Abstract
Activating transcription factor 5 (ATF5) is a stress response transcription factor of the cAMP-responsive element-binding/ATF family. Earlier, we reported that ATF5 expression is up-regulated in response to stress, such as amino acid limitation or arsenite exposure. Although ATF5 is widely expressed in the brain and the olfactory epithelium, the role of ATF5 is not fully understood. Here, the olfactory bulbs (OBs) of ATF5-deficient mice are smaller than those of wild-type mice. Histological analysis reveals the disturbed laminar structure of the OB, showing the thinner olfactory nerve layer, and a reduced number of interneurons. This is mainly due to the reduced number of bromodeoxyuridine-positive proliferating cells in the subventricular zone, where the interneuron progenitors are formed and migrate to the OBs. Moreover, the olfaction-related aggressive behavior of ATF5-deficient mice is reduced compared to wild-type mice. Our data suggest that ATF5 plays a crucial role in mouse OB development via interneuron.
Graphical abstract
ATF5-deficient mice (ATF5-/-) have smaller olfactory bulbs (OBs) showing the thinner olfactory nerve layer and a reduced number of interneurons.
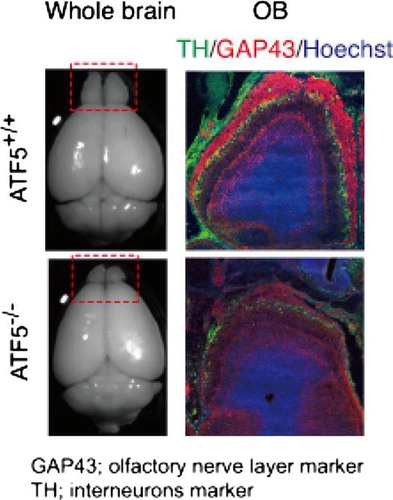
The olfactory bulb (OB) is a structure of the forebrain involved in olfactory function. The OB, which is more developed in the mouse than in the human, consists of 2 parts: the main olfactory bulb (MOB) and the accessory olfactory bulb (AOB). The MOB is involved in the recognition of chemical odor cues detected by the main olfactory epithelium (MOE). The AOB is involved in the response to pheromone cues detected by the vomeronasal organ (VNO).
The MOB has a laminar structure and transmits odor information from the MOE in the nasal cavity to the brain.Citation1) The outermost section of the OB is the olfactory nerve layer (ONL), which consists of olfactory axons from the olfactory epithelium (OE). The glomerulus exists in the glomerular layer (GL), the second layer of the OB, where olfactory nerve axons form synapses with dendrites of 2 types of projection neurons (mitral cells and tufted cells) in the glomerulus. The mitral and tufted cells form the mitral cell layer (MCL) and the external plexiform layer, respectively. Periglomerular cells and granule cells are interneurons in the GL and granule cell layer (GCL), respectively. Periglomerular cells form synapses between the glomeruli and the granule cells form synapses with mitral cells. Projection neurons and interneurons of the OB are produced in different ways.Citation1,2) Projection neurons arise from telencephalic progenitors during embryonic day 11 (E11)–E13 in mice. At E14–E18, the interneurons are formed from the lateral ganglionic eminences of the embryonic telencephalon, which form the subventricular zone (SVZ), and migrate to the OB along the rostral migratory stream (RMS).
Clinical studies have revealed that small OB size reflects olfactory dysfunction in patients who are suffering from congenital anosmia, neurodegenerative disorder, psychiatric disorder, post-infectious and olfactory deficits, etc.Citation3−8) Interestingly, olfactory dysfunction may also be induced by chemical, biological, and mental stress.Citation9,10) However, the molecular pathogenesis of these OB size-related defects is incompletely understood.
Activating transcription factor 5 (ATF5), a member of the ATF/cAMP-responsive element-binding transcription factor family, is reported to regulate cell differentiation, survival, and apoptosis.Citation11−17) ATF5 is widely expressed in the brain, including the ventricular zone (VZ) and the SVZ, where neurogenesis occurs, and is highly expressed in the OE.Citation12,18−20) Recently, Wang et al. have reported that ATF5 expressed in olfactory sensory neurons (OSNs), was required for terminal differentiation and survival of OSNs.Citation19)
We and others have demonstrated that ATF5 expression is up-regulated at the translational step when cells respond to stress, including amino acid limitation, arsenite exposure, endoplasmic reticulum (ER) stress, and heat shock.Citation21−24) Translational up-regulation of ATF5 under stress condition is induced by phosphorylation of eukaryotic initiation factor 2α (eIF2α), although global translation is down-regulated.Citation23) In the process of olfactory sensory neuron maturation, ER burden of odorant receptor, a membrane protein, induces ER stress, followed by eIF2α phosphorylation by PERK. This stress response leads to ATF5 gene expression, resulting in acceleration of maturation of OSNs.Citation20) Thus, under the intrinsic stress condition, ATF5 is important for normal cell differentiation and survival of OSNs in the OE. However, it remains to be determined whether ATF5 regulates the formation of OB, to which axons of OSNs form ONL and SVZ-born interneurons migrate.
In this study, ATF5-deficient (ATF5–/–) mice were generated to identify roles of ATF5 in the OB development. Seventy percent of ATF5–/– mice die within 3 days after birth. Anatomic analysis revealed that the OBs of ATF5–/– mice are smaller than those of ATF5+/– mice and have an irregular laminar structure suggesting that ATF5 is required for OB development.
Materials and methods
Generation of ATF5-deficient mice
Basic methods for the establishment of mice with targeted gene disruption are described elsewhere.Citation25) A targeting vector was constructed using pMulti-ND 1.0 containing the neoresistance gene (neor) as a positive selection marker, and diphtheria toxin A-chain (DT) as a negative selection marker. A 2.4 kb KpnI–KpnI fragment and a 6.2 kb XhoI–XhoI fragment were inserted as a short arm and a long arm, respectively. Embryonic stem cells derived from 129/Sv (D3) were electroporated with PmeI-digested linearized DNA. Of 385 G418-resistant clones, four had undergone homologous recombination correctly. Three targeted cell lines were injected into C57BL/6 blastocysts, resulting in the birth of male chimeric mice that were crossed with C57BL/6 to obtain heterozygous mutants. Congenic mice used in this study were the offspring of crosses for >25 generations. Pairs of PCR primers were used for genotyping ATF5 knockout mice.
For the wild-type allele:
smgATF5-s3 5′-CAGTACGTGAAGGACCTGCTAATTGAGGTG-3′
smgATF5-as5 5′-AGTGGCTTCCTGCTTTATGTTCATGCCAGG-3′
For the mutant allele:
smKOa-s1 5′-GTCACACTTAATTAAGGCCGCAATGGCCAG-3′
smKOa-as1 5′-CATGTTCTTGGGTCGACGGTATCGATAAGC-3′
Thirty cycles of PCR (96 °C for 10 s, 58 °C for 30 s, and 68 °C for 1 min) were done with KOD FX DNA polymerase (TOYOBO, Osaka, Japan). Neonatal mice delivered via cesarean section were fed ~20 mg of milk every 3 h. Hand-feeding of formula milk with a nursing bottle of Hoshiba Nipple-Yajima Style (provided by Meiji Dairies Corporation) was done as described.Citation26,27)
Histology and immunohistochemistry
Immunohistochemistry was done essentially as described.Citation28) In brief, coronal, sagittal, and horizontal sections 20 or 50 μm in thickness were cut with a cryostat. Non-specific binding sites were blocked with PBS containing 0.1% (v/v) Tween 20 and 2% (v/v) skim milk; then, the specimens were incubated with primary antibodies at 4 °C overnight, washed, and then incubated with Alexa594- or Alexa488-labeled secondary antibodies (Molecular Probes, Eugene, OR). The primary antibodies were used as follows: Tbx21 (guinea pig, 1:5000; provided by Dr. YoshiharaCitation29)); Reelin (rabbit, 1:50, Santa Cruz Biotechnology Inc., Santa Cruz, CA); GABA (rabbit, 1:2000; Sigma–Aldrich); NCAM (rat, 1:250; Millipore, Billerica, MA); tyrosine hydroxylase (TH) (mouse, 1:400; Millipore); GAP43 (rabbit, 1:50, R&D Systems, Minneapolis, MN); bromodeoxyuridine (rat, 1:200; AbD Serotec, Oxford, UK); and cleaved caspase3 (rabbit, 1:200, Cell Signaling, Danvers, MA). Hoechst 33258 was obtained from Nacalai Tesque (Osaka, Japan). Images of immunofluorescence-labeled sections were obtained with a fluorescence microscope (KEYENCE BZ-9000, Osaka, Japan) or a confocal microscope (Olympus FV1000, Tokyo, Japan).
Labeling with bromodeoxyuridine (BrdU)
Pregnant mice were injected intraperitoneally with BrdU (200 mg/kg of body weight) and sacrificed after 3 h.Citation29,30) For proliferation analysis, 20 μm thick, serial, coronal sections were prepared from E14 mice. Sections were treated with 2 M HCl at 40 °C for 20 min and neutralized with 40 mM sodium borate buffer (pH 8.5) before staining with anti-BrdU antibody.
Nissl staining
Nissl staining was done as described by Long et al.Citation31) Sections on slides were soaked in 0.1% (w/v) cresyl violet for 30 min; dehydrated by passage through a series of ethanol (70, 80, and 100%, v/v) and then 100% (v/v) xylene; and mounted. The images were analyzed by light microscopy using KEYENCE BZ-9000.
Preparation of cell lysate and immunoblotting
The OB was homogenized and protein was extracted in RIPA buffer (25 mM Tris–HCl, pH 7.6, 150 mM NaCl, 1% (w/v) NP-40, 1% (w/v) sodium deoxycholate, and 0.1% (w/v) SDS) with CompleteTM protease inhibitors (Roche Diagnostics, Indianapolis, IN, USA) as described.Citation24) Lysates were centrifuged to clear debris. Protein concentration was determined using the BCA Protein Assay kit (Thermo Scientific, Waltham, MA). Proteins (10 μg per sample) were separated by SDS–PAGE (10% (w/v) polyacrylamide gel) and transferred to a PVDF membrane (Millipore). The membranes were incubated with anti-TH antibody or anti-β-actin antibody.
Resident–intruder test
The resident–intruder test was done as described.Citation32−35) To test the aggressiveness, a male mouse was kept in a cage with a female mouse for 1 week for territory to be established. When testing, the female mouse was removed and an unfamiliar male wild-type mouse was put into the cage with the resident mouse. The number of attacks made by the resident mouse against the intruder mouse during 15 min was recorded.
Statistical analysis
All quantitative data are given as mean ± S.E.M. All statistical analysis was done with the Student’s t-test after the analysis of variance. The significant level of the statistical difference was set at p ≤ 0.05.
Results
Generation of ATF5-deficient mice
ATF5-deficient mice were generated by homologous recombination to address the physiological role of ATF5. A targeting construct of ATF5 was designed to replace exon 3 with a neomycin-resistant gene (neor), which was deleted by cre-loxP recombination (Fig. S1(A)). The genotype of ATF5 in the wild type (ATF5+/+), heterozygote (ATF5+/–), and homozygote (ATF5–/–) was confirmed by genomic PCR analysis, and the expression of ATF5 mRNA was done by Northern blot analysis (Fig. S1(B) and (C)). Female ATF5+/– mice were backcrossed with the male of C57BL/6 genetic background for >19 generations. About two thirds of congenic ATF5–/– mice die within 3 days after birth (Fig. ). ATF5+/– and ATF5+/+ mice are viable and grow to adulthood normally as judged by external macroscopic observations. We asked whether neonatal death of ATF5–/– mice is caused by the inability of pups to suckle. To test this, we investigated the survival rate of ATF5–/– mice, hand-fed formula milk using a nursing bottle compared to hand-fed ATF5+/+ and ATF5+/– mice. The neonatal lethality of ATF5–/– mice was rescued by hand-feeding (Fig. S2). The inability of ATF5–/– pups to suckle might be responsible for the neonatal lethality of ATF5–/– mice, at least in part. Surviving adult ATF5–/– mice of about 12 weeks of age were significantly lighter, 8.97% in body weight, than ATF5+/+ mice (data not shown) consistent with the previous report.Citation19) We could not find additional macroscopic features in ATF5–/– mice.
Abnormal morphology of the olfactory bulb in ATF5–/– mice
Next, we speculated ATF5–/– pups died because they could not communicate with the mother or could not find her teat. Abnormal behavior is caused by defective brain function, at least in part; therefore, we attempted to identify ATF5 physiological function in vivo, especially in the brain. Anatomical analysis of the brain demonstrated that the tissue weight of the OB is reduced in the adult ATF5–/– mice compared to their ATF5+/– littermates (Fig. (A)–(C)). Neonatal ATF5–/– mice have smaller OBs compared to ATF5+/+ littermates (Fig. S3(A)–(C)) suggesting that ATF5 is important for OB development. ATF5 genotype had no effect on the brain weight except the OB (data not shown). The adult OB has a highly ordered laminar structure. The detailed structure of the OB was analyzed using Hoechst and Nissl-stained sections of ATF5+/+ and ATF5–/– mice (Fig. (D)–(G) and Fig. S4). Hoechst-stained sections of the OBs of 12 week old ATF5–/– mice have a laminar structure, but have an irregular GL (Fig. (D)–(G)). Nissl-stained sections show the OBs of 2 week old ATF5–/– mice that have an irregular GL and thinner ONL than those of ATF5+/+ mice (Fig. S4(A) and (B)). Neither typical GL nor MCL was observed in the OBs of postnatal day 0 (P0) ATF5–/– mice, whereas these structural layers were detected in P0 ATF5+/+ mice (Fig. S4(C) and (D)). These data suggest that ATF5 is important for the OB’s morphological development.
Fig. 2. Reduced size of the OB in ATF5–/– mice.
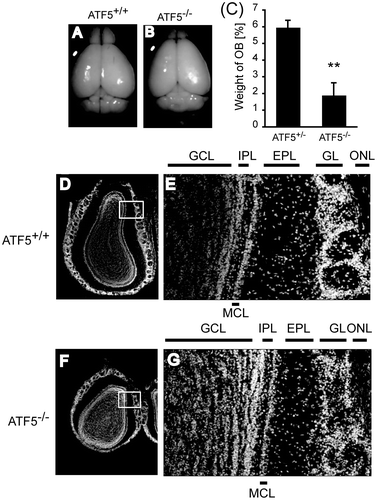
Disorganized projection neurons of the mitral cell layer in ATF5–/– mice
The projection neurons and interneurons of the OB were analyzed by using various immunohistochemical markers in order to examine the detailed laminar structure of the small OBs of ATF5–/– mice. Projection neurons in the MCL were visualized by using Tbx21 and Reelin antibodies. Tbx21 is detected in the nucleus of mitral cells in the OB and Reelin is expressed in the proximal dendrites of mitral cells.Citation29,31) The Tbx21- and Reelin-positive MCL of ATF5–/– mice was disorganized and thicker than that of ATF5+/+ mice (Fig. S5). The Reelin-positive dendrites of mitral cells are oriented in an apical direction in ATF5+/+ mice (Fig. S5(C)). By contrast, the orientation of the dendrites of mitral cells is disorganized in ATF5–/– mice (Fig. S5(F)). These results suggest that ATF5 is required for the formation of MCL of the OB.
Decrease in the interneurons in ATF5–/– mice
Interneurons in ATF5–/– mice were examined by immunohistology using GABA and TH antibodies. GABA is expressed in the GABAergic interneurons of granule cells in the GCL and in periglomerular cells in the GL. TH is expressed in the GABAergic and the dopaminergic periglomerular cells in the GL. GABA immunoreactivity is detected in the granule cells and periglomerular cells in the GCL and the GL in ATF5+/+ mice (Fig. (A) and (B)). However, the expression of GABA in the GCL and the GL of ATF5–/– mice is reduced at both E16 (Fig. (D)) and P0 (Fig. (E)) compared to ATF5+/+ mice. TH immunoreactivity is detected in the periglomerular cells in the GL of ATF5+/+ mice (Fig. (C)), but the TH immunoreactivity is expressed weakly and discontinuously in the GL of ATF5–/– mice (Fig. (F)). Western blotting shows a significant decrease in TH protein expression of the OBs of ATF5–/– mice (Fig. S6(A)). These results indicate the OBs of ATF5–/– mice have reduced TH- and GABA-positive cells suggesting that the interneurons are reduced in the OB of ATF5–/– mice.
Fig. 3. Sparse interneurons and impaired surrounding of the OB by ONL in ATF5–/– mice.
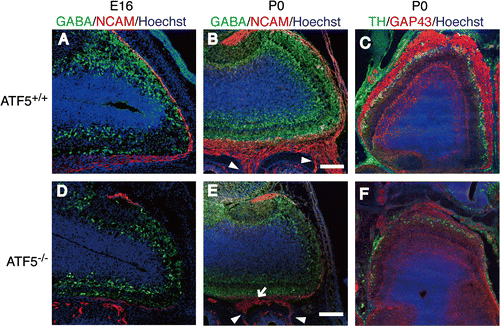
To assess whether the reduction of interneurons in the ATF5–/– OB is caused by reduced proliferation of interneuron progenitors in the OB and SVZ, we used a short pulse of BrdU at E14 (when the OB interneuron is formed in SVZ).Citation2,29) BrdU, a thymidine analog, is detected as the proliferation marker by immunohistochemistry, which is a common method for labeling cells in the S-phase of the cell cycle.Citation30,36,37) Three hours after injection of BrdU at E14, the BrdU-positive cells were analyzed in the SVZ and OB by immunohistology. The number of BrdU-positive cells in the ATF5–/– OB is reduced significantly compared to the ATF5+/+ OB (Fig. (A)–(C)). The number of BrdU-positive cells in the SVZ is also reduced significantly in the ATF5–/– mice (Fig. (D)–(F)).
Fig. 4. Reduced number of proliferating interneuron progenitors in ATF5–/– mice.
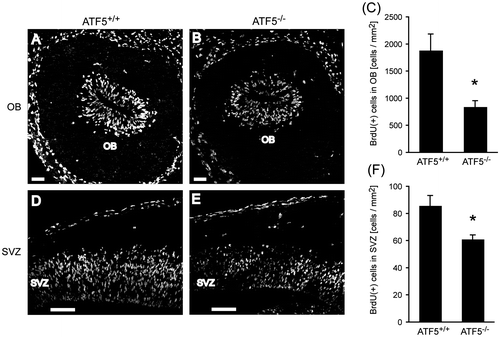
Next, to investigate whether the reduction of OB size in the ATF5–/– is caused by cell death in the OB, apoptotic cells were analyzed by immunohistology using cleaved caspase 3 antibodies. The number of cleaved caspase 3-positive cells in the OB of ATF5–/– mice is similar to that of ATF5+/+ mice (data not shown). These results indicate that the number of interneurons in GL and GCL of OBs and the proliferation of interneuron progenitors were reduced in ATF5–/– mice suggesting that ATF5 is important for interneuron regulation.
Defective formation of the olfactory nerve layer of the olfactory bulb in ATF5–/– mice
Nissl-stain analysis reveals the ONL in ATF5–/– mice are obviously thinner compared to ATF5+/+ mice (Fig. S4(A) and (B)). To confirm this, we analyzed the ONL by immunohistology using NCAM and GAP43 antibodies. At E16 and P0, the NCAM-positive ONL of ATF5+/+ mice surround the OB continuously (Fig. (A) and (B)). By contrast, the NCAM-positive ONL of ATF5–/– mice is thin and discontinuous (Fig. (D) and (E)). GAP43-positive ONL is also thin and does not surround the OB completely in ATF5–/– mice (Fig. (F)). Olfactory axons are projected to the OB from the OE in ATF5+/+ mice (Fig. (B), arrowheads). By contrast, ATF5–/– olfactory axons projected from the OE to the OB were severely limited and insufficient (Fig. (E), arrowheads). In particular, most olfactory axons sometimes form small tangled spheres between the OE and the OB (Fig. (E), arrow). At E18 and P0, overlapping area of NCAM-positive olfactory axons and TH-positive interneurons is wide in ATF5+/+ mice (Fig. S6(B) and (C)) indicating that the olfactory axons penetrate and interact sufficiently into the interneuron layer. However, the overlapping area is narrow in ATF5–/– mice showing that penetration of the olfactory axons into the interneuron layer is shallow in ATF5–/– mice (Fig. S6(D) and (E)). These results indicate that the ONL of OBs is thin and the axonal projection of olfactory neurons from the OE is defective in ATF5–/– mice. OB sections were stained with anti-cleaved caspase 3 at P0, as cleaved caspase 3 is the active form of caspase 3 to examine whether apoptosis occurs in the ONL of ATF5–/– mice OBs. Only a few cells expressing cleaved caspase 3 in the OE and NCAM-positive ONL are detected in ATF5+/+ mice (Fig. S7(A) and (B), arrowheads), but the expression of cleaved caspase 3 is abundant in the OE and NCAM-positive ONL of ATF5–/– mice (Fig. S7(C) and (D), arrowheads). These results indicate that apoptosis is inactive in OBs of both ATF5–/– and ATF5+/+ mice, but active in OSNs of ATF5–/– mice.
ATF5–/– mice are less aggressive than ATF5+/+ mice
Next, we asked whether ATF5 could affect olfaction initiating behavior. Therefore, the olfaction-related aggressiveness was evaluated. Resident male mice attack the intruder male mice to protect their territory. The aggression of the male mice is evoked by the detection of the pheromones present in the male mouse urine Citation34) by the neurons of the VNO and the MOE.Citation33,38,39) A male ATF5–/– mouse (resident mouse) was caged with a female wild-type mouse for 1 week to establish male territory. After removal of the female mouse, an unfamiliar wild-type intruder male was put into the cage and the number of attacks by the resident mouse was counted. The number of attacks of resident male ATF5–/– mice decreased significantly to 20% of the ATF5+/+ mice (Fig. ).
Discussion
ATF5–/– mice were generated to characterize the physiological function of ATF5. ATF5–/– mice, two thirds of which die as neonates, have smaller olfactory bulbs (OBs) compared to wild-type mice (Figs. and ). The smaller OBs of ATF5–/– mice have a disturbed laminar structure, especially in the GL and the ONL. The interneuron cells are reduced significantly in the GL and GCL and the numbers of BrdU-positive proliferating cells in the SVZ and the OB are reduced significantly in ATF5–/– mice (Figs. and ). Interneurons are supplied from proliferating cells that reside in the SVZ and migrate into the OB via the RMS in the embryonic stage. This attenuated supply of interneuron progenitors from the SVZ to the OB could reduce the number of interneurons in the OB of ATF5–/– mice resulting in, at least in part, the reduced size of the OB in ATF5–/– mice. It was suggested that expression of ATF5 in the VZ and the SVZ might be important for the cell-cycle regulation and for the proliferation of neural progenitors in those regions.Citation12,18) Therefore, ATF5 can be important for OB formation via interneuron supply.
In ATF5–/– mice, the ONL does not surround the OB completely. Moreover, most olfactory axon projections are reduced significantly and fail to reach to the OB from the OE in ATF5–/– mice (Fig. ). In addition, cleaved caspase 3 (an apoptosis marker) is readily detected in the OE of ATF5–/– mice (Fig. S7). These results indicate that apoptosis is induced in the OSNs whose axons are expected to cover the OB, resulting in the abnormal ONL structure. These observations are consistent with the recent report by Wang et al.Citation19) showing that ATF5 is required for maturation of the OSNs and that apoptosis is induced among OSNs in the OE of ATF5–/– mice. In similar to the ATF5–/– phenotype, mice lacking the zinc-finger gene Fez, most of which die within 1 day after birth, have smaller OBs, impaired interneuron development, and axonal projection of OSNs.Citation40) Fez is expressed in the OE, but not in the OB. Examination of Fez-mutant mice suggests that the signals from OSNs controlled the development of the OB. Therefore, lacking of ATF5 expression in the OSN leads to immature OSNs whose axons fail to transmit odor signal to the OB, resulting in the smaller OB. However, it is unknown what kind of factors or genes are involved in the molecular processes of the reduced volume of the OB under the inadequate odorant signal-transmission to the OB. We detected ATF5 mRNA in the OB at P0 and E17 using the PCR technique (M. Umemura and Y. Takahashi, unpublished results). ATF5 mRNA is also detected in the SVZ. In this study, we demonstrated the attenuated BrdU incorporation in the OB and the SVZ of ATF5–/– mice. Therefore, it could be possible that ATF5 in the SVZ might be involved in the poor development of OB under the insufficient transmission of odorant signals to the OB.
ATF5–/– mice have smaller OBs with a disorganized laminar structure compared to wild-type mice. It is reported that the small OB size reflects olfactory hypofunction in human patients.Citation41) We examined whether ATF5–/– mice have impaired olfaction by behavior analysis. The cookie-finding is an olfaction test in which mice search for a cookie hidden under the bedding in the cage.Citation35) ATF5–/– mice tended to take longer to find the hidden cookie (M. Umemura, K. Tsunematsu, and Y. Takahashi, unpublished results). We used a resident–intruder test, in which a resident ATF5–/– male mouse attacked a wild-type male intruder mouse to protect its territory, for the analysis of olfaction. The number of attacks made by the resident ATF5–/– mouse was reduced compared to ATF5+/+ mice (Fig. ). The aggression of male mice is evoked by the detection of the pheromones present in the male mouse urine Citation34) by the neurons of the VNO and the MOE.Citation33,38,39) The olfactory information detected in the MOE is transmitted to the OB, which in turn is transmitted to the primary olfactory cortex. The frequency of aggression of ATF5–/– mice decreased dramatically suggesting that they have weak olfaction. In general, aggressive behavior can be caused by many factors. The ATF5–/– mice olfactory sensitivity to aggressiveness-inducible pheromones warrants further investigation.
In this study, analysis of ATF5-deficient mice revealed that ATF5 is important for the OB development and function. In particular, ATF5 is required to form the inner layer of OB and to supply the interneuron to OB. It has been suggested that the olfactory dysfunction can be induced by chemical, biological, and mental stress. Oxidative chemical burden, viral infection, and mental trauma can cause anosmia.Citation5,7−10) Reduced OB volume is a prominent characteristic in these patients. ATF5 might be concerned with the molecular pathogenesis of these stress-induced OB size-related defects and the olfactory dysfunction. We can assume that ATF5 might function in the proper development of OB under stress conditions. However, this hypothesis remains to be tested. Future work to explore the relationship of ATF5 expression and the olfactory dysfunction is under consideration. Especially, molecular roles of ATF5 in cellular processes of proliferation and differentiation in brain development and adult neurogenesis should be explored in near future. In addition, further study is needed to investigate how ATF5 modulates OB development in detail. Exploration of ATF5-related OB malformation in mice could supply important insights for preventive and therapeutic approaches to human disease.
Supplemental material
The supplemental material for this paper is available at http://dx.doi.org/10.1080/09168451.2015.1012042.
Funding
The work was supported by Japanese government.
Supplemental Materials
Download MS Word (4.2 MB)Acknowledgments
We thank Dr Yoshihiro Yoshihara (Riken) for providing the anti-Tbx21 antibody and Dr Seiko Hoshi for her kind support of formula milk and a nursing bottle of Hoshiba Nipple-Yajima Style for hand-feeding. We thank Dr Takashi Yamazaki, Yumiko Maruyama, Akiko Kawai, Tatsuya Onishi, Kazunori Sasaki, Shun Aoyagi, Yuji Tsunokuma, Ayako Matsuzaki, Tae Ogura, Ryoko Tanabe, and Naoto Mori for their technical supports and Mr. Allen M. Meyer for his excellent proofreading. We also thank Dr Hidenori Hirose, Dr Tatsuya Yoshimi, and Dr Akiko Matsumoto for helpful discussion.
Notes
Abbreviations: AOB, accessory olfactory bulb; ATF5, activating transcription factor 5; BrdU, bromodeoxyuridine; CREB, cAMP responsive element binding; EPL, external plexiform layer; GCL, granule cell layer; GL, glomerular layer; IPL, internal plexiform layer; MCL, mitral cell layer; MOE, main olfactory epithelium; OB, olfactory bulb; ONL, olfactory nerve layer; OSN, olfactory sensory neuron; RMS, rostral migratory stream; SVZ, subventricular zone; and VNO, vomeronasal organ.
References
- Adam Y, Mizrahi A. Circuit formation and maintenance-perspectives from the mammalian olfactory bulb. Curr. Opin. Neurobiol. 2010;20:134–140.10.1016/j.conb.2009.11.001
- Hinds JW. Autoradiographic study of histogenesis in the mouse olfactory bulb. I. Time of origin of neurons and neuroglia. J. Comp. Neurol. 1968;134:287–304.10.1002/(ISSN)1096-9861
- Abolmaali ND, Hietschold V, Vogl TJ, Huttenbrink KB, Hummel T. MR evaluation in patients with isolated anosmia since birth or early childhood. AJNR Am. J. Neuroradiol. 2002;23:157–164.
- Wang J, You H, Liu JF, Ni DF, Zhang ZX, Guan J. Association of olfactory bulb volume and olfactory sulcus depth with olfactory function in patients with Parkinson disease. AJNR Am. J. Neuroradiol. 2011;32:677–681.10.3174/ajnr.A2350
- Negoias S, Croy I, Gerber J, Puschmann S, Petrowski K, Joraschky P, Hummel T. Reduced olfactory bulb volume and olfactory sensitivity in patients with acute major depression. Neuroscience 2010;169:415–421.10.1016/j.neuroscience.2010.05.012
- Nguyen AD, Pelavin PE, Shenton ME, Chilakamarri P, McCarley RW, Nestor PG, Levitt JJ. Olfactory sulcal depth and olfactory bulb volume in patients with schizophrenia: an MRI study. Brain Imaging Behav. 2011;5:252–261.10.1007/s11682-011-9129-0
- Mueller A, Rodewald A, Reden J, Gerber J, von Kummer R, Hummel T. Reduced olfactory bulb volume in post-traumatic and post-infectious olfactory dysfunction. NeuroReport. 2005;16:475–478.10.1097/00001756-200504040-00011
- Rombaux P, Huart C, Deggouj N, Duprez T, Hummel T. Prognostic value of olfactory bulb volume measurement for recovery in postinfectious and posttraumatic olfactory loss. Otolaryngol. Head Neck Surg. 2012;147:1136–1141.10.1177/0194599812459704
- Brenneman KA, James RA, Gross EA, Dorman DC. Olfactory neuron loss in adult male CD rats following subchronic inhalation exposure to hydrogen sulfide. Toxicol. Pathol. 2000;28:326–333.10.1177/019262330002800213
- Ross PM, Whysner J, Covello VT, Kuschner M, Rifkind AB, Sedler MJ, Trichopoulos D, Williams GM. Olfaction and symptoms in the multiple chemical sensitivities syndrome. Prev. Med. 1999;28:467–480.10.1006/pmed.1998.0469
- Persengiev SP, Devireddy LR, Green MR. Inhibition of apoptosis by ATFx: a novel role for a member of the ATF/CREB family of mammalian bZIP transcription factors. Genes Dev. 2002;16:1806–1814.10.1101/gad.992202
- Angelastro JM, Ignatova TN, Kukekov VG, Steindler DA, Stengren GB, Mendelsohn C, Greene LA. Regulated expression of ATF5 is required for the progression of neural progenitor cells to neurons. J. Neurosci. 2003;23:4590–4600.
- Angelastro JM, Mason JL, Ignatova TN, Kukekov VG, Stengren GB, Goldman JE, Greene LA. Downregulation of activating transcription factor 5 is required for differentiation of neural progenitor cells into astrocytes. J. Neurosci. 2005;25:3889–3899.10.1523/JNEUROSCI.3447-04.2005
- Greene LA, Lee HY, Angelastro JM. The transcription factor ATF5: role in neurodevelopment and neural tumors. J. Neurochem. 2009;108:11–22.10.1111/jnc.2008.108.issue-1
- Li G, Xu Y, Guan D, Liu Z, Liu DX. Hsp70 protein promotes survival of C6 and U87 glioma cells by inhibition of ATF5 degradation. J. Biol. Chem. 2011;286:20251–20259.10.1074/jbc.M110.211771
- Liu DX, Qian D, Wang B, Yang JM, Lu Z. p300-Dependent ATF5 acetylation is essential for Egr-1 gene activation and cell proliferation and survival. Mol. Cell. Biol. 2011;31:3906–3916.10.1128/MCB.05887-11
- Dluzen D, Li G, Tacelosky D, Moreau M, Liu DX. BCL-2 is a downstream target of ATF5 that mediates the prosurvival function of ATF5 in a cell type-dependent manner. J. Biol. Chem. 2011;286:7705–7713.10.1074/jbc.M110.207639
- Torres-Peraza JF, Engel T, Martin-Ibanez R, Sanz-Rodriguez A, Fernandez-Fernandez MR, Esgleas M, Canals JM, Henshall DC, Lucas JJ. Protective neuronal induction of ATF5 in endoplasmic reticulum stress induced by status epilepticus. Brain. 2013;136:1161–1176.10.1093/brain/awt044
- Wang SZ, Ou J, Zhu LJ, Green MR. Transcription factor ATF5 is required for terminal differentiation and survival of olfactory sensory neurons. Proc. Natl. Acad. Sci. U.S.A; 2012;109:18589–18594.
- Dalton RP, Lyons DB, Lomvardas S. Co-opting the unfolded protein response to elicit olfactory receptor feedback. Cell. 2013;155:321–332.10.1016/j.cell.2013.09.033
- Zhou D, Palam LR, Jiang L, Narasimhan J, Staschke KA, Wek RC. Phosphorylation of eIF2 directs ATF5 translational control in response to diverse stress conditions. J. Biol. Chem. 2008;283:7064–7073.10.1074/jbc.M708530200
- Watatani Y, Kimura N, Shimizu YI, Akiyama I, Tonaki D, Hirose H, Takahashi S, Takahashi Y. Amino acid limitation induces expression of ATF5 mRNA at the post-transcriptional level. Life Sci. 2007;80:879–885.10.1016/j.lfs.2006.11.013
- Watatani Y, Ichikawa K, Nakanishi N, Fujimoto M, Takeda H, Kimura N, Hirose H, Takahashi S, Takahashi Y. Stress-induced translation of ATF5 mRNA is regulated by the 5′-untranslated region. J. Biol. Chem. 2008;283:2543–2553.10.1074/jbc.M707781200
- Hatano M, Umemura M, Kimura N, Yamazaki T, Takeda H, Nakano H, Takahashi S, Takahashi Y. The 5′-untranslated region regulates ATF5 mRNA stability via nonsense-mediated mRNA decay in response to environmental stress. FEBS J. 2013;280:4693–4707.10.1111/febs.12440
- Inoue N, Ikawa M, Isotani A, Okabe M. The immunoglobulin superfamily protein Izumo is required for sperm to fuse with eggs. Nature. 2005;434:234–238.10.1038/nature03362
- Hoshiba J. Method for hand-feeding mouse pups with nursing bottles. Contemp. Top. Lab. Anim. Sci. 2004;43:50–53.
- Yajima M, Hoshiba J, Terahara M, Yajima T. Reduced thymic size and numbers of splenic CD4 + and CD8 + cells in artificially reared mouse pups. Biosci. Biotechnol. Biochem. 2007;71:2420–2427.10.1271/bbb.70121
- Fukuda T, Sugita S, Inatome R, Yanagi S. CAMDI, a novel disrupted in schizophrenia 1 (DISC1)-binding protein, is required for radial migration. J. Biol. Chem. 2010;285:40554–40561.10.1074/jbc.M110.179481
- Yoshihara S, Omichi K, Yanazawa M, Kitamura K, Yoshihara Y. Arx homeobox gene is essential for development of mouse olfactory system. Development 2005;132:751–762.10.1242/dev.01619
- Tang X, Falls DL, Li X, Lane T, Luskin MB. Antigen-retrieval procedure for bromodeoxyuridine immunolabeling with concurrent labeling of nuclear DNA and antigens damaged by HCl pretreatment. J. Neurosci. 2007;27:5837–5844.10.1523/JNEUROSCI.5048-06.2007
- Long JE, Garel S, Depew MJ, Tobet S, Rubenstein JL. DLX5 regulates development of peripheral and central components of the olfactory system. J. Neurosci. 2003;23:568–578.
- Stowers L, Holy TE, Meister M, Dulac C, Koentges G. Loss of sex discrimination and male-male aggression in mice deficient for TRP2. Science. 2002;295:1493–1500.10.1126/science.1069259
- Mandiyan VS, Coats JK, Shah NM. Deficits in sexual and aggressive behaviors in Cnga2 mutant mice. Nat. Neurosci. 2005;8:1660–1662.10.1038/nn1589
- Chamero P, Marton TF, Logan DW, Flanagan K, Cruz JR, Saghatelian A, Cravatt BF, Stowers L. Identification of protein pheromones that promote aggressive behaviour. Nature 2007;450:899–902.10.1038/nature05997
- Le Pichon CE, Valley MT, Polymenidou M, Chesler AT, Sagdullaev BT, Aguzzi A, Firestein S. Olfactory behavior and physiology are disrupted in prion protein knockout mice. Nat. Neurosci. 2009;12:60–69.10.1038/nn.2238
- Gratzner HG. Monoclonal antibody to 5-bromo- and 5-iododeoxyuridine: a new reagent for detection of DNA replication. Science 1982;218:474–475.10.1126/science.7123245
- Miller MW, Nowakowski RS. Use of bromodeoxyuridine-immunohistochemistry to examine the proliferation, migration and time of origin of cells in the central nervous system. Brain Res. 1988;457:44–52.10.1016/0006-8993(88)90055-8
- Wang Z, Balet Sindreu C, Li V, Nudelman A, Chan GC, Storm DR. Pheromone detection in male mice depends on signaling through the type 3 adenylyl cyclase in the main olfactory epithelium. J. Neurosci. 2006;26:7375–7379.10.1523/JNEUROSCI.1967-06.2006
- Nunez-Parra A, Pugh V, Araneda RC. Regulation of adult neurogenesis by behavior and age in the accessory olfactory bulb. Mol. Cell. Neurosci. 2011;47:274–285.10.1016/j.mcn.2011.05.003
- Hirata T, Nakazawa M, Yoshihara S, Miyachi H, Kitamura K, Yoshihara Y, Hibi M. Zinc-finger gene Fez in the olfactory sensory neurons regulates development of the olfactory bulb non-cell-autonomously. Development 2006;133:1433–1443.10.1242/dev.02329
- Buschhuter D, Smitka M, Puschmann S, Gerber JC, Witt M, Abolmaali ND, Hummel T. Correlation between olfactory bulb volume and olfactory function. Neuroimage 2008;42:498–502.10.1016/j.neuroimage.2008.05.004