaplicin on cell proliferation, cell cycle-related proteins expression, and ER-α degradation in T47D cells.
Notes: (A) The cells (5000 cells/well in a 96-well plate) were treated with β-thujaplicin at the indicated concentrations (5–50 μM) for 72 h, and cell viability (%) was determined by MTT assay. Values represent means ± SD. A–D and a–e, different letters indicate statistical differences at p < 0.05. (B) The cells (5 × 105 cells/well in a 6-well plate) were treated with β-thujaplicin at 10, 30, 50 μM, and immunoblotting against ER-α, cyclin D1, and CDK4 was performed. (C and D) The cells (5 × 105 cells/well in a 6-well plate) were treated with β-thujaplicin alone (50 μM) or together with MG132 (10 μM) for 24 h, and immunoblotting against ER-α, cyclin D1, CDK4, and ubiquitin was then performed. After the quantification, the protein expressions were expressed as means ± SD. *p < 0.05, **p < 0.01 compared to β-thujaplicin treated group.
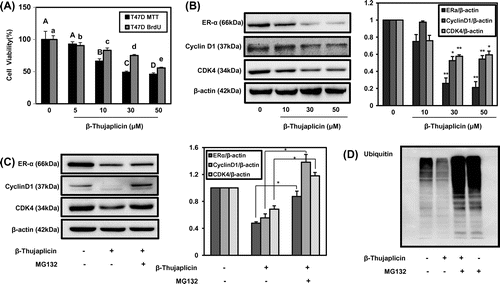
Discussion
It is well known that the cell cycle is mediated by molecular mediators such as cyclins and CDKs.Citation18) Cyclin D1, which is the early Gl phase marker, was reported to be highly amplified in some subset of mammary tumors, and inhibitors of cyclin D partners, CDK 4 and 6, have been developed to treat the hormone sensitive breast cancer,Citation19,20) indicating that the regulation of cell cycle is one of critical approaches to manage the mammary tumorigenesis. In this study, we found that β-thujaplicin identified from Chamaecyparis obtuse exerted the strong suppressive effect on the expression of G1 phase modulators, cyclin D1, and CDK4 (Figs. (A) and (B)), which might result in G0/G1 cell cycle arrest (Fig. (B)) and cell growth inhibition (Figs. (A) and (A)) in ER-positive breast cancer cells, MCF-7 and T47D.
Estrogen signaling is mediated via ERs including ER-α and ER-β. Interestingly, however, the size of ligand binding pockets in ER-α and ER-β is different and there are only 17% homology in activation function (AF)-1 domain and 55% homology in AF-2 domain between ER-α and ER-β,Citation21) indicating that ER-α and ER-β possess the distinct set of regulatory genes,Citation22) which led to different cellular responses and clinical outcomes. Many studies have demonstrated that ER-α agonist stimulated the proliferation of mammary cells which can increase the risk of breast cancer while ER-β agonist blocked inflammatory responses and inhibited the growth of breast cancer cells.Citation23−25) Here, the effect of β-thujaplicin on regulating the expression of ER-α and/or ER-β was investigated and it showed the significant suppression of ER-α expression in a dose- and time-dependent manner without affecting ER-β in ER-positive breast cancer cells (Fig. (B)), suggesting the possible roles of β-thujaplicin in inhibiting the cellular proliferation through ER-α regulation.
Ubiquitin–proteasome-dependent proteolysis, one of the post-transcriptional modifications, is an important regulatory pathway determining the protein expression levels.Citation26) ER-α ubiqutination is one of the major mechanisms to determine the stability and degradation of ER-α, where several E3 ligases responsible for ubiquitinating ER-α including mouse double minute 2 (MDM2) and breast cancer susceptibility gene 1/Brca1-associated RING domain protein 1 (BRCA1/BARD1) were characterized.Citation27,28) With the treatment of well-known proteasome inhibitor MG132, β-thujaplicin was found to increase the level of ubiquitinated proteins (Figs. (B) and (D)) and down-regulated ER-α by β-thujaplicin was significantly recovered in both MCF-7 and T47D cells (Figs. (A) and (C)). These results support that β-thujaplicin may enhance the ubiquitination of ER-α which lead to its degradation in ER-positive breast cancer cells although further studies to determine the exact molecular targets are necessary.
Recently, it was reported that estrogen response element was existed in cyclin D1 promoter in ER-positive T47D human breast cancer cells.Citation29) demonstrating that cyclin D1 is one of the target genes of ER-α in human mammary epithelial cells. In line with the result, we also found that β-thujaplicin significantly suppressed the expression of cyclin D1 (Figs. (A) and (B)) and blocking the degradation of ER-α by proteasome inhibition was demonstrated to result in the recovery of cyclin D1 expression in both MCF-7 and T47D cells (Figs. (A) and (C)). In addition, co-treatment of β-thujaplicin and MG-132 strongly reduced the anti-proliferative activity of β-thujaplicin in MCF-7 cells (Fig. (C)). These findings elucidate that β-thujaplicin inhibited the expression of cyclin D1, one of ER-α target proteins, by regulating ER-α degradation and eventually suppressed the proliferation of hormone sensitive human breast cancer cells. However, the recovery of ER-α by treatment of MG132 is relatively weak compared to that of cyclin D1 and CDK4 (Figs. (A) and (C)), indicating ER-α regulation by β-thujaplicin is partially involved in cyclin D1 and CDK4 expression. Several studies have demonstrated that β-thujaplicin significantly suppressed the expression of cyclin D1 in H1975 human lung adenocarcinoma cells and FEM human melanoma cells which is not expressing ER-α.Citation8,11) In addition, β-thujaplicin was reported to exert inhibitory activity Skp2 ubiquitin ligase targeting p27 ubiquitination and its degradation.Citation8) These findings suggest that β-thujaplicin may modulate the additional ubiquitin-dependent protein degradations which results in down-regulation of cyclin D1 and CDK4 although further studies are necessary.
To our best knowledge, it is the first report that β-thujaplicin commonly reduced the ER-α expression via inducing the proteolysis by ubiquitination and suppressed cell cycle mediators, cyclin D1 and CDK4 resulting in G0/G1 arrest, and eventually inhibited the cellular proliferation in both MCF-7 and T47D luminal subtype of human breast cancer cells. These results suggest that β-thujaplicin may be considered as a potent agent regulating the hormone sensitive mammary tumorigenesis.
Acknowledgments
This work was supported by Mid-career Researcher Program (NRF-2012R1A2A2A02011748) and by the Bio-Synergy Research Project (NRF-2013M3A9C4078156) of the Ministry of Science, ICT and Future Planning through the National Research Foundation.
Additional information
Funding
References
- Erdtman H, Gripenberg J. Antibiotic substances from the heart wood of Thuja plicata Don. Nature. 1948;161:719.10.1038/161719a0
- Nozoe T. Uber die Farbstoffe im Holzteile des “Hinoki”-Baumes. I: Hinokitin und Hinokitiol [About the dyses in the wooden parts of the “Hinoki” tree]. Bull. Chem. Soc. Japan. 1936;3:295–298.
- Trust TJ, Coombs RW. Antibacterial activity of beta-thujaplicin. Can. J. Microbiol. 1973;19:1341–1346.10.1139/m73-216
- Yamano H, Yamazaki T, Sato K, Shiga S, Hagiwara T, Ouchi K, Kishimoto T. In vitro inhibitory effects of hinokitiol on proliferation of Chlamydia trachomatis. Antimicrob. Agents Chemother. 2005;49:2519–2521.10.1128/AAC.49.6.2519-2521.2005
- Jayakumar, T, Hsu, WH, Yen, TL, Luo, JY, Kuo, YC, Fong, TH, Sheu, JR. Hinokitiol, a natural tropolone derivative, offers neuroprotection from thromboembolic stroke in vivo. Evidence-Based Complement. Alternat. Med. 2013; 2013:Article ID 840487, 8 pages.
- Byeon SE, Lee YG, Kim JC, Han JG, Lee HY, Cho JY. Hinokitiol, a natural tropolone derivative, inhibits TNF-alpha production in LPS-activated macrophages via suppression of NF-kappaB. Planta. Med. 2008;74:828–833.10.1055/s-2008-1074548
- Shih MF, Chen LY, Tsai PJ, Cherng JY. In vitro and in vivo therapeutics of beta-thujaplicin on LPS-induced inflammation in macrophages and septic shock in mice. Int. J. Immunopathol. Pharmacol. 2012;25:39–48.
- Liu S, Yamauchi H. p27-Associated G1 arrest induced by hinokitiol in human malignant melanoma cells is mediated via down-regulation of pRb, Skp2 ubiquitin ligase, and impairment of Cdk2 function. Cancer Lett. 2009;286:240–249.10.1016/j.canlet.2009.05.038
- Lee YS, Choi KM, Kim W, Jeon YS, Lee YM, Hong JT, Yun YP, Yoo HS. Hinokitiol inhibits cell growth through induction of S-phase arrest and apoptosis in human colon cancer cells and suppresses tumor growth in a mouse xenograft experiment. J. Nat. Prod. 2013;76:2195–2202.10.1021/np4005135
- Wang WK, Lin ST, Chang WW, Liu LW, Li TY, Kuo CY, Hsieh JL, Lee CH. Hinokitiol induces autophagy in murine breast and colorectal cancer cells. Environ. Toxicol. Forthcoming 2015. doi:10.1002/tox.22023.
- Li LH, Wu P, Lee JY, Li PR, Hsieh WY, Ho CC, Ho CL, Chen WJ, Wang CC, Yen MY, Yang SM, Chen HW. Hinokitiol induces DNA damage and autophagy followed by cell cycle arrest and senescence in gefitinib-resistant lung adenocarcinoma cells. Plos One. 2014;9:e104203.10.1371/journal.pone.0104203
- Jemal A, Bray F, Center MM, Ferlay J, Ward E, Forman D. Global cancer statistics. CA Cancer J. Clin. 2011;61:69–90.10.3322/caac.v61:2
- Perou CM, Sorlie T, Eisen MB, van de Rijn M, Jeffrey SS, Rees CA, Pollack JR, Ross DT, Johnsen H, Akslen LA, Fluge O, Pergamenschikov A, Williams C, Zhu SX, Lonning PE, Borresen-Dale AL, Brown PO, Botstein D. Molecular portraits of human breast tumours. Nature. 2000;406:747–752.10.1038/35021093
- Sorlie T, Tibshirani R, Parker J, Hastie T, Marron JS, Nobel A, Deng S, Johnsen H, Pesich R, Geisler S, Demeter J, Perou CM, Lonning PE, Brown PO, Borresen-Dale AL, Botstein D. Repeated observation of breast tumor subtypes in independent gene expression data sets. Proc. Natl. Acad. Sci. USA. 2003;100:8418–8423.10.1073/pnas.0932692100
- Rakha EA, Ellis IO. Triple-negative/basal-like breast cancer: review. Pathology. 2009;41:40–47.10.1080/00313020802563510
- Chang M. Tamoxifen resistance in breast cancer. Biomol. Ther. (Seoul). 2012;20:256–267.10.4062/biomolther.2012.20.3.256
- Kao J, Salari K, Bocanegra M, Choi YL, Girard L, Gandhi J, Kwei KA, Hernandez-Boussard T, Wang P, Gazdar AF, Minna JD, Pollack JR. Molecular profiling of breast cancer cell lines defines relevant tumor models and provides a resource for cancer gene discovery. Plos One. 2009;4:e6146.10.1371/journal.pone.0006146
- Ivanchuk SM, Rutka JT. The cell cycle: accelerators, brakes, and checkpoints. Neurosurgery. 2004;54:692–699.10.1227/01.NEU.0000109534.28063.5D
- Burandt E, Grunert M, Lebeau A, Choschzick M, Quaas A, Janicke F, Muller V, Scholz U, Bokemeyer C, Petersen C, Geist S, Paluchowski P, Wilke C, Heilenkotter U, Simon R, Sauter G, Wilczak W. Cyclin D1 gene amplification is highly homogeneous in breast cancer. Breast Cancer. 2014. doi:10.1007/s12282-12014-10538-y.
- Migliaccio I, Di Leo A, Malorni L. Cyclin-dependent kinase 4/6 inhibitors in breast cancer therapy. Curr. Opin. Oncol. 2014;26:568–575.
- Leitman DC, Paruthiyil S, Vivar OI, Saunier EF, Herber CB, Cohen I, Tagliaferri M, Speed TP. Regulation of specific target genes and biological responses by estrogen receptor subtype agonists. Curr. Opin. Pharmacol. 2010;10:629–636.10.1016/j.coph.2010.09.009
- Vivar OI, Zhao X, Saunier EF, Griffin C, Mayba OS, Tagliaferri M, Cohen I, Speed TP, Leitman DC. Estrogen receptor beta binds to and regulates three distinct classes of target genes. J. Biol. Chem. 2010;285:22059–22066.10.1074/jbc.M110.114116
- Harris HA, Albert LM, Leathurby Y, Malamas MS, Mewshaw RE, Miller CP, Kharode YP, Marzolf J, Komm BS, Winneker RC, Frail DE, Henderson RA, Zhu Y, Keith JC. Evaluation of an estrogen receptor-beta agonist in animal models of human disease. Endocrinology. 2003;144:4241–4249.10.1210/en.2003-0550
- Helguero LA, Faulds MH, Gustafsson JA, Haldosen LA. Estrogen receptors alfa (ERalpha) and beta (ERbeta) differentially regulate proliferation and apoptosis of the normal murine mammary epithelial cell line HC11. Oncogene. 2005;24:6605–6616.10.1038/sj.onc.1208807
- Paruthiyil S, Parmar H, Kerekatte V, Cunha GR, Firestone GL, Leitman DC. Estrogen receptor beta inhibits human breast cancer cell proliferation and tumor formation by causing a G2 cell cycle arrest. Cancer Res. 2004;64:423–428.10.1158/0008-5472.CAN-03-2446
- Zhou W, Slingerland JM. Links between oestrogen receptor activation and proteolysis: relevance to hormone-regulated cancer therapy. Nat. Rev. Cancer. 2014;14:26–38.
- Duong V, Boulle N, Daujat S, Chauvet J, Bonnet S, Neel H, Cavailles V. Differential regulation of estrogen receptor alpha turnover and transactivation by Mdm2 and stress-inducing agents. Cancer Res. 2007;67:5513–5521.10.1158/0008-5472.CAN-07-0967
- Ma Y, Fan S, Hu C, Meng Q, Fuqua SA, Pestell RG, Tomita YA, Rosen EM. BRCA1 regulates acetylation and ubiquitination of estrogen receptor-alpha. Mol. Endocrinol. 2010;24:76–90.10.1210/me.2009-0218
- Giulianelli S, Vaque JP, Soldati R, Wargon V, Vanzulli SI, Martins R, Zeitlin E, Molinolo AA, Helguero LA, Lamb CA, Gutkind JS, Lanari C. Estrogen receptor alpha mediates progestin-induced mammary tumor growth by interacting with progesterone receptors at the cyclin D1/MYC promoters. Cancer Res. 2012;72:2416–2427.10.1158/0008-5472.CAN-11-3290