Abstract
We investigated the ameliorative effect of freshwater clam extract (FCE) on fatty liver, hypercholesterolemia, and liver injury in rats exposed to chloretone. Furthermore, we examined the effects of major FCE components (fat and protein fractions) to determine the active components in FCE. Chloretone increased serum aminotransferase activities and led to hepatic lipid accumulation. Serum aminotransferase activities and hepatic lipid content were lower in rats fed total FCE or fat/protein fractions of FCE. Expression of fatty acid synthase and fatty acid desaturase genes was upregulated by chloretone. Total FCE and fat/protein fractions of FCE suppressed the increase in gene expression involved in fatty acid synthesis. Serum cholesterol levels increased twofold upon chloretone exposure. Total FCE or fat/protein fractions of FCE showed hypocholesterolemic effects in rats with hypercholesterolemia induced by chloretone. These suggest that FCE contains at least two active components against fatty liver, hypercholesterolemia, and liver injury in rats exposed to chloretone.
Graphical abstract
Freshwater clam extract (FCM) suppresses accumulation of hepatic lipids and liver cell injury induced by xenobiotics.
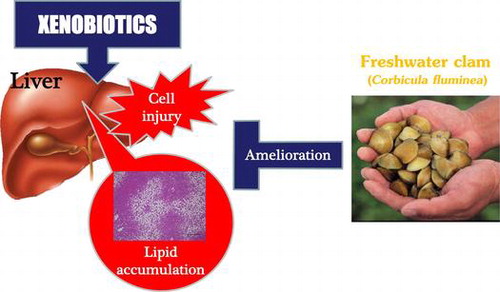
Xenobiotics are generally encountered as environmental pollutants, agrochemicals, and drugs. Administration of xenobiotics to animals causes many pathologic and metabolic changes. Such changes include: (i) lipid accumulation in the liver,Citation1,2) (ii) enhancement of lipid peroxidation,Citation3,4) (iii) hypercholesterolemia with elevated levels of high-density lipoprotein (HDL) cholesterol and apolipoprotein (apo) A–I,Citation5,6) (iv) activation of microsomal drug-metabolizing enzymes in the liver,Citation7) (v) liver injury,Citation8) (vi) decreased levels of vitamin A in the liver,Citation4) and (vii) increased levels of ascorbic acid in the urine and tissues of rats.Citation1,9) It has been speculated that xenobiotic-induced fatty liver disease is caused by the expression of the genes of lipogenic enzymes.Citation10) Oral administration of xenobiotics causes fatty liver and chronic mild liver injury in rats.Citation4) Therefore, this model may be a feasible experimental model for liver disorders without genetic modification and ingestion of alcohol.
The freshwater clam (Corbicula fluminea) is a popular edible bivalve in Asia and is believed (particularly in Japan) to be beneficial to liver health. Studies have reported that freshwater clam extract (FCE) protects against liver injury,Citation11–13) ameliorates hypercholesterolemiaCitation14–17) and fatty liver disease,Citation11,15) and accelerates ethanol metabolism.Citation11) Previously, we focused particularly on the hypocholesterolemic effects of FCECitation14–17) and found that FCE not only has hypocholesterolemic effects but also other beneficial effects against fatty liver diseaseCitation15) and fatty acid metabolism.Citation18) Furthermore, FCE prevents the acute injury to liver cells induced by D-galactosamine,Citation11) hemorrhage,Citation12) or carbon tetrachloride (CCl4).Citation13)
Therefore, we investigated FCE for its protective effects on fatty liver and liver injury in rats fed the xenobiotic chloretone and found that FCE or its major components, fat or protein fractions, ameliorated xenobiotic-induced liver dysfunction. Taken together, our results suggest that FCE has various beneficial effects against liver injury accompanied by the accumulation of hepatic lipid.
Materials and methods
Materials
FCE was prepared using a procedure described previously.Citation11,14–18) Briefly, freshwater clams were steamed until their shells opened, and then the edible portions were removed. Edible portions were minced, extracted with boiling water, and passed through an 80-mesh filter. The filtrate was spray-dried for use as FCE. FCE yield from raw material was approximately 1.5% (w/w). FCE contained soluble materials and comprised approximately 35% insoluble materials. The approximate composition of 100 g FCE is 62.8 g protein, 6.9 g carbohydrate, 4.0 g moisture, 20.9 g crude fat, and 5.4 g ash. FCE was extracted overnight in 5 volumes of chloroform:methanol mixture (2:1, v/v). The residue was extracted once with the same solvent. The chloroform:methanol extract was evaporated at < 40 °C using a rotary evaporator. The remainder was used as the fat fraction, which yielded 21% (w/w) from FCE. The fat fraction had a dark brown color and was a highly viscous liquid. The phytosterol concentration and fatty acid composition in the fat fraction have been described previously.Citation16) The total amount of phytosterols in the fat fraction of FCE was 0.71%.Citation16)
A notable difference has been observed between FCE and corn oil in terms of the concentrations of oleic and linoleic acids, which are the major components of corn oil (the oil control used in the present study). Concentrations of oleic acid and linoleic acid in FCE fat are less than one-thirteenth and one-fifty-second of those concentrations in corn oil.Citation16) In addition, levels of long-chain and polyunsaturated fatty acids are extremely low in the fat fraction of FCE.Citation16) Previously,Citation17) we fractionated the FCE fat fraction into nine sub-fractions by silica gel column chromatography, and then revealed the characteristics of each sub-fraction by thin-layer chromatography (TLC). TLC analysis revealed that the FCE fat fraction contains several lipophilic components (e.g. triacylglycerols, sterols, sterol esters, and sphingolipids).Citation17) Also, two fractions containing mainly sphingolipids, triacylglycerols, and sterol esters exerted potent hypocholesterolemic effects in rats fed a high-cholesterol diet.Citation17) The residue was air-dried at room temperature and suspended in 4 volumes of sodium acetate buffer (8 mM, pH 4.8). Next, β-amylase (EC3.2.1.2; 45.2 unit/mg; type II-B from barley; Sigma–Aldrich, Saint Louis, MO, USA) was added at an enzyme:substrate ratio of 1:1000 (w/w) to decompose polysaccharides (mainly glycogen). Incubation continued at room temperature for 2 h, and samples were boiled for 15 min to deactivate β-amylase. The product of enzymatic decomposition was added to 3 volumes of acetone and incubated at −20 °C overnight to precipitate proteins, which were air-dried at room temperature to obtain the protein fraction. The resultant protein fraction was 71% (w/w) of FCE. We have described the amino acid composition of FCE in a previous study.Citation15) Levels of glycine and proline were threefold more in FCE and one-third the levels found in casein, respectively.Citation15) The approximate composition of the 100 g FCE protein fraction was 74.9 g protein, 11.5 g carbohydrate, 7.5 g moisture, 0.1 g crude fat, and 6.0 g ash.
Animals
The experimental procedures used in this study complied with the guidelines set by the Animal Care and Use Committee of Oita University (Oita, Japan). Male Wistar rats (4 weeks; approximately 70 g; Japan SLC, Inc., Hamamatsu, Japan) were housed in individual stainless steel cages in an environmentally controlled room maintained at 23 °C with a 12 h light–dark cycle (lights on 20:00–08:00). Throughout the study, rats had access to food and tap water ad libitum.
Diet
Rats were fed a commercial stock diet (5L37; Japan SLC, Inc.) to adapt to a breeding environment for 2 days. Then, they were fed a 20% casein diet to adapt to a type of feedstuff for 3 days. The composition of each test diet is shown in Table .
Table 1. Composition of the experimental diets.
Thirty male rats were divided into five groups of six: 1 (basal diet); 2 (control diet); 3 (FCE diet); 4 (fat fraction of FCE); and 5 (protein fraction of FCE). Rats in groups 2–5 were fed a diet containing chloretone. Animals were given their respective test diets for 14 days. Previously, we compared diets that contained 3, 15, or 30% FCE, and we found that 30% FCE was the most effective for lowering cholesterol in xenobiotic-treated rats (unpublished results). Moreover, we determined that 14 days was a sufficient period to observe the effects of FCE because the hypocholesterolemic effect reached a plateau at 30% FCE within 14 days (unpublished results). Therefore, we used a 30% FCE diet for 14 days in this experiment. Doses of the fat and protein fractions of FCE used in this experiment were equivalent to those found in 30% FCE. The chloretone diet comprised the basal diet supplemented with 3 g chloretone/kg body weight. Test substances were added to the chloretone diet via casein, sucrose, or corn oil. Fat-fraction doses were decided by calculating the yield for the fat fraction from a diet containing 30% FCE. The protein in the diet that contained the FCE protein fraction was obtained only from the protein fraction of FCE. Protein levels in each diet were adjusted on an isonitrogenous basis at the expense of cornstarch and sucrose. All diets consisted of approximately 7% fat, which was supplied by 7% corn oil (basal, control, and protein fraction diets), 0.95% corn oil plus 6.05% fat from FCE (FCE diet), or 0.95% corn oil plus 6.05% of the fat fraction of FCE (FCE fat-fraction diet). Mineral mix and vitamin mix were added at concentrations of 35 and 10 g/kg diet, respectively.
Sample collection
Feces were collected during the final 3 days of the experimental period (11–14 days) and used to determine the levels of neutral sterols and bile acids. All rats were killed on the last day of the experimental period by decapitation at 10:00, after 4 h of fasting. Blood was collected in heparin-containing tubes. Livers were removed, weighed, flash-frozen in liquid nitrogen, and stored at −80 °C until analysis of hepatic lipids and genes linked to drug metabolism, cholesterol synthesis, and metabolism. Liver samples were also fixed in 10% formalin for histologic analysis.
Biochemical analysis
Serum levels of aspartate aminotransferase (AST; EC2.6.1.1) activity, alanine aminotransferase (ALT; EC2.6.1.2) activity, alkaline phosphatase (ALP; EC3.1.3.1) activity, total cholesterol, HDL-cholesterol, triglycerides, and free fatty acids were determined using commercial kits. Determiner AST II, Determiner ALT II, and Determiner ALP were purchased from Kyowa-Medics, Inc. (Tokyo, Japan). T-CHO and TG-EN were obtained from Kainos Laboratories (Tokyo, Japan). HDL-Cholesterol Test was purchased from Wako Pure Chemical Industries (Osaka, Japan). NEFA reagent B was obtained from Sysmex Corporation (Hyogo, Japan). Approximately 2.5 g liver was homogenized, and lipids were extracted with a chloroform:methanol mixture (2:1, v:v) as described by Folch et al.Citation19). Total liver lipids were determined gravimetrically. Concentrations of cholesterol and triglycerides in lipid extracts from livers were determined by the methods described above. Phospholipid concentrations in lipid extracts from livers were measured using Phospholipids C-Test (Wako Pure Chemical Industries).
Total neutral sterols and bile acids were extracted from fecal matter by the method described by Delaney et al.Citation20) Neutral sterols in feces were analyzed as trimethylsilyl esters using gas chromatography/mass spectrometry (GC/MS) (GC 6890 equipped with 5973MSD and a 30 m × 0.25 mm HP-5MS capillary column; Agilent Technologies, Santa Clara, CA, USA), with 5α-cholestane used as the internal standard. Injector and detector temperatures were set at 300 and 230 °C, respectively. The initial column temperature of 245 °C was maintained for 2 min and then increased to 300 °C at 2 °C/min. Total bile acids in feces were determined by enzymatic means by the method described by Sheltawy and Losowsky, with lithocholic acid included as a standard.Citation21)
Total RNA was isolated according to the method described by Chomczynski and Sacchi.Citation22) Total RNA (20 μg) was subjected to northern blot hybridization and quantitative real-time polymerase chain reaction (qRT-PCR). For analysis of northern blotting, cDNA clones of rat cytochrome P-450 (Cyp)1a1, rat Cyp2b1, and mouse Apoe were labeled using the Megaprime DNA Labeling system (Amersham, Buckinghamshire, UK) and used for hybridization. Specific hybridization was quantified by an image analyzer (BAS 2500; Fuji Film, Tokyo, Japan). The quantity of Apoe mRNA was unaffected by all treatments employed in this study (data not shown), so we used it as a normalization standard.Citation23,24) For the qRT-PCR assay, cDNA was prepared using a High-capacity cDNA Reverse Transcription kit (Applied Biosystems, Carlsbad, CA, USA) according to manufacturer instructions. qRT-PCR amplifications were undertaken using ABI StepOne (Applied Biosystems) in a reaction mixture of 20 μL, which contained 8 μL of cDNA (1:80 dilution), 10 μL of 2 × Power SYBR Master Mix (Applied biosystems), and 200 nM of each primer. The primers used were as follows: rat mRNA of Fasn forward, 5′-CCAAGCAGGCACACACAATG-3′; rat mRNA for Fasn reverse, 5′-GATACCTCCGTCGACAATAG-3′, rat mRNA for Fads1 forward, 5′-GCTGAAAGGCCTCCTATGTC-3′; rat mRNA for Fads1 reverse, 5′-TTCCGGTCATGATCAATGTG-3′, rat mRNA for Me forward, 5′-CTTGTTGCCACCCTGCATTG-3′; rat mRNA for Me reverse, 5′-TAAGCACACTGTAGAAGAGC-3′, rat mRNA for Cyp7a1 forward, 5′-TGTGTGAGGGACCAGGTCTCT-3′; rat mRNA for Cyp7a1 reverse, 5′-AGCTCCAAAAGGTTGGAGGA-3′, rat mRNA for Abcg5 forward, 5′-TGTCCTTCAGCGTCAGCAAC-3′; rat mRNA for Abcg5 reverse, 5′-TCTGGCCACTCTCGATGTAC-3′, rat rRNA for 18s forward, 5′-CGCCGCTAGAGGTGAAATTC-3′; rat rRNA for 18s reverse, 5′-TTGGCAAATGCTTTCGCTC-3′. Melting curve data for qRT-PCR were collected to determine PCR specificity. Expression was calculated using standard curves. Gene expression was normalized with 18s ribosomal RNA as an internal control.
Histological methods
Liver tissues fixed in formalin were embedded in paraffin and stained with hematoxylin and eosin (H & E). Frozen liver sections were used for Oil Red-O staining. Sections were sliced (thickness, 2 μm), stained with H & E or Oil Red-O, and examined under light microscopy. Tissue images were taken using a microscope (BZ-9000; Keyence, Osaka, Japan).
Statistical analysis
Data are the mean ± SE. Differences among values were analyzed for significance using one-way analysis of variance (ANOVA) followed by Tukey’s multiple-range test.Citation25) These analyses were undertaken using SPSS version 10.0 (SPSS Japan; Tokyo, Japan). Differences were considered significant at p < 0.05.
Results
No significant differences in food intake were observed among all five groups, even though rats had ad libitum access to diets (Table ). The body weight gain in rats fed the chloretone diet was less than that in rats fed the chloretone-free basal diet. Supplementation of chloretone diets with total FCE or with fat/protein fractions of FCE did not affect body weight gain compared with chloretone diets without these test substances. Compared with the basal diet group, liver size increased significantly in response to the control group (Table ). Rats fed FCE or protein fraction of FCE exhibited significantly lighter weights of liver than those in the control group. Serum activities of AST and ALT (markers of hepatocellular injury) and ALP (biochemical marker of cholestasis) are listed in Table . Serum activities of AST and ALT were significantly higher in the control group than in the basal group (Table ). Supplementation of chloretone diets with total FCE or fat/protein fractions of FCE suppressed increments in AST and ALT. Chloretone exposure did not alter serum activities of ALP, but ALP activities were reduced in rats fed a total FCE diet or fat/protein fractions of FCE (Table ).
Table 2. Effect of FCE and its fat/protein fractions on food intake, body weight, relative liver weight, serum parameters, and hepatic lipids in rats fed chloretone for 14 daysTable Footnote1.
Total cholesterol in serum was significantly higher in rats fed a chloretone diet than in rats fed a basal diet (Table ). Total FCE and fat/protein fractions of FCE suppressed the increased serum level of cholesterol. Serum levels of HDL cholesterol were also significantly higher in the control group than in the basal group. Rats fed FCE or fat fractions of FCE exhibited lower serum levels of HDL cholesterol than those in the chloretone group, but significant differences in serum levels of triglycerides were not observed among the groups (Table ). Rats fed a chloretone diet exhibited slightly higher levels of free fatty acids in serum than rats fed a basal diet (p > 0.05). Rats fed the fat fraction of FCE exhibited lower levels of free fatty acids in serum than the control group (Table ).
Levels of total lipids, cholesterol, triglycerides, and phospholipids in the liver were greater in rats fed a chloretone diet than in those fed a basal diet (Table ). Levels of total lipids, cholesterol, and triglycerides in the livers of rats fed total FCE or fat/protein fractions of FCE were significantly lower than those in the control group (Table ). Liver phospholipids were unaltered upon supplementation of the chloretone diet with total FCE or the fat/protein fraction of FCE (Table ).
The photomicrographs in Fig. (A)–(E) show notable morphologic changes in livers stained with H & E from rats fed chloretone and FCE. The chloretone diet increased the number of vacuoles in the periportal area (zone 1); supplementation with total FCE or fat/protein fractions of FCE resulted in obvious amelioration of vacuole accumulation. Oil Red-O staining confirmed that the vacuoles were fat droplets (Fig. (F)–(J)). These results are consistent with the finding of lipid accumulation in the liver (Table ).
Fig. 1. Histologic changes in liver lobules by FCE and its fat/protein fractions in rats fed chloretone for 14 days.
Notes: All images are light micrograph liver sections and show rats fed a (A), (F) basal diet; (B), (G) diet that contained chloretone; (C), (H) diet that contained chloretone supplemented with total FCE; (D), (I) diet that contained chloretone supplemented with the fat fraction of FCE; (E), (J) diet that contained chloretone supplemented with the protein fraction of FCE. In figure (A)–(E), sections were stained with H & E, and scale bar indicate 100 μm. P, portal vein; C, central vein. In figure (F)–(J), sections were stained with Oil Red-O, and scale bar indicate 500 μm.
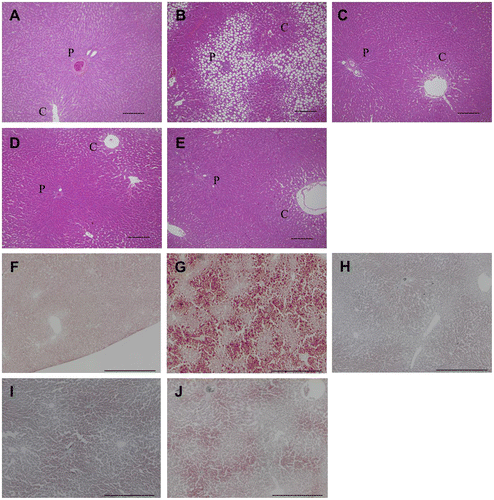
Fecal weight and sterol composition from the final 3 days of the experiment are shown in Table . The dry weight of the feces was heavier in rats fed total FCE or fat/protein fractions of FCE than in basal and control groups. Chloretone exposure did not alter the levels of neutral sterols (i.e. sum of cholesterol, coprostanol, and coprostanone) in feces. Levels of total neutral sterols were higher in the feces of rats fed total FCE or fat fractions of FCE than in the feces of control rats (Table ). Chloretone supplementation of the basal diet did not affect excretion of bile acid into feces. Levels of bile acids in feces were significantly higher in rats fed total FCE or fat/protein fractions of FCE than that in rats fed the basal diet and in control groups (Table ).
Table 3. Changes in feces and fecal sterols by FCE and its fat/protein fractions in rats fed chloretone for 14 daysTable Footnote1.
To gain an insight into the mechanism by which FCE ameliorates liver injury and fatty liver disease, we investigated expression of liver genes with regard to inducible drug-metabolizing enzymes and lipid metabolism. Gene expression of the typical drug-inducible Cyps—Cyp1a1/1a2 and Cyp2b1/2b2—was measured (Fig. ). The amount of Cyp1a1/1a2 mRNA was slightly higher in rats fed total FCE or fat fractions of FCE than in those of the control group (p > 0.05), whereas hepatic mRNA of Cyp1a1/1a2 was unaffected by dietary chloretone. Chloretone induced expression of Cyp2b1/2b2 genes in the liver, but FCE or fat/protein fractions of FCE did not affect levels of Cyp2b1/2b2 mRNA.
Fig. 2. Changes of hepatic gene expression by FCE and its fat/protein fractions in rats fed chloretone for 14 days.
Notes: Levels of mRNA for Cyp1a1 and Cyp2b1 were measured by northern blotting. Levels of Apoe mRNA were used as normalization standards for northern blotting because these levels were not changed significantly in our experiments. Levels of mRNA for Abcg5, Cyp7a1, Fads1, Fasn, and Me were measured by RT-PCR. Additionally, 18s ribosomal RNA was used as the internal control for the mRNA levels analyzed by RT-PCR. Statistical differences among values were analyzed by ANOVA and Tukey’s multiple-range test. Data are the mean ± SE represented by vertical bars. a, b Mean values with different letters indicate a significant difference; p < 0.05. FCE, freshwater clam extract; Abcg5, ATP-binding cassette G5; Cyp, cytochrome P450; Cyp7a1, cholesterol 7 alpha-hydroxylase; Fasn, fatty acid synthase; Fads1, fatty acid desaturase 1; Me, malic enzyme.
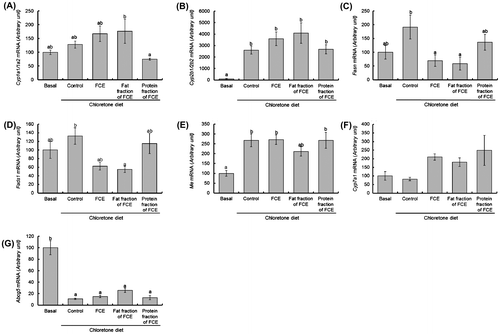
Hepatic levels of mRNA from the key enzymes of lipid metabolism, such as fatty acid synthase (Fasn), fatty acid desaturase (Fads) 1, and malic enzyme (Me), are indicated in Fig. . Typically, Fasn and Fads1 mRNA were higher in rats fed chloretone than in rats fed a basal diet, but the difference between these two groups was not significant. The chloretone-induced increase in the levels of hepatic Fasn mRNA was suppressed by FCE or fat fractions of FCE. The increase of Fads1 mRNA level induced by chloretone tended to be suppressed by FCE, and significantly suppressed by fat fraction of FCE. However, the protein fractions of FCE did not affect mRNA levels of Fasn and Fads1. Hepatic levels of the mRNA of Me were higher in rats fed chloretone as compared with those fed a basal diet. The induced expression by chloretone of the Me gene did not change due to the FCE diet or fat/protein fractions of FCE. The induced expression by chloretone of the Me gene did not change due to the FCE diet or fat/protein fractions of FCE. The mRNA levels of hepatic Cyp7a1 (the rate-limiting enzyme in the metabolic pathway from cholesterol to bile acids) tended to be higher in rats fed total FCE and fat/protein fractions of FCE than the levels in the control group, though the difference was not significant. Hepatic expression of the adenosine triphosphate-binding cassette (Abc)g5 gene was suppressed by chloretone; fat fractions of FCE tended to increase levels of Abcg5 mRNA compared with control rats, but the difference was not significant (Fig. ).
Discussion
Studies have shown that freshwater clams have protective properties against liver injury induced D-galactosamine,Citation11) hemorrhage,Citation12) and CCl4Citation13) in rats. In this study, we examined whether FCE and fat/protein fractions of FCE exerted hepatoprotective effects in rats with hepatic lipid accumulation induced by exposure to the xenobiotic chloretone.
The livers of rats that were fed chloretone showed lipid accumulation (Fig. ). Moreover, serum activities of aminotransferases (biomarkers of damage to liver cells) were increased by feeding chloretone to rats. We demonstrated that FCE prevents hepatic accumulation of lipid droplets and hepatocellular damage and that the fat/protein fractions of FCE exhibit similar effects (Table and Fig. ). FCE and its fractions (especially fat fractions) showed potent lipid-lowering and hepatoprotective effects.
In this study, FCE and its fractions suppressed the accumulation of lipids (mainly triglycerides) in hepatocytes. Previously, we suggested that fatty liver disease is induced by xenobiotics through the induction of NADPH-generating enzymes, including glucose-6-phosphate dehydrogenase (G6PD), ME, and 6-phosphogluconate dehydrogenase in the liver.Citation10) Our recent transcriptomic study indicated that the mechanisms used by FCE to lower hepatic levels of triglycerides may be related to the regulation of gene expression of the synthesis and signaling of fatty acids.Citation18) Moreover, analyses of microarray networks led us to hypothesize that sterol regulatory element-binding protein-1, the key transcriptional regulator of expression of lipogenic genes, is involved in the action of FCE.Citation18) In addition, FCE affected expression of the gene encoding key lipogenic enzymes (Fig. ).
Xenobiotic diets that include components such as chloretone, polychlorinated biphenyls (PCBs), and butylated hydroxytoluene have been shown to induce CYP expression.Citation6) CYPs have been suggested to be a source of reactive oxygen species (ROS) due to the release of activated oxygen molecules such as superoxide and hydrogen peroxide during microsomal electron transport.Citation26) Additionally, xenobiotics induce hepatic lipid peroxidation.Citation27) It has been hypothesized that a central cause of xenobiotic-induced hepatotoxicity is excessive lipid peroxidation. Hepatic gene expression of Cyp2b1/2b2 was induced by chloretone (Fig. ), but induction of expression of Cyp2b1/2b2 genes was unaltered by FCE (Fig. ). Therefore, the ameliorative effect of FCE on liver injury and fatty liver disease could not be explained by modulation of chloretone-induced drug-metabolizing enzymes. Recently, Hsu et al.Citation13) reported that oral administration of FCE suppressed hepatic lipid peroxidation in rats treated with CCl4. In contrast, we reported that high dietary levels of vitamin E suppressed the hepatic lipid peroxidation induced by PCBs, but that PCB-induced liver lesions were not ameliorated by vitamin E.Citation4) Moreover, Gulauert et al.Citation28) reported that dietary vitamin E elevated hepatic levels of α-tocopherol, but that vitamin E did not affect the hepatic lesions induced by PCB administration in rats. Therefore, hepatic lipid peroxidation itself may not cause the morphologic damage induced by xenobiotics.Citation4) ROS induce nuclear expression of factor-kappa B,Citation29) which is involved in upregulation of expression of many proinflammatory genes.Citation30) FCE administration ameliorates liver damage by suppressing the production of tumor necrosis factor (TNF)-α, which is induced by hemorrhage.Citation12) However, more investigations are required to ascertain if FCE and its fractions suppress TNF-α production in rats exposed to xenobiotics.
Iritani et al.Citation31) indicated that triglycerides prepared from the freshwater clam Corbicula japonica caused a reduction in hepatic levels of G6PD and ME. The sum of long-chain polyunsaturated fatty acids (C18:4, C20:5, and C22:6) in triglycerides of C. japonica was >35% of the total, instead of linoleic acid (C18:2), in corn oil.Citation31) They speculated that these long-chain polyunsaturated fatty acids might be the active triglyceride components in freshwater clams that reduce lipogenesis.Citation31) Moreover, n-3 polyunsaturated fatty acids exert protective effects against liver injury by reducing TNF-α in the non-genetic model of non-alcoholic steatohepatitis in rats.Citation32) In fat fractions of FCE, the concentrations of oleic and linoleic acids (which are the major fatty acids in corn oil [control oil]) are less than one-thirteenth and one-fiftieth, respectively, of the concentrations found in corn oil.Citation16) In addition, long-chain and polyunsaturated fatty acids in the fat fractions of FCE are very low.Citation16) Nonetheless, the fat fractions of FCE suppressed hepatic lipid accumulation and liver injury (Table and Fig. ). Therefore, the effective component in the fat fractions of FCE may be an unknown fat-soluble compound other than long-chain polyunsaturated fatty acids.
Dietary proteins and their specific composition of amino acids are known to affect hepatic lipogenesis.Citation33–35) Additionally, specific dietary amino acids have hepatoprotective effects.Citation36) Protein fractions of FCE suppressed lipid deposition and cell damage in hepatocytes (Table and Fig. ). Previously, we indicated that the amino-acid composition of FCE is similar to that of casein, but that the level of glycine in FCE was threefold higher than that of glycine in comparison with casein.Citation15) Some studies have reported that dietary levels of glycine affect lipogenesis.Citation37,38) In addition, Stachlewitz et al.Citation39) demonstrated that dietary glycine shows hepatoprotective effects against the hepatotoxicity induced by D-galactosamine. Glycine suppresses TNF-α production by Kupffer cells.Citation40) Whether glycine levels in FCE explain the hypolipogenic and hepatoprotective actions of FCE would be interesting to consider. Iritani et al.Citation33) reported that dietary plant proteins (wheat gluten or soybean protein) suppress hepatic accumulation of triglycerides and the activity of lipogenic enzymes (e.g. G6PD and ME) to higher levels than that by dietary animal proteins (casein or fish protein). They also indicated that soybean protein suppresses gene expression of lipogenic enzymes to a greater extent than casein.Citation34) Ascencio et al.Citation41) demonstrated that soybean protein exerts its preventive effects against fatty liver disease by suppressing expression of the Srebf1 gene in the liver. Mixtures of amino acids that simulate soybean protein suppress the hepatic activity of G6PD and ME, but such mixtures do not affect triglyceride deposition in the liver compared with those that simulate casein.Citation33) Therefore, we speculate that unknown mechanisms (but not the composition of amino acids) are responsible for decreased deposition of lipids in rats fed protein fractions of FCE.
Rats used in this study developed not only fatty liver and liver injury but also hypercholesterolemia, which was induced by enhanced cholesterogenesis in the liver (Table ).Citation42) FCE and its fat/protein fractions exerted a hypocholesterolemic effect in hypercholesterolemic rats in which cholesterogenesis was stimulated by feeding chloretone (Table ). Studies have indicated that the mechanisms underlying the hypocholesterolemic effects of FCE are triggered primarily by enhanced hepatic degradation of cholesterol to bile acids; cholesterol excretion from the liver to the bile duct; and fecal excretion of neutral sterols and bile acids.Citation14–16) In this study, the fat/protein fractions of FCE tended to upregulate expression of Cyp7a1 in the liver (the rate-limiting enzyme in the metabolic pathway from cholesterol to bile acids). The fat/protein fractions of FCE exerted different effects on sterol excretion in feces (Table ). The fat fractions of FCE enhanced excretion of neutral sterols and bile acids into feces in hypercholesterolemic rats fed chloretone, whereas the protein fractions of FCE enhanced fecal excretion of bile acids and did not affect excretion of neutral sterols in hypercholesterolemic rats fed chloretone. These hypocholesterolemic actions of the fat/protein fractions of FCE in a hypercholesterolemic rats fed chloretone are similar to those in other types of hypercholesterolemic rats fed a high-cholesterol diet.Citation16) In addition, our data suggest that chloretone suppresses hepatic gene expression of Abcg5, which has a critical role in the excretion of cholesterol from the liver to the bile duct. Therefore, suppression of expression of the Abcg5 gene may (at least in part) explain the hypercholesterolemia induced by xenobiotics. FCE and its fat/protein fractions upregulated hepatic expression of the Abcg5 gene in hypercholesterolemic rats induced by feeding a high-cholesterol diet in our previous study,Citation16) but FCE and its two major fractions did not affect expression of the Abcg5 gene in the hypercholesterolemic model using chloretone employed in this study.
This study revealed that FCE and its fat/protein fractions exerted a protective effect on hepatic lipid accumulation in rats exposed to the xenobiotic chloretone. Analyses of DNA microarrays have revealed that FCE suppresses gene expression in lipogenesis in normal rat livers.Citation18) In this study, FCE and its fat fractions suppressed hepatic expression of Fasn and Fads1 genes, but the protein fractions of FCE did not affect gene expression. Thus, we speculate that the fat fractions and protein fractions affect different genes. Therefore, FCE contains multiple active components, and the action of FCE is due to multiple mechanisms.
Conflicts of interest
The authors declare no conflict of interest.
Acknowledgment
We received no specific grants from other institutions.
Notes
Abbreviations: Abc, ATP-binding cassette; ANOVA, analysis of variance; apo, apolipoprotein; CCl4, carbon tetrachloride; Cyp, cytochrome P-450; Fads, fatty acid desaturase; Fasn, fatty acid synthase; FCE, freshwater clam extract; G6PD, glucose-6-phosphate dehydrogenase; GC/MS, gas chromatography/mass spectrometry; HDL, high-density lipoprotein; H & E, hematoxylin and eosin; Me, malic enzyme; PCB, polychlorinated biphenyl; qRT-PCR, quantitative real-time polymerase chain reaction; ROS, reactive oxygen species; Srebf, sterol regulatory element-binding transcription factor; TNF, tumor necrosis factor.
References
- Kato N, Tani T, Yoshida A. Effect of dietary level of protein on liver microsomal drug-metabolizing enzymes, urinary ascorbic acid and lipid metabolism in rats fed PCB-containing diets. J. Nutr. 1980;110:1686–1694.
- Oda H, Matsushita N, Hirabayashi A, Yoshida A. Cholesterol-rich very low density lipoproteins and fatty liver in rats fed polychlorinated biphenyls. Biosci. Biotechnol. Biochem. 1994;58:2152–2158.10.1271/bbb.58.2152
- Kato N, Kawai K, Yoshida A. Effect of dietary level of ascorbic acid on the growth, hepatic lipid peroxidation, and serum lipids in guinea pig fed polychlorinated biphenyls. J. Nutr. 1981;111:1727–1733.
- Oda H, Yamashita K, Sasaki S, Horio F, Yoshida A. Long-term effects of dietary polychlorinated biphenyl and high level of vitamin E on ascorbic acid and lipid metabolism in rats. J. Nutr. 1987;117:1217–1223.
- Oda H, Matsushita N, Hirabayashi A, Yoshida A. Hyperlipoproteinemia in rats fed polychlorinated biphenyls. J. Nutr. Sci. Vitaminol. 1990;36:117–122.10.3177/jnsv.36.117
- Oda H, Yoshida A. Effect of feeding xenobiotics on serum high density lipoprotein and apolipoprotein A-I in rat. Biosci. Biotechnol. Biochem. 1994;58:1646–1651.10.1271/bbb.58.1646
- Poland A, Knutson JC. 2,3,7,8-Tetrachlorodibenzo-p-dioxin and related halogenated aromatic hydrocarbons: examination of the mechanism of toxicity. Annu. Rev. Pharmacol. Toxicol. 1982;22:517–554.10.1146/annurev.pa.22.040182.002505
- Sturgill MG, Lambert GH. Xenobiotic-induced hepatotoxicity: mechanisms of liver injury and methods of monitoring hepatic function. Clin. Chem. 1997;43:1512–1526.
- Horio F, Yoshida A. Effect of some xenobiotics on ascorbic acid metabolism in rats. J. Nutr. 1982;112:416–425.
- Hitomi Y, Wakayama M, Oda H, Yoshida A. Liver-specific induction of NADPH-generating enzymes by polychlorinated biphenyls in rats. Biosci. Biotechnol. Biochem. 1993;57:1134–1136.10.1271/bbb.57.1134
- Chijimatsu T, Yamada A, Miyaki H, Yoshinaga T, Murata N, Hata M, Abe K, Oda H, Mochizuki S. Effect of freshwater clam (Corbicula fluminea) extract on liver function in rat. Nippon Shokuhinkagaku Kougaku Kaishi (in Japanese). 2008;5:63–68.10.3136/nskkk.55.63
- Peng TC, Subeq YM, Lee CJ, Lee CC, Tsai CJ, Chang FM, Lee RP. Freshwater clam ameliorates acute liver injury induced by hemorrhage in rats. Am. J. Chin. Med. 2008;36:1121–1133.10.1142/S0192415X08006466
- Hsu CL, Hsu CC, Yen GC. Hepatoprotection by freshwater clam extract against CCl4-induced hepatic damage in rats. Am. J. Chin. Med. 2010;38:881–894.10.1142/S0192415X10008329
- Chijimatsu T, Tatsuguchi I, Abe K, Oda H, Mochizuki S. A freshwater clam (Corbicula fluminea) extract improves cholesterol metabolism in rats fed on a high-cholesterol diet. Biosci. Biotechnol. Biochem. 2008;72:2566–2571.10.1271/bbb.80257
- Chijimatsu T, Tatsuguchi I, Oda H, Mochizuki S. A freshwater clam (Corbicula fluminea) extract reduces cholesterol level and hepatic lipids in normal and xenobiotics-induced hypercholesterolaemic rats. J. Agric. Food Chem. 2009;57:3108–3112.10.1021/jf803308h
- Chijimatsu T, Umeki M, Okuda Y, Yamada K, Oda H, Mochizuki S. The fat and protein fractions of freshwater clam (Corbicula fluminea) extract reduce serum cholesterol and enhance bile acid biosynthesis and sterol excretion in hypercholesterolaemic rats fed a high-cholesterol diet. Br. J. Nutr. 2011;105:526–534.10.1017/S0007114510004058
- Chijimatsu T, Umeki M, Kataoka Y, Kobayashi S, Yamada K, Oda H, Mochizuki S. Lipid components prepared from a freshwater Clam (Corbicula fluminea) extract ameliorate hypercholesterolaemia in rats fed high-cholesterol diet. Food Chem. 2013;136:328–334.10.1016/j.foodchem.2012.08.070
- Laurent T, Okuda Y, Chijimatsu T, Umeki M, Kobayashi S, Kataoka Y, Tatsuguchi I, Mochizuki S, Oda H. Freshwater clam extract ameliorates triglyceride and cholesterol metabolism through the expression of genes involved in hepatic lipogenesis and cholesterol degradation in rats. Evid. Based Complement. Alternat. Med. 2013; Article ID 830684: http://dx.doi.org/10.1155/2013/830684.
- Folch J, Lees M, Sloane Stanley GH. A simple method for the isolation and purification of total lipides from animal tissues. J. Biol. Chem. 1957;226:497–509.
- Delaney B, Nicolosi RJ, Wilson TA, Carlson T, Frazer S, Zheng G, Hess R, Ostergren K, Haworth J, Knutson N. β-Glucan fractions from barley and oats are similarly antiatherogenic in hypercholesterolaemic Syrian golden hamsters. J. Nutr. 2003;133:468–475.
- Sheltawy MJ, Losowsky MS. Determination of fecal bile acids by an enzymatic method. Clin. Chim. Acta. 1975;64:127–132.10.1016/0009-8981(75)90194-1
- Chomczynski P, Sacchi N. Single-step method of RNA isolation by acid guanidinum thiocyanate-phenol-chloroform extraction. Anal. Biochem. 1987;162:156–159.10.1016/0003-2697(87)90021-2
- Oda H, Nozawa K, Hitomi Y, Kakinuma A. Laminin-rich extracellular matrix maintains high level of Hepatocyte nuclear factor 4 in rat Hepatocyte culture. Biochem. Biophys. Res. Commun. 1995;212:800–805.10.1006/bbrc.1995.2039
- Yoshida Y, Kimura N, Oda H, Kakinuma A. Insulin suppresses the induction of CYP2B1 and CYP2B2 gene expression by Phenobarbital in adult rat cultured hepatocytes. Biochem. Biophys. Res. Commun. 1996;229:182–188.10.1006/bbrc.1996.1777
- Tukey JW. Comparing individual means in the analysis of variance. Biometrics. 1949;5:99–114.10.2307/3001913
- Zangar RC, Davydov DR, Verma S. Mechanisms that regulate production of reactive oxygen species by cytochrome P450. Toxicol. Appl. Pharmacol. 2004;199:316–331.10.1016/j.taap.2004.01.018
- Fadhel Z, Lu Z, Robertson LW, Glauert HP. Effect of 3,3′,4,4′-tetrachlorobiphenyl and 2,2′,4,4′,5,5′-hexachlorobiphenyl on the induction of hepatic lipid peroxidation and cytochrome P-450 associated enzyme activities in rats. Toxicology. 2002;175:15–25.10.1016/S0300-483X(02)00086-0
- Glauert HP, Lu Z, Kumar A, Bunaciu RP, Patel S, Tharappel JC, Stemm DN, Lehmler HJ, Lee EY, Robertson LW, Spear BT. Dietary vitamin E does not inhibit the promotion of liver carcinogenesis by polychlorinataed biphenyls in rats. J. Nutr. 2005;135:283–286.
- Sen CK, Packer L. Antioxidant and redox regulation of gene transcription. FASEB J. 1996;10:709–720.
- Baeuerle PA, Henkel T. Functional and activation of NF-κB in the immune system. Annu. Rev. Immunol. 1994;12:141–179.10.1146/annurev.iy.12.040194.001041
- Iritani N, Fukuda E, Inoguchi K, Tsubosaka M, Tashiro S. Reduction of lipogenic enzymes by shellfish triglycerides in rat liver. J. Nutr. 1980;110:1664–1670.
- Svegliati-Baroni G, Candelaresi C, Saccomanno S, Ferretti G, Bachetti T, Marzioni M, De Minicis S, Nobili L, Salzano R, Omenetti A, Pacetti D, Sigmund S, Benedetti A, Casini A. A model of insulin resistance and nonalcoholic steatohepatitis in rats. Role of peroxisome proliferator-activated receptor-α and n-3 polyunsaturated fatty acid treatment on liver injury. Am. J. Pathol. 2006;169:846–860.10.2353/ajpath.2006.050953
- Iritani N, Nagashima K, Fukuda H, Katsurada A, Tanaka T. Effects of dietary proteins on lipogenic enzymes in rat liver. J. Nutr. 1986;116:190–197.
- Iritani N, Hoshomi H, Fukuda H, Tada K, Ikeda H. Soybean protein suppresses hepatic lipogenic enzyme gene expression in Wistar fatty rats. J. Nutr. 1996;126:380–388.
- Shimizu M, Tanabe S, Morimatsu F, Nagao K, Yanagita T, Kato N, Nishimura T. Consumption of Pork-liver protein Hydrolysate reduces body fat in Otsuka Long-evans Tokushima fatty rats by suppressing Hepatic Lipogenesis. Biosci. Biotechnol. Biochem. 2006;70:112–118.10.1271/bbb.70.112
- Komano T, Yokoyama FR, Funakoshi R, Egashira Y, Sanada H. Mechanism of the suppression against D-galactosamine-induced hepatic injury by dietary amino acids in rats. Amino Acids. 2009;37:239–247.10.1007/s00726-008-0139-1
- Hafidi ME, Pérez I, Zamora J, Soto V, Carvajal-Sandoval G, Baños G. Glycine intake decreases plasma free fatty acids, adipose cell size, and blood pressure in sucrose-fed rats. Am. J. Physiol. Regul. Integr. Comp. Physiol. 2004;287:R1387–R1393.10.1152/ajpregu.00159.2004
- Park T, Oh J, Lee K. Dietary taurine or glycine supplementation reduces plasma and liver cholesterol and triglyceride concentrations in rats fed a cholesterol-free diet. Nutr. Res. 1999;19:1777–1789.10.1016/S0271-5317(99)00118-9
- Stachlewitz RF, Seabra V, Bradford B, Bradham CA, Rusyn I, Germolec D, Thurman RG. Glycine and uridine prevent D-galactosamine hepatotoxicity in the rat: role of Kupffer cells. Hepatology. 1999;29:737–745.10.1002/(ISSN)1527-3350
- Wheeler MD, Ikejema K, Enomoto N, Stacklewitz RF, Seabra V, Zhong Z, Yin M, Schemmer P, Rose ML, Rusyn I, Bradford B, Thurman RG. Glycine: a new anti-inflammatory immunonutrient. Cell Mol. Life Sci. 1999;56:843–856.10.1007/s000180050030
- Ascencio C, Torres N, Isoard-Acosta F, Gómez-Pérez FJ, Hernández-Pando R, Tovar AR. Soy protein affects serum insulin and hepatic SREBP-1 mRNA and reduces fatty liver in rats. J. Nutr. 2004;134:522–529.
- Nagaoka S, Masaki H, Aoyama Y, Yoshida A. Effects of excess dietary tyrosine or certain xenobiotics on the cholesterogenesis in rats. J. Nutr. 1986;116:726–732.