Abstract
SNQ2 was identified as a caffeine-resistance gene by screening a genomic library of Saccharomyces cerevisiae in a multicopy vector YEp24. SNQ2 encodes an ATP-binding cassette transporter and is highly homologous to PDR5. Multicopy of PDR5 also conferred resistance to caffeine, while its resistance was smaller than that of SNQ2. Residual caffeine contents were analyzed after transiently exposing cells to caffeine. The ratios of caffeine contents were 21.3 ± 8.8% (YEp24-SNQ2) and 81.9 ± 8.7% (YEp24-PDR5) relative to control (YEp24, 100%). In addition, multicopies of SNQ2 or PDR5 conferred resistance to rhodamine 6G (R6G), which was widely used as a substrate for transport assay. R6G was exported by both transporters, and their efflux activities were inhibited by caffeine with half-maximal inhibitory concentrations of 5.3 ± 1.9 (YEp24-SNQ2) and 17.2 ± 9.6 mM (YEp24-PDR5). These results demonstrate that Snq2p is a more functional transporter of caffeine than Pdr5p in yeast cells.
Graphical Abstract
Multicopies of SNQ2 or PDR5 conferred caffeine resistance; single knockout (snq2Δ, pdr5Δ) did not lead to caffeine sensitivity, although snq2Δ pdr5Δ double knockout led to significant sensitivity.
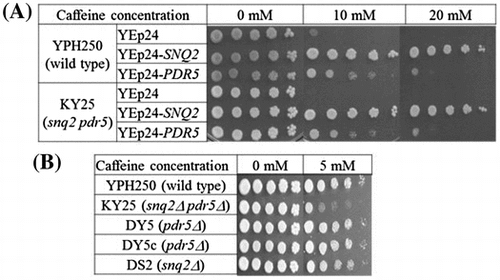
Caffeine (1,3,7-trimethylpurine-2,6-dione) is a purine derivative found in beverages and foods such as coffee, tea, and chocolate, and is known to stimulate the central nervous system and to have a diuretic effect via inhibition of adenosine receptors in higher animals.Citation1,2) In addition, caffeine can affect cell cycle checkpoints and inhibits DNA repair and carcinogen metabolism.Citation3) Caffeine is also known to be an inhibitor of mammalian target of rapamycin (TOR), which is an atypical serine/threonine kinase and is a central controller of cell growth, proliferation, metabolism, and angiogenesis.Citation4) Recently, the right dose of caffeine is expected to reduce risk of myocardial infarction and Alzheimer’s disease.Citation5)
Generally, all cells have developed resistance to toxic compounds, and the resistance is based on different mechanisms. The phenotypes of multidrug resistance (MDR) and pleiotropic drug resistance (PDR) are often associated with overexpression and/or activation of ATP-binding cassette (ABC) transporters, which export a variety of structurally and functionally unrelated drugs out of cells or into vacuoles (or lysosomes).Citation6,7) Detoxification enzymes such as cytochrome P450 and glutathione S-transferase are also involved in the resistance to toxic compounds.Citation8,9) In mammals, caffeine is known to be metabolized by a P450 enzyme,Citation10) while no caffeine transporter has been identified. Saccharomyces cerevisiae is widely used as a eukaryotic model organism, and resistance of the yeast has been extensively studied against various drugs. However, neither transporter nor detoxification enzyme for caffeine has been reported.
In this study, we screened for caffeine-resistance genes using a S. cerevisiae genomic library based on a multicopy vector YEp24. As a result, SNQ2 was identified and the gene encodes a plasma membrane ABC transporter.Citation11,12) Snq2p is functionally homologous to Pdr5p, which is also a plasma membrane ABC transporter.Citation6,7,13) Snq2p and Pdr5p are full-sized ABC transporters and belong to the ABCG subfamily according to its sequence similarity and overall architecture.Citation6,7) Here, we have investigated the functional roles of Snq2p, comparing with those of Pdr5p in caffeine-resistance strategies, and demonstrate that Snq2p and Pdr5p mediate caffeine efflux and resistance in S. cerevisiae.
Materials and methods
S. cerevisiae strains, culture conditions, and DNA manipulation. The S. cerevisiae strains used in this study are listed in Table . Cells were cultured on YPD medium (1% yeast extract, 2% peptone, 2% glucose, pH 5.5–6.0) or synthetic complete (SC) medium (2% glucose, 0.67% yeast nitrogen base without amino acids supplemented with appropriate amino acids and bases, pH 5.5–6.0) at 30 °C. Agar plates were prepared using 1.5–1.8% (w/v) agar. All DNA manipulations were performed essentially as described by Sambrook et al.Citation17) and Burke et al.Citation18). Restriction endonucleases and DNA-modifying enzymes were purchased from New England Biolabs (MA, USA) and Toyobo (Osaka, Japan) and were used as recommended by their suppliers. Escherichia coli DH5α was used for plasmid preparation. E. coli transformants were cultured in LB (0.5% yeast extract, 1% Peptone, 1% NaCl, pH 7.2) medium supplemented with 50 μg/mL ampicillin at 37 °C. DNA sequencing was performed by the Sanger method,Citation19) on an Applied Biosystems 373A DNA sequencer or BigDye Terminator ver. 3.1 Cycle Sequencing kit (Applied Biosystems, CA, USA) and an ABI PRISM 310 NT Genetic Analyzer. Oligonucleotide primers were purchased from Hokkaido System Science (Hokkaido, Japan).
Table 1. S. cerevisiae strains used in this study.
Screening of caffeine-resistance genes. To construct genomic library, genomic DNA of S288C was partially digested with Sau3AI, and the resulting DNA fragments (approximately 3–8 kb) were collected and cloned into the Bam HI site of YEp24 multicopy vector (New England Biolabs, MA, USA) as described before.Citation16) Yeast strain YPH250 was used as a host, and clones which grew on SC medium containing 7 mM caffeine were selected and used in further analysis.
Construction of YEp24-SNQ2 and YEp24-PDR5. S288C genomic DNA was used as a PCR template in a 30-cycle reaction with LA-Taq DNA polymerase (Takara, Kyoto, Japan) according to manufacturer’s instructions. The DNA primers used to reclone the SNQ2 gene into YEp24 were the following: 5′-ATGGATCCACGGCAAGGAAGTGGCGCGAAA-3′ (the BamHI site is underlined) and 5′-ATTTGGTAATTGGGAGAGGTCGCTGCATG-3′ (the SphI site is downstream of the open reading frame). The primers used to construct YEp24 harboring the PDR5 gene were the following: 5′-AATAGCTAGCAGCACCTCGTTGGCGCAGTC-3′ (the NheI site is underlined) and 5′-TCAGGGCATGCTGAGTGCTCCTTCGACTTG-3′ (the SphI site is underlined). Appropriate restriction sites were created by PCR to facilitate insertion of each gene into the vector. The resulting PCR fragments contained SNQ2 nucleotides −735 to +5331 and PDR5 nucleotides −816 to +5240, in which the A of the translational start codon is defined as +1. Both fragments were designed to contain their own promoter. PCR products were restriction-digested and inserted into the corresponding sites of YEp24 to obtain YEp24-SNQ2 and YEp24-PDR5.
Construction of SNQ2 knockout strains. DNA fragments (snq2Δ::kanMX4) for SNQ2 knockout were prepared from the genomic DNA of the ID 3951 (snq2Δ::kanMX4) as described.Citation16) Knockout of SNQ2 was performed in the YPH250 (wild type) and DY5c (pdr5Δ::CgHIS3) cells by the one-step gene-replacement method to obtain DS2 (snq2Δ::kanMX4) and KY25 (snq2Δ::kanMX4 pdr5Δ::CgHIS3). Deletion of SNQ2 by the gene knockout was verified by PCR.
Spot analysis to evaluate drug resistance or sensitivity. Cells were grown in SC medium with shaking for 22–24 h at 30 °C; growth was measured by optical density at 660 nm (OD660). The cells were diluted to OD660 = 1.0 in 0.85% (w/v) NaCl and used for further analysis. Serial dilutions were spotted on YPD plates with various drug concentrations and incubated at 30 °C. Drugs used in this study were caffeine, 4-nitroquinoline-1-oxide (4-NQO), clotrimazole (CTZ), and rhodamine 6G (R6G). After culture for 3 days, images of cell growth were captured for a representative of at least six independent experiments.
TLC (thin layer chromatography) determination of intracellular caffeine content. To evaluate caffeine efflux, intracellular residual caffeine was extracted from cells exposed to caffeine and the content was determined. Cells (KY25 cells harboring plasmids) were grown overnight with shaking in 50 mL SC medium at 30 °C. A small portion of the culture was transferred to 500 mL YPD and then cultivated at 30 °C to OD660 of 0.6–0.8. To enhance gene expression of transporters, one-tenth volume of nitrogen supply (5% yeast extract, 10% peptone, 50 mM tryptophan, pH 6.0) was added to the culture, and incubation continued for 2 h.Citation20) Subsequently, 15 mM caffeine was added to the culture and incubated for another 2 h. Cells were harvested by centrifugation at 2000 × g for 5 min at 4 °C. Cells were washed four times [2% (w/v) glucose, 0.8% NaCl, 20 mM 2-(N-morpholino)ethanesulfonic acid (MES), pH 6.0] according to Mahé et al. with modifications.Citation21) Cells were divided into five fractions and kept at −30 °C before use.
Intracellular residual caffeine was extracted three times from the cells (from 100 mL culture) with 2 mL chloroform. The extract (~6 mL) was concentrated under nitrogen, applied to a TLC plate with fluorescent indicator (Merck, Darmstadt, Germany), and developed in chloroform:ethanol (9:1, v/v). The plate was viewed under an ultraviolet lamp. Densitometric evaluation of the caffeine spots was performed with NIH Image J software (http://rsb.info.nih.gov/ij/). The ratio of caffeine content was represented as the percentage relative to control (KY25 cells harboring YEp24), which was set at 100%. The mean and standard deviation of the relative ratio in three samples were computed, and data were analyzed using Student’s t-test.
Assay of R6G-efflux activity. The R6G-efflux activity was measured according to the modified method of Tsujimoto et al.Citation16) In brief, cells were cultured as described above (TLC determination of caffeine content), harvested, and washed with 50 mM MES buffer (pH 6.0). The cells were suspended in R6G solution [50 mM of MES (pH 6.0), 100 μM R6G, 5 mM 2-deoxyglucose, and 10 mM sodium azide] and incubated for 30 min at 30 °C. 2-Deoxyglucose and sodium azide were used to deplete ATP. Cells were washed and diluted in 50 mM MES buffer (pH 6.0) containing 5 mM glucose or 2-deoxyglucose. For the inhibition assay, caffeine was added to 50 mM MES buffer (pH 6.0) containing 5 mM glucose for final concentrations of 5, 10, and 21 mM. R6G fluorescence (excitation wavelength, 529 nm; emission wavelength, 553 nm) was monitored in a cell suspension (OD660 = 0.75) at 30 °C using a spectrofluorometer (RF-5300PC, Shimadzu, Kyoto, Japan). The final volume of the reaction mixture was 2 mL. Activity was defined as the difference in the maximum slope in fluorescence intensity in the presence of glucose and 2-deoxyglucose. The half-maximal inhibitory concentration (IC50) of caffeine was determined. The mean and standard deviation were calculated from four independent experiments.
Results
Screening of caffeine-resistance genes
We screened for caffeine-resistance genes in a YEp-24-based genomic library. Of approximately 342,000 clones obtained, 60 clones grew on SC medium containing 7 mM caffeine. Following exclusion of overlapping clones by restriction mapping and DNA sequencing, 19 clones were selected. One clone contained PDR1 encoding a transcription factor that regulated the expression of SNQ2, PDR5 and so on via pleiotropic drug response elements.Citation6,7,11–13) In the meantime, we selected the clone TM-1 which cells grew best on the caffeine-containing medium. TM-1 harbored an approximately 8-kb DNA fragment containing SNQ2 and neighbor genes. Although multicopy of SNQ2 conferred hyper-resistance to 4-NQO, triaziquone, and other chemicals,Citation11,12) involvement of SNQ2 to caffeine resistance has not been reported. As no transporter involved in caffeine resistance has been reported, TM-1 was used for further analysis. To identify the caffeine-resistant gene, SNQ2 was recloned by PCR and inserted into YEp24 vector. Multicopy of SNQ2 conferred significant resistance to caffeine, and the resistance was almost equal to that of TM-1 (data not shown). Thus, we have investigated the functional roles of Snq2p in caffeine-resistance strategies. The remaining clones will be described elsewhere.
SNQ2 and PDR5 mediate caffeine resistance
The multicopy effect of SNQ2 was also evaluated in a YPD medium. YPH250 cells harboring YEp24-SNQ2 (SNQ2-overexpressing cells) grew on YPD plates containing 10 mM or 20 mM caffeine (Fig. (A)) as well as on SC medium containing 7 mM caffeine (data not shown), although there was little or no cell growth in control cells (YPH250 cells harboring YEp24) in the presence of caffeine (Fig. (A)). These results indicated that multicopy of SNQ2 greatly affected on caffeine resistance in the yeast.
Fig. 1. Spot analysis of caffeine resistance.
Notes: Cells were serially diluted, and 5 μL of each diluted sample (1:1, 1:10, 1:50, 1:100, and 1:1000) was spotted on YPD plates in the presence or absence of caffeine as described in the Materials and Methods. Cells were then incubated at 30 °C for 3 days. (A) Caffeine resistance. Cells (YPH250 or KY25) harboring plasmid (YEp24, YEp24-SNQ2 or YEp24-PDR5). (B) Caffeine sensitivity. Wild-type (YPH250) and four knockout (DY5, DY5c, DS2, and KY25) cells.
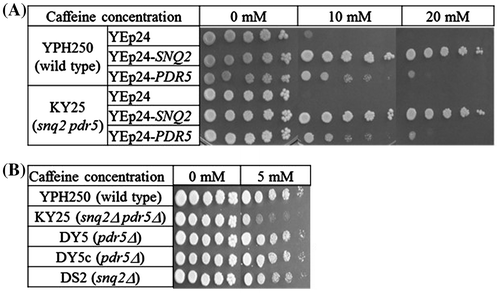
We also analyzed the effect of PDR5,Citation6,7,13) which is homologous to SNQ2 (39.3% identity and 70.8% similarity in primary sequence). Pdr5p is one of the best characterized ABC transporters and is known to be involved in resistance against various chemicals such as R6G and CTZ,Citation16,22–24) although involvement of PDR5 to caffeine resistance has not been reported. Multicopy of PDR5 also conferred resistance to 10 mM caffeine, but its resistance was smaller than that of SNQ2 (Fig. (A)). In the presence of 20 mM caffeine, YPH250 cells harboring YEp24-PDR5 (PDR5-overexpressing cells) grew very little, while YPH250 cells harboring YEp24-SNQ2 (SNQ2-overexpressing cells) grew significantly (Fig. (A)), showing that multicopy of SNQ2 was more effective.
We next evaluated whether knockout of SNQ2 and PDR5 would affect caffeine sensitivity. The growth of DS2 (snq2Δ::kanMX4), DY5 (pdr5Δ::kanMX4), and DY5c (pdr5Δ::CgHIS3) cells was almost similar to that of YPH250 (wild type) in the presence of 5 mM caffeine, whereas KY25 (snq2Δ::kanMX4 pdr5Δ::CgHIS3) cells were more sensitive to caffeine than the other four (YPH250, DS2, DY5, and DY5c) cells (Fig. (B)). Multicopies of SNQ2 or PDR5 conferred caffeine resistance (Fig. (A)); single knockout of SNQ2 or PDR5 did not lead to caffeine sensitivity, although double knockout of SNQ2 and PDR5 led to significant sensitivity (Fig. (B)). These results indicate that SNQ2 and PDR5 serve complementary functions in caffeine resistance. On the other hands, there was no difference in cell growth between DY5 (pdr5Δ::kanMX4) and DY5c (pdr5Δ::CgHIS3) (Fig. (B)), indicating that the marker genes (CgHIS3 and kanMX4) had no effect on caffeine resistance. Caffeine sensitivity tests showed that KY25 cells were more sensitive to caffeine than DS2, DY5, and DY5c as well as YPH250 cells (Fig. (B)), indicating that the multicopy analysis using wild-type or single knockout cells will be affected by SNQ2 and/or PDR5 genes on chromosome. Therefore, we judged that the use of KY25 cells would be appropriate in further analysis to clarify the difference between Snq2p and Pdr5p. Next, we confirmed the multicopy effect of SNQ2 or PDR5 in KY25 cells. Multicopies of SNQ2 or PDR5 also led to caffeine resistance in KY25 cells, and multicopy of SNQ2 was more effective in caffeine resistance than that of PDR5 in KY25 as well as YPH250 cells (Fig. (A)). YPH250 cells harboring YEp24 slightly grew in the presence of 10 mM caffeine, although KY25 cells harboring YEp24 could not grow (Fig. (A)). These results showed that the use of KY25 cells was more appropriate in further analysis.
Caffeine was exported by Snq2p and Pdr5p
It was shown that Snq2p and Pdr5p mediated caffeine resistance (Fig. ), but the mechanisms of resistance were not determined. We evaluated the intracellular caffeine contents by TLC analysis following the exposure of cells to caffeine. When cells (KY25 cells harboring YEp24, YEp24-SNQ2, or YEp24-PDR5) were exposed to 15 mM caffeine for 2 h as described in Materials and Methods, there was no difference in cell growth (data not shown). The exposure time (2 h) was similar to the doubling time of yeast; thus, exposure would not affect cell growth. After exposure, intracellular caffeine was extracted from the cells, separated by TLC, and quantified using NIH image. Our results showed that multicopies of SNQ2 or PDR5 led to the decrease of intracellular residual caffeine. The ratios of residual caffeine were 21.8 ± 8.8% (YEp24-SNQ2) and 81.9 ± 8.7% (YEp24-PDR5) relative to control (YEp24, 100%) (Fig. ). At least five independent experiments showed the same tendency of caffeine accumulation. These observations demonstrated that both transporters exported caffeine and that Snq2p was a more effective transporter of caffeine than Pdr5p.
Fig. 2. Intracellular caffeine accumulation.
Notes: KY25 (snq2Δ::kanMX4 pdr5Δ::CgHIS3) cells harboring plasmids were cultured and harvested as described in the Materials and Methods. Caffeine was extracted three times with chloroform and developed on silica gel TLC plates in chloroform:ethanol (9:1, v/v). Densitometry of the caffeine spots was performed with NIH Image J software (http://rsb.info.nih.gov/ij/). Data represent the mean ± SD relative to control (KY25 harboring YEp24) cells, which are set to 100%. *p < 0.05; **p < 0.001.
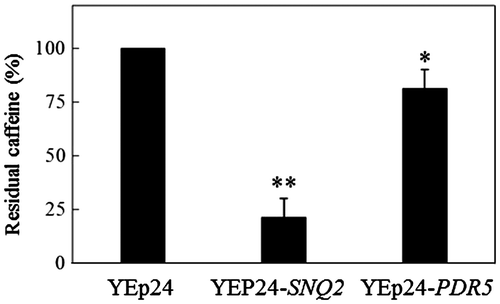
When cells were exposed to caffeine in the absence of glucose, there was no difference in intracellular caffeine contents (data not shown), suggesting Snq2p and Pdr5p would not affect caffeine transport by simple diffusion.
Substrate specificity of Snq2p and Pdr5p
In addition to caffeine, three chemicals (4-NQO, CTZ, and R6G) were used in drug resistance assays to investigate the substrate specificity of Snq2p and Pdr5p. The chemicals used in this study are structurally and functionally unrelated. 4-NQO is a quinoline derivative and is known to be a substrate of Snq2p.Citation11,12) CTZ is an azole antifungal agent and is exported by Pdr5p using an ATP energy.Citation16,23) R6G is a derivative of xanthene which is widely used as a substrate for transporter assay, and is also exported by Pdr5p in an energy-dependent manner.Citation16,22–24)
Multicopy of SNQ2 yielded significant resistance to R6G and 4-NQO, but not to CTZ (Fig. ). On the other hands, multicopy of PDR5 yielded significant resistance to R6G and CTZ, but not to 4-NQO (Fig. ). The results of resistance to 4-NQO and CTZ were consistent with the previous reports.Citation12,16,22–24) Thus, the two ABC pumps can share the same substrate (caffeine and R6G), and each of the pumps has a specific substrate (4-NQO or CTZ). These results indicate that SNQ2 and PDR5 will exhibit complementary function on resistance to R6G as well as caffeine, but not to 4-NQO and CTZ.
R6G-efflux activity in intact whole cells
Fig. indicates that Snq2p as well as Pdr5p export R6G. We evaluated the R6G-efflux activity of Snq2p or Pdr5p in intact KY25 cells harboring the corresponding plasmids (YEp24-SNQ2 or YEp24-PDR5) in the presence of glucose (an energy source) or 2-deoxyglucose (an inhibitor of glycolysis) using the modifying method of Tsujimoto et al.Citation16) Increase of R6G efflux in the presence of 2-deoxyglucose was due to simple diffusion, and its increase in the presence of glucose was due to an energy-dependent efflux by ABC transporters plus simple diffusion. In KY25 cells harboring YEp24 (control), there was no difference between the increases of R6G intensity in the presence of glucose and 2-deoxyglucose (Fig. (A)), suggesting the other ABC transporters (not Snq2p and Pdr5p) did not affect the R6G efflux, because KY25 (snq2Δ::kanMX4 pdr5Δ::CgHIS3) cells were used in this assay. Fig. showed that the rate of simple diffusion was similar in all three cell types, suggesting Snq2p and Pdr5p did not affect simple diffusion of R6G. KY25 cells harboring YEp24-SNQ2 or YEp24-PDR5 (SNQ2- or PDR5-overexpressing cells) exported R6G in the presence of glucose as an energy, and the activity of the former was less than half of that of the latter (Fig. ). These results are consistent with the R6G resistance test described above (Fig. ) and show that Snq2p is less effective against R6G than Pdr5p.
Fig. 4. R6G transport activity and inhibition by caffeine.
Notes: KY25 harboring YEp24 (A) YEp24-SNQ2 (B) or YEp24-PDR5 (C) were cultured, harvested, and exposed to R6G as described in the Materials and Methods. Cells were incubated in 50 mM HEPES (pH 6.0) buffer containing the following chemicals: ○, 5 mM 2-deoxyglucose; ●, 5 mM glucose; △, 5 mM glucose and 2 mM caffeine; ▲, 5 mM glucose and 5 mM caffeine; □, 5 mM glucose and 10 mM caffeine; and ■, 5 mM glucose and 21 mM caffeine. R6G fluorescence (excitation wavelength, 529 nm; emission wavelength, 553 nm) was sequentially monitored at 30 °C using a spectrofluorometer.
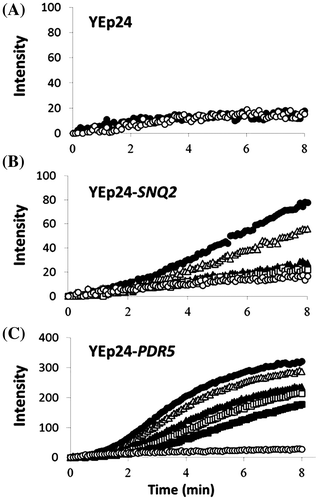
Caffeine inhibited R6G export of Snq2p and Pdr5p in a concentration-dependent manner (Fig. ). In KY25 cells harboring YEp24-SNQ2, 2 mM caffeine significantly inhibited the activity, while more than 5 mM caffeine nearly abolished the activity (Fig. (B)). In KY25 cells harboring YEp24-PDR5, 21 mM caffeine did not completely inhibit R6G-efflux activity. To determine the affinity for caffeine, IC50 values were calculated using the activity: the IC50 values of caffeine were 5.3 ± 1.9 mM (YEp24-SNQ2) and 17.2 ± 9.6 mM (YEp24-PDR5). Thus, Snq2p may have a higher affinity for caffeine than Pdr5p, consistent with the spot results indicating caffeine resistance (Fig. ) and caffeine residual contents (Fig. ). In order to compare their affinities for caffeine, it will be essential to know their kinetic parameters and the inhibitory mechanisms using plasma membranes.
Discussion
In this study, SNQ2 was identified as a caffeine-resistance gene in a multicopy genomic library screen. PDR5, a homolog of SNQ2, was also involved in caffeine resistance. To our knowledge, this is the first report that SNQ2 and PDR5 are ABC transporter genes involved in caffeine resistance. The gene name of SNQ2 was originally derived from its mutant phenotype: the null mutant exhibited sensitivity to 4-nitroquinoline-1-oxide (4-NQO, a quinoline derivative).Citation11) SNQ2 overexpression conferred hyper-resistance to triaziquone, 1-methyl-3-nitro-1-nitrosoguanidine, and other chemicals.Citation11,12) Snq2p is functionally homologous to Pdr5p, which is also a plasma membrane ABC transporter involved in cellular detoxification.Citation6,7,13) Pdr5p is one of the best characterized and most abundant ABC transporters in yeast and is known to export various chemicals such as R6G and CTZ.Citation16,22–24) In vivo, Snq2p and Pdr5p mediate transport of estradiol (a steroid hormone)Citation21) and 3,3′-dipropylthiacarbocyanine iodide [diS-C3(3), a fluorescent probe].Citation25) Recent attention has been focused on the roles of ABC transporters in tolerance of organic solvents. Cells expressing Snq2p or Pdr5p exhibit improved tolerance to biofuel alkanes; intracellular C10 alkane was reduced by 25 and 33% in cells expressing either Snq2p or Pdr5p after 24 h exposure.Citation26) Overexpression of Snq2p facilitated tolerance to dimethyl sulfoxide, a hydrophilic organic solvent.Citation27) Our results showed that multicopies of SNQ2 or PDR5 yielded significant resistance to caffeine, R6G and 4-NQO, or caffeine, R6G and CTZ, respectively (Figs. and ). Thus, their observations indicate that Snq2p and Pdr5p share common substrates, but also have specificities. The relationships between Pdr5p structure and activity have been extensively studied,Citation20,28–32) but the structure–function relationships of Snq2p have not been reported. Therefore, it is interesting and important to compare Snq2p and Pdr5p for improving our understanding of ABC transporters.
Single knockout of SNQ2 or PDR5 did not lead to caffeine sensitivity, although snq2Δ pdr5Δ double knockout led to significant sensitivity (Fig. (B)). These results indicated that Snq2p and Pdr5p cooperate in a caffeine-resistance strategy. One of the two transporters is sufficient to confer caffeine resistance in yeast, and their roles are complementary. R6G is a substrate of Pdr5pCitation16,21–24) and is a mitochondrial ATP synthase inhibitor.Citation33,34) However, it was reported that R6G was not a substrate of Snq2p based on drug sensitivity testing in single snq2Δ knockout cells, while pdr5Δ single knockout and snq2Δ pdr5Δ double knockout cells were sensitive to R6G.Citation22) Elevated expression of PDR5 in the absence of SNQ2 would lead to an increase of drug-efflux activity, so knockout of SNQ2 would not cause sensitivity to R6G. Interestingly, DS2 (snq2Δ::kanMX4) cells were slightly stronger in CTZ-containing medium, and DY5 (pdr5Δ::kanMX4) and DY5c (pdr5Δ::CgHIS3) cells were slightly stronger in 4-NQO-containing medium in comparison to wild-type cells (data not shown). They will be due to the fact that inactivation of SNQ2 or PDR5 affects the transcription of the remaining homologs and induces that of their own promoters.Citation35) These observations show that SNQ2 and PDR5 serve complementary functions in resistance to caffeine and R6G.
After exposure to caffeine, caffeine was extracted and analyzed to show that Snq2p and Pdr5p exported caffeine. As a result, the ratios of caffeine contents were 21.3 ± 8.8% (YEp24-SNQ2) and 81.9 ± 8.7% (YEp24-PDR5) relative to control (YEp24, 100%), and Snq2p was a more functional transporter of caffeine than Pdr5p (Fig. ). These results were consistent with the spot analysis (Fig. ). However, when cells were incubated in glucose-containing buffer without caffeine and nutrients (not in YPD medium) after the initial caffeine exposure, no differences were observed in caffeine residual contents of three cell types (KY25 cells harboring YEp24, YEp24-SNQ2, or YEP24-PDR5) (data not shown). These results suggested that multicopies of SNQ2 or PDR5 did not affect the intake and metabolism of caffeine and that the exported caffeine was associated with hydrophobic substances in the medium to promote the efflux of caffeine. Accepter proteins such as serum albumin, which has hydrophobic regions and can bind lipids, can bind a variety of transporter substrates in higher animals.Citation36) If there were no hydrophobic acceptor in extracellular medium, the exported caffeine would associate with the cell surface or penetrate into the cell. In the R6G-transport assay, such acceptors are not needed, because cell surface-associated and extracellular dyes can be measured. Hydrophobic and amphiphilic substances exported by transporters require an accepter to inhibit reabsorption into the cell.
To estimate the affinity for caffeine, we used a fluorescent dye as a transporter substrate, because it allows a convenient measurement of time-dependent changes. Thus, we tried to detect the export activity of R6G using KY25 cells (snq2Δ::kanMX4 pdr5Δ::CgHIS3) harboring plasmids, because the complementary effect of Snq2p and Pdr5p can be negligible in KY25 cells. R6G was exported in KY25 cells harboring YEp24-SNQ2 or YEp24-PDR5 (SNQ2- or PDR5-overexpressing cells) in an energy-dependent manner (Fig. ). Rhodamine 123, rhodamine B, and fluorescein were also used in transport assay (data not shown). Rhodamine 123 was exported in low amounts from PDR5-overexpressing cells in the presence of glucose, but not from SNQ2-overexpressing cells (data not shown). Rhodamine B and fluorescein were not substrates of Snq2p and Pdr5p (data not shown). Therefore, R6G would be an effective substrate for transport assays of Snq2p and Pdr5p. Using cells overexpressing SNQ2 or PDR5, R6G-efflux activity was measured. As a result, Snq2p was a less effective R6G transporter than Pdr5p (Figs. 3 and 4). In addition, caffeine inhibited the R6G-efflux activities of Snq2p and Pdr5p with half-maximal inhibitory concentrations of 5.3 ± 1.9 (YEp24-SNQ2) and 17.2 ± 9.6 mM (YEp24-PDR5). (Fig. ). The inhibition analysis indicated that Snq2p may have a higher affinity for caffeine than Pdr5p (Fig. ), consistent with the spot and TLC analyses (Figs. and ). However, their kinetic parameters in caffeine-efflux and modes of inhibition of R6G-efflux by caffeine were not determined. Therefore, further analysis is needed to understand their molecular mechanisms of action.
Here, we have demonstrated that Snq2p and Pdr5p mediate efflux of caffeine and R6G. Multicopy of SNQ2 led to more effective caffeine resistance and efflux than that of PDR5 (Figs. , and ). However, it may be difficult to conclude that Snq2p is a more functional transporter of caffeine than Pdr5p, because their protein contents may be significantly different in yeast cells. Plasma membranes were prepared from KY25 cells harboring YEp24, YEp24-SNQ2, or YEp24-PDR5 according to Ernst et al.Citation20) Specific bands on sodium dodecyl sulfate polyacylamide gel electrophoresis (SDS-PAGE) gels could be assigned to Snq2p and Pdr5p, but were absent in control membranes (YEp24). These were confirmed by tandem mass spectrometry analysis. Densitometric evaluation of protein bands was performed with NIH Image J software. The analysis showed that ratios of Snq2p and Pdr5p contents were 0.45 ± 0.16 and 0.70 ± 0.14, respectively, relative to Pma1p, a plasma membrane H+-ATPase (preliminary data). These results indicated that the content of Snq2p was similar or less than that of Pdr5p in plasma membranes. Therefore, we concluded that Snq2p is a more functional transporter of caffeine than Pdr5p. Very recently, it was reported that human ABCG2, a member of the ABCG subfamily including Snq2p and Pdr5p, was identified as one of six genes potentially involved in pharmacokinetics and pharmacodynamics of caffeine.Citation37) Therefore, ABCG members will gain more attention in the control of intracellular concentration of caffeine.
Acknowledgments
We are thankful to the National BioResource Project in Japan (http://www.nbrp.jp/) for providing the yeast strains. We express our gratitude to Dr. Tohru Saeki of Kyoto Prefectural University for his generous advice.
References
- Dews PB. Caffeine. Annu. Rev. Nutr. 1982;2:323–341.10.1146/annurev.nu.02.070182.001543
- Dews PB, O’Brien CP, Bergman J. Caffeine: behavioral effects of withdrawal and related issues. Food Chem. Toxicol. 2002;40:1257–1261.10.1016/S0278-6915(02)00095-9
- Ferguson LR, Philpott M. Nutrition and mutagenesis. Annu. Rev. Nutr. 2008;28:313–329.10.1146/annurev.nutr.28.061807.155449
- Zhou H, Luo Y, Huang S. Updates of mTOR inhibitors. Anticancer Agents Med. Chem. 2010;10:571–81.10.2174/187152010793498663
- Rockenfeller P1, Madeo F. Ageing and eating. Biochim. Biophys. Acta. 2010;1803(4):499–506.10.1016/j.bbamcr.2010.01.001
- Paumi CM, Chuk M, Snider J, Stagljar I, Michaelis S. ABC transporters in Saccharomyces cerevisiae and their interactors: New technology advances the biology of the ABCC (MRP) subfamily. Microbiol. Mol. Biol. Rev. 2009;73:577–593.10.1128/MMBR.00020-09
- Prasad R, Goffeau A. Yeast ATP-binding cassette transporters conferring multidrug resistance. Annu. Rev. Microbiol. 2012;66:39–63.10.1146/annurev-micro-092611-150111
- Nakajima T, Aoyama T. Polymorphism of drug-metabolizing enzymes in relation to individual susceptibility to industrial chemicals. Ind. Health. 2000;38(2):143–152.10.2486/indhealth.38.143
- Morel M1, Meux E, Mathieu Y, Thuillier A, Chibani K, Harvengt L, Jacquot JP, Gelhaye E. Xenomic networks variability and adaptation traits in wood decaying fungi. Microbiol. Biotechnol. 2013;6:248–263.10.1111/1751-7915.12015
- Kot M1, Daniel WA. Caffeine as a marker substrate for testing cytochrome P450 activity in human and rat. Pharmacol. Rep. 2008;60:789–797.
- Haase E, Servos J, Brendel M. Isolation and characterization of additional genes influencing resistance to various mutagens in the yeast Saccharomyces cerevisiae. Curr. Genet. 1992;21:319–324.10.1007/BF00351689
- Servos J, Haase E, Brendel M. Gene SNQ2 of Saccharomyces cerevisiae, which confers resistance to 4-nitroquinoline-N-oxide and other chemicals, encodes a 169 kDa protein homologous to ATP-dependent permeases. Mol. Gen. Genet. 1993;236:214–218.10.1007/BF00277115
- Leppert G1, McDevitt R, Falco SC, Van Dyk TK, Ficke MB, Golin J. Cloning by gene amplification of two loci conferring multiple drug resistance in Saccharomyces. Genetics. 1990;125:13–20.
- Mortimer RK, Johnston JR. Genealogy of principal strains of the yeast genetic stock center. Genetics. 1986;113:35–43.
- Sikorski RS, Hieter P. A system of shuttle vectors and yeast host strains designed for efficient manipulation of DNA in Saccharomyces cerevisiae. Genetics. 1989;122:19–27.
- Tsujimoto Y, Takase D, Okano H, Tomari N, Watanabe K, Matsui H. Functional roles of YPT31 and YPT32 in clotrimazole resistance of Saccharomyces cerevisiae through effects on vacuoles and ATP-binding cassette transporter(s). J. Biosci. Bioeng. 2013;115:4–11.10.1016/j.jbiosc.2012.08.011
- Sambrook J, Fritsch EF, Maniatis T. Molecular cloning: a laboratory manual. 2nd ed. Cold Spring Harbor (NY): Cold Spring Harbor Laboratory Press; 1989.
- Burke D, Dawson D, Stearns T. Methods in yeast genetics: a Cold Spring Harbor Laboratory course manual. Cold Spring Harbor (NY): Cold Spring Harbor Laboratory Press; 2000.
- Sanger F. Determination of nucleotide sequences in DNA. Science. 1981;214:1205–1210.10.1126/science.7302589
- Ernst R, Kueppers P, Klein CM, Schwarzmueller T, Kuchler K, Schmitt L. A mutation of the H-loop selectively affects rhodamine transport by the yeast multidrug ABC transporter Pdr5. Proc. Natl. Acad. Sci. USA. 2008;105:5069–5074.10.1073/pnas.0800191105
- Mahé Y, Lemoine Y, Kuchler K. The ATP binding cassette transporters Pdr5 and Snq2 of Saccharomyces cerevisiae can mediate transport of steroids in Vivo. J. Biol. Chem. 1996;271:25167–25172.10.1074/jbc.271.41.25167
- Rogers B, Decottignies A, Kolaczkowski M, Carvajal E, Balzi E, Goffeau A. The pleitropic drug ABC transporters from Saccharomyces cerevisiae. J. Mol. Microbiol. Biotechnol. 2001;3:207–214.
- Golin J, Kon ZN, Wu C-P, Martello J, Hanson L, Supernavage S, Ambudkar SV, Sauna ZE. Complete inhibition of the Pdr5p multidrug efflux pump ATPase activity by its transport substrate clotrimazole suggests that GTP as well as ATP may be used as an energy source. Biochemistry. 2007;46:13109–13119.10.1021/bi701414f
- Kolaczkowski M, van der Rest M, Cybularz-Kolaczkowska A, Soumillion JP, Konings WN, Goffeau A. Anticancer drugs, ionophoric peptides, and steroids as substrates of the yeast multidrug transporter pdr5p. J Biol. Chem. 1996;271:31543–31548.10.1074/jbc.271.49.31543
- Hendrych T, Kodedová M, Sigler K, Gásková D. Characterization of the kinetics and mechanisms of inhibition of drugs interacting with the S. cerevisiae multidrug resistance pumps Pdr5p and Snq2p. Biochim. Biophys. Acta. 2009;1788:717–723.10.1016/j.bbamem.2008.12.001
- Ling H, Chen B, Kang A, Lee JM, Chang MW. Transcriptome response to alkane biofuels in Saccharomyces cerevisiae: Identification of efflux pumps involved in alkane tolerance. Biotechnol. Biofuels. 2013;6:95. doi: 10.1186/1754-6834-6-95.10.1186/1754-6834-6-95
- Nishida N, Ozato N, Matsui K, Kuroda K, Ueda M. ABC transporters and cell wall proteins involved in organic solvent tolerance in Saccharomyces cerevisiae. J. Biotechnol. 2013;165:145–152.10.1016/j.jbiotec.2013.03.003
- Furman C, Mehla J, Ananthaswamy N, Arya N, Kulesh B, Kovach I, Ambudkar SV, Golin J. The deviant ATP-binding site of the multidrug efflux pump Pdr5 plays an active role in the transport cycle. J. Biol. Chem. 2013;288:30420–30431.10.1074/jbc.M113.494682
- Downes MT, Mehla J, Ananthaswamy N, Wakschlag A, Lamonde M, Dine E, Ambudkar SV, Golin J. The transmission interface of the Saccharomyces cerevisiae multidrug transporter Pdr5: Val-656 located in intracellular loop 2 plays a major role in drug resistance. Antimicrob. Agents Chemother. 2013;57:1025–1034.10.1128/AAC.02133-12
- Kueppers P, Gupta RP, Stindt J, Smits SH, Schmitt L. Functional impact of a single mutation within the transmembrane domain of the multidrug ABC transporter Pdr5. Biochemistry. 2013;52:2184–2195.10.1021/bi3015778
- Chen Z, Li J, Wang W, Guo X, Li Y, Mao X, Chen X, Guan W. Mutations adjacent to the end of transmembrane helices 6 and 7 independently affect drug efflux capacity of yeast ABC transporter Pdr5p. Biochim. Biophys. Acta. 2014;1838:932–939.10.1016/j.bbamem.2013.12.002
- Gupta RP, Kueppers P, Hanekop N, Schmitt L. Generating symmetry in the asymmetric ATP-binding cassette (ABC) transporter Pdr5 from Saccharomyces cerevisiae. J. Biol. Chem. 2014;289:15272–15279.10.1074/jbc.M114.553065
- Wieker HJ, Kuschmitz D, Hess B. Inhibition of yeast mitochondrial F1-ATPase, F0F1-ATPase and submitochondrial particles by rhodamines and ethidium bromide. Biochim. Biophys. Acta. 1987;892:108–117.10.1016/0005-2728(87)90253-2
- Bullough DA, Ceccarelli EA, Roise D, Allison WS. Inhibition of the bovine-heart mitochondrial F1-ATPase by cationic dyes and amphipathic peptides. Biochim. Biophys. Acta. 1989;975:377–383.10.1016/S0005-2728(89)80346-9
- Kolaczkowska A, Kolaczkowski M, Goffeau A, Moye-Rowley WS. Compensatory activation of the multidrug transporters Pdr5p, Snq2p, and Yor1p by Pdr1p in Saccharomyces cerevisiae. FEBS Lett. 2008;582:977–983.10.1016/j.febslet.2008.02.045
- Stein WD. Kinetics of the multidrug transporter (P-glycoprotein) and its reversal. Physiol Rev. 1997;77:545–590.
- Paynter N, Monda KL, Amin N, Fischer K, Renstrom F, Ngwa JS, Huikari V, Cavadino A, Nolte IM, Teumer A, Yu K, Marques-Vidal P, Rawal R, Manichaikul A, Wojczynski MK, Vink JM, Zhao JH, Burlutsky G, Lahti J, Mikkilä V, Lemaitre RN, Eriksson J, Musani SK, Tanaka T, Geller F, Luan J, Hui J, Mägi R, Dimitriou M, Garcia ME, Ho WK, Wright MJ, Rose LM, Magnusson PK, Pedersen NL, Couper D, Oostra BA, Hofman A, Ikram MA, Tiemeier HW, Uitterlinden AG, van Rooij FJ, Barroso I, Johansson I, Xue L, Kaakinen M, Milani L, Power C, Snieder H, Stolk RP, Baumeister SE, Biffar R, Gu F, Bastardot F, Kutalik Z, Jacobs DR Jr, Forouhi NG, Mihailov E, Lind L, Lindgren C, Michaëlsson K, Morris A, Jensen M, Khaw KT, Luben RN, Wang JJ, Männistö S, Perälä MM, Kähönen M, Lehtimäki T, Viikari J, Mozaffarian D, Mukamal K, Psaty BM, Döring A, Heath AC, Montgomery GW, Dahmen N, Carithers T, Tucker KL, Ferrucci L, Boyd HA, Melbye M, Treur JL, Mellström D, Hottenga JJ, Prokopenko I, Tönjes A, Deloukas P, Kanoni S, Lorentzon M, Houston DK, Liu Y, Danesh J, Rasheed A, Mason MA, Zonderman AB, Franke L, Kristal BS; International parkinson’s disease genomics consortium (IPDGC); North American Brain Expression Consortium (NABEC); UK Brain Expression Consortium (UKBEC), Karjalainen J, Reed DR, Westra HJ, Evans MK, Saleheen D, Harris TB, Dedoussis G, Curhan G, Stumvoll M, Beilby J, Pasquale LR, Feenstra B, Bandinelli S, Ordovas JM, Chan AT, Peters U, Ohlsson C, Gieger C, Martin NG, Waldenberger M, Siscovick DS, Raitakari O, Eriksson JG, Mitchell P, Hunter DJ, Kraft P, Rimm EB, Boomsma DI, Borecki IB, Loos RJ, Wareham NJ, Vollenweider P, Caporaso N, Grabe HJ, Neuhouser ML, Wolffenbuttel BH, Hu FB, Hyppönen E, Järvelin MR, Cupples LA, Franks PW, Ridker PM, van Duijn CM, Heiss G, Metspalu A, North KE, Ingelsson E, Nettleton JA, van Dam RM, Chasman DI. Genome-wide meta-analysis identifies six novel loci associated with habitual coffee consumption. Mol Psychiatry. Forthcoming. doi:10.1038/mp.2014.107.