Abstract
Purified recombinant sorbose dehydrogenase from Sinorhizobium sp. 97507 exhibited high reactivity for 1,5-anhydro-d-glucitol (1,5-AG) and l-sorbose, but little activity for the other sugars or sugar alcohols tested. Kinetic analysis revealed that its catalytic efficiency (kcat/Km) for l-sorbose and 1,5-AG is 1.8 × 102 and 1.5 × 102 s−1·M−1, respectively.
Sorbose dehydrogenase [SDH (EC 1.1.99.32)] catalyzes the oxidation of l-sorbose to l-sorbosone, a precursor of 2-keto-l-gulonic acid, in the l-ascorbic acid (vitamin C) production pathway.Citation1) The enzymatic properties of membrane-bound SDH from Gluconobacter melanogenus UV10 (GmSDH) have been described by Sugisawa et al.Citation2). The enzyme showed high substrate specificity for l-sorbose, but did not exhibit activity for any other sugars or sugar alcohols tested. 1,5-anhydro-d-glucitol (1,5-AG) is a six-carbon monosaccharide of glucopyranose with a 1-deoxy form (Fig. ). Reactivity of SDH from Sinorhizobium sp. for 1,5-AG has not been reported. 1,5-AG is found in bacteria, animals, and plants. 1,5-AG is considered one of the main polyols in human blood.Citation3) In addition, in recent years, 1,5-AG has also been used as a marker for glycemic control, because it responds sensitively to changes in blood glucose level.Citation4) The concentration of 1,5-AG in human blood samples is determined by biochemical methods using enzymes that exhibit activity for 1,5-AG. Pyranose oxidase from Polyporus obtusus, which oxidizes the hydroxyl group of pyranose at position C-2, is widely used for 1,5-AG measurement.Citation5) Enzymes that oxidize 1,5-AG have been identified in several microorganisms. Dehydrogenases that oxidize 1,5-AG exist in Agrobacterium sp.,Citation6) Rahnella aquatilis,Citation7) Eupenicillium crustaceum,Citation8) Cytophaga marinoflava,Citation9) and Trichoderma longibrachiatum.Citation10) In this study, we describe the enzymatic properties of recombinant SDH from Sinorhizobium sp. 97507 (SiSDH) and the potential applications of the purified enzyme in the measurement of 1,5-AG concentration.
We amplified a putative sdh gene by polymerase chain reaction (PCR) using Sinorhizobium sp. 97507 as the source of genomic DNA. Specific PCR primer sets were designed from the nucleotide sequence of the SMa1414 gene, which exists in the pSymA megaplasmid of the symbiotic, nitrogen-fixing soil bacterium Sinorhizobium meliloti 1021.Citation11) The SMa1414 gene encodes a flavin adenine dinucleotide-dependent dehydrogenase, and its amino acid sequence is 73% identical to that of SDH (EC 1.1.99.32; UniProt Accession Number: Q47944.1) from Gluconobacter oxydans.Citation12) The putative sdh gene (GenBank Accession Number: LC012351) was subcloned into the expression plasmid pET-21a (Novagen, Inc., Madison, WI, USA) using the In-Fusion System. DNA sequencing analysis revealed that the PCR product was identical to SMa1414, except that the Met54 residue of SMa1414 was Leu in the putative sdh gene from Sinorhizobium sp. 97507. E. coli BL21 (DE3) cells harboring the pET-21a-SiSDH plasmid were aerobically cultivated in Terrific Broth medium.Citation13) After the cell-free extract, prepared from harvested cells, was solubilized in Triton X-100, the sample was purified by affinity chromatography using TALON Metal Affinity Resin (Takara Bio Inc., Otsu, Japan). Following ultracentrifugation of the cell-free extract, the activity of the supernatant solution was approximately 15% that of the original enzyme (approximately 85% of the enzyme activity remained in the precipitate, which included the membrane fraction). This result suggests that SiSDH is a membrane-bound protein adherent to the inner cell membrane, consistent with the description of GmSDH by Sugisawa et al. Citation2). Enzyme activity was detected by a colorimetric method using 1-methoxy-5-methylphenazinium methyl sulfate and 2,6-dichlorophenolindophenol (DCIP) at 37 °C. The specific activity of purified SiSDH was 12 U mg−1 of protein at a yield of 28%. The homogeneity of SiSDH was confirmed by sodium dodecyl sulfate-polyacrylamide gel electrophoresis analysis; the molecular mass of the subunit was approximately 59 kDa (Supplementary Fig. 1). A gel filtration experiment using prepurified SiSDH showed two peaks of approximately 150 and 672 kDa. Enzyme activity was confirmed in both fractions. An enzyme activity of 64% of the original enzyme was detected for the 150 kDa fraction. Therefore, it appears likely that SiSDH forms a homodimer. The purified enzyme was unstable, and 30% of enzyme activity was lost after storage at 4 °C for 2 days. Next, we examined the effects of pH and temperature on activity and stability. The optimum pH and temperature of SiSDH were 8.5 and 40 °C, respectively (Supplementary Fig. 2). No decrease in activity was observed in the pH range 5.5–8.0 (Supplementary Fig. 3A). The enzyme was stable at temperatures of up to 30 °C, and 82% of enzyme activity persisted at 35 °C. Enzyme activity was almost completely abrogated by a 10 min incubation at 55 °C (Supplementary Fig. 3B).
SiSDH was highly specific for l-sorbose and 1,5-AG (Table ). Activities for other substrates tested were less than 2% of that for l-sorbose. We examined the kinetic parameters of SiSDH using l-sorbose and 1,5-AG. The Km and kcat values for l-sorbose were 62.4 mM and 11.2 s−1, respectively, whereas those for 1,5-AG were 97.5 mM and 14.1 s−1, respectively. The resulting catalytic efficiencies (kcat/Km) of SiSDH for l-sorbose and 1,5-AG were 1.8 × 102 and 1.5 × 102 s−1·M−1, which were similar. Sugisawa et al.Citation2) reported that purified GmSDH has a Km value of 100 mM for l-sorbose with DCIP as the electron acceptor. Both SDHs exhibited a similar Km value for l-sorbose. However, the Km values of SiSDH for l-sorbose and 1,5-AG are considered high compared with intracellular enzymes in general. Therefore, these sugars may not be natural substrates of SiSDH.
Table 1. Substrate specificity of purified recombinant sorbose dehydrogenase.
Subsequently, we attempted to determine 1,5-AG concentration using purified SiSDH under standard conditions. Fig. shows the correlation between optical density at 600 nm and 1,5-AG concentration. A linear correlation was observed between 1,5-AG concentration and SiSDH activity within a range of 0.5–5 mM. However, the measurement range of 1,5-AG in clinical practice is approximately 0.006–0.3 mM. Therefore, SiSDH is unsuitable for detecting low concentrations of 1,5-AG, and larger amounts of SiSDH may be necessary to quantify low 1,5-AG concentrations. Because 1,5-AG dehydrogenases reportedly have a low Km value (0.5–6.6 mM),Citation6,9,10) these enzymes are capable of measuring low 1,5-AG concentrations with smaller amounts of enzyme compared with SiSDH. Therefore, the Km value of SiSDH is a disadvantage for 1,5-AG measurement. However, SiSDH shows reactivity for only l-sorbose and 1,5-AG, and it is possible that SiSDH may be used for 1,5-AG measurement after improvement of its Km value.
Fig. 2. Calibration curve of 1,5-anhydro-d-glucitol.
Note: Enzyme activities were measured under standard conditions at various concentrations of 1,5-anhydro-d-glucitol.
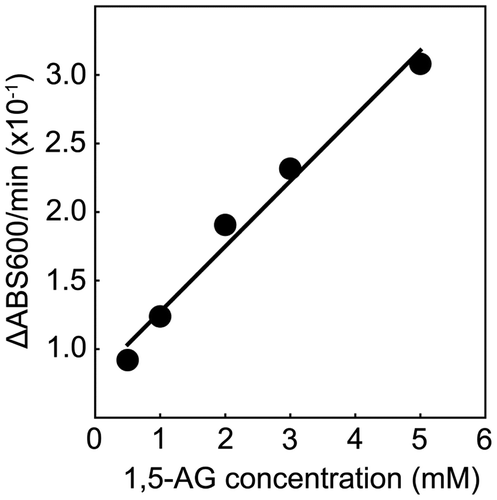
On the basis of substrate specificity, SDHs can be divided into two groups: Type 1 SDHs (EC 1.1.99.12), which react with the C-5 hydroxyl group of l-sorbose and Type 2 SDHs, such as GmSDH (EC 1.1.99.32),Citation2) which react with the C-1 hydroxyl group of l-sorbose. Pyrroloquinoline quinone (PQQ)-dependent l-sorbose/l-sorbosone dehydrogenase from G. oxydans DSM 4025 was reported to be a Type 1 SDH by Asakura and Hoshino.Citation14) The enzyme exhibits broad substrate specificity, and reacts with sugars, sugar alcohols, and alcohols. The enzyme also converts d-sorbitol to l-sorbose and d-glucose. Therefore, G. oxydans DSM 4025 PQQ-dependent l-sorbose/l-sorbosone dehydrogenase is capable of catalyzing the oxidation of both OH groups at the C-1 and C-5 positions of d-sorbitol. SiSDH recognizes pyranose rings, exhibits no activity for alcohols, such as ethanol, 1-propanol, and 1-butanol (data not shown), and shares high (73%) homology with SDH (EC 1.1.99.32) from G. oxydans.Citation12) Accordingly, it is probable that, rather than a Type 1 SDH like PQQ-dependent l-sorbose/l-sorbosone dehydrogenase, SiSDH is a Type 2 SDH, capable of reacting with the C-1 hydroxyl group of l-sorbose. Although SiSDH appears to react with the hydroxyl group of l-sorbose at position C-1, 1,5-AG does not contain a corresponding OH group at position C-1 (Fig. ). Considering our findings regarding SiSDH reactivity for l-sorbose and 1,5-AG, SiSDH must react with a hydroxyl group of 1,5-AG. SiSDH may catalyze the regioselective oxidation of a hydroxyl group of the pyranose ring. It is established that pyranose 2-oxidase from Trametes multicolor oxidizes d-glucose at the C-2 position and 2-keto-d-glucose at the C-3 position by rotation of the substrate in the active site.Citation15) Therefore, the mechanism of action of SiSDH may be elucidated when its X-ray crystal structure is solved. Further study is required to identify the site at which SiSDH acts on 1,5-AG.
Supplemental material
The supplemental material for this paper is available at http://dx.doi.org/10.1080/09168451.2015.1012148.
1012148.zip
Download Zip (254.5 KB)Notes
Abbreviations:1,5-AG, 1,5-anhydro-d-glucitol; AGDs, 1,5-AG dehydrogenases; DCIP, 2,6-dichlorophenolindophenol; GmSDH, Gluconobacter melanogenus UV10 membrane-bound SDH; PCR, polymerase chain reaction; PQQ, pyrroloquinoline quinone; SDH, sorbose dehydrogenase; SiSDH, Sinorhizobium sp. 97507 recombinant SDH.
References
- Hancock RD, Viola R. Biotechnological approaches for l-ascorbic acid production. Trends Biotechnol. 2002;20:299–305.10.1016/S0167-7799(02)01991-1
- Sugisawa T, Hoshino T, Nomura S, Fujiwara A. Isolation and characterization of membrane-bound-l-sorbose dehydrogenase from Gluconobacter melanogenus UV10. Agric. Biol. Chem. 1991;55:363–370.10.1271/bbb1961.55.363
- Yamanouchi T, Tachibana Y, Akanuma H, Minoda S, Shinohara T, Moromizato H, Miyashita H, Akaoka I. Origin and disposal of 1,5-anhydroglucitol, a major polyol in the human body. Am. J. Physiol. 1992;236:E268–E273.
- McGill JB, Ammirati EB, Cole TG, Gautille T, Nowatzke W, Sarno MJ, Houghton S. Circulating 1,5-Anhydroglucitol levels in adult patients with diabetes reflect longitudinal changes of glycemia. Diabetes Care. 2004;27:1859–1865.10.2337/diacare.27.8.1859
- Fukumura Y, Tajima S, Oshitani S, Ushijima Y, Kobayashi I, Hara F, Yamamoto S, Yabuuchi M. Fully enzymatic method for determining 1,5-anhydro-d-glucitol in serum. Clin. Chem. 1994;40:2013–2016.
- Ebinuma H, Ushizawa K, inventor; Sekisui medical Co., Ltd, assigneee. 1,5-anhydroglucitol dehydrogenase, it production. Japan patent 2886846. 1999 Feb 12.
- Uchida M, Kondo H, inventor; Unitika Ltd, assignee. 1,5-anhydroglucitol dehydrogenase, it production. Japan patent 1999-018762. 1999 Jan 26.
- Nishii K, Sato S, Nakamura T, inventor; Nippon kayaku Co., Ltd, Japan patent 2665681. 1997 Jun 27.
- Tsugawa W, Horiuchi S, Tanaka M, Wake H, Sode K. Purification of a marine bacterial glucose dehydrogenase from Cytophaga marinoflava and its application for measurement of 1,5-anhydro-d-glucitol. Appl. Biochem. Biotechnol. 1996;56:302–310.
- Yoshida N, Uchida E, Katsuragi T, Tani Y. A novel NAD-dependent dehydrogenase, Highly specific for 1,5-Anhydro-d-glucitol, from Trichoderma longibrachiatum strain 11-3. Appl. Environ. Microbiol. 2003;69:2603–2607.10.1128/AEM.69.5.2603-2607.2003
- Barnett MJ, Fisher RF, Jones T, Komp C, Abola AP, Barloy-Humbler FR, Bowser L, Capela D, Galibert F, Gouzy JR, Gurjal M, Hong A, Huizar L, Hyman RW, Kahn D, Kahn ML, Kalman S, Keating DW, Palm C, Peck MC, Surzycki R, Wells DH, Yeh K, Davis RW, Federspiel NA, Long SR. Nucleotide sequence and predicted functions of the entire Sinorhizobium meliloti pSymA megaplasmid. Proc. Natl. Acad. Sci. USA. 2001;98:9883–9888.10.1073/pnas.161294798
- Saito Y, Ishii Y, Hayashi H, Imao Y, Akashi T, Yoshikawa K, Noguchi Y, Soeda S, Yoshida M, Niwa M, Hosoda J, Shimomura K. Cloning of genes coding for l-sorbose and l-sorbosone dehydrogenases from Gluconobacter oxydans and microbial production of 2-keto-l-gulonate, a precursor of l-ascorbic acid, in a recombinant G. oxydans strain. Appl. Environ. Microbiol. 1997;63:454–460.
- Tartoff KD, Hobbs CA. Improved media for growing plasmid and cosmid clones. Bethesda Res. Lab. Focus. 1987;9:12.
- Asakura A, Hoshino T. Isolation and characterization of a new quinoprotein dehydrogenase, l-sorbose/l-sorbosone dehydrogenase. Biosci. Biotechnol. Biochem. 1999;63:46–53.10.1271/bbb.63.46
- Kujawa M, Ebner H, Leitner C, Hallberg BM, Prongjit M, Sucharitakul J, Ludwig R, Rudsander U, Peterbauer C, Chaiyen P, Haltrich D, Divne C. Structural basis for substrate binding and regioselective oxidation of monosaccharides at C3 by pyranose 2-oxidase. J. Biol. Chem. 2006;281:35104–35115.10.1074/jbc.M604718200