Abstract
Konjac-mannan has been suggested to exert a protective effect against visceral obesity. However, there is little knowledge about the effects of liquid konjac (LK) that is partially alkali gelled. The purpose of the present work was to evaluate the potential beneficial effects of a LK powder on obesity in mice. Male C57BL/6J mice were fed a high-fat diet supplemented with either 2.5 or 5% LK powder for 80 days. Growth parameters, abdominal fat content, serum biochemical markers, and hepatic lipid accumulations were measured. Dietary supplementation with LK resulted in decreased body weight gain and abdominal fat accumulation. Dose-dependent decreases were observed in accumulation of hepatic lipids and serum total cholesterol, leptin, insulin concentrations. The study findings indicated that LK had preventing effect against obesity, including the reduction of abdominal and hepatic lipid accumulation and serum parameters related to obesity.
Graphical abstract
Body weight (upper) and mesenteric fat (lower) in mice fed the test diets for 80 days. Error bars represent SD. Different superscript letters are significantly different (p < 0.05).
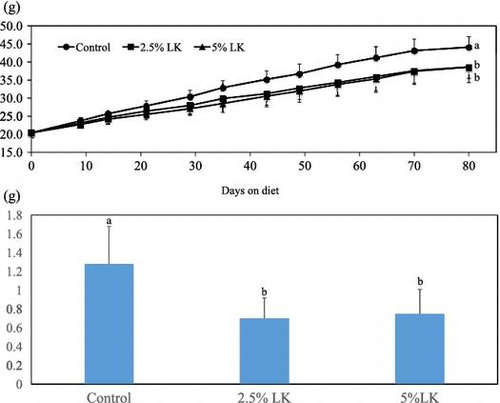
Key words:
Edible “Konjac” (Amorphophallus konjac) is generally prepared by mixing konjac flour with water, thereby gelling it at pH 10.5–12. Dietary fiber in edible konjac gel is insoluble, whereas glucomannan in intact konjac is soluble.Citation1) Therefore, the beneficial effects of glucomannan in konjac flour for health are normally lost in alkali-gelled konjac.Citation1) To ameliorate this reduction in soluble glucomannan, a liquid konjac (LK) was developed by a commercial manufacturer.Citation2) To produce LK, konjac powder is swollen with water and treated with an alkali at pH 10 or above and then, after lowering the pH value to 8 or below, treated with mixed enzymes including cellulase, pectinase, and protease while lowering the temperature under vigorous stirring. Thus, it is possible to create a composition that sustains the gelling power and biological functions of glucomannan and enables more homogeneous mixing of a konjac material at a higher concentration than in other food materials. This LK is considered to be partially alkali-gelled konjac, which means that LK consists of both soluble and insoluble dietary fiber.
It has been reported that glucomannan reduces the postprandial rise in plasma glucose,Citation3–7) improves lipid metabolism,Citation8–11) reduces body weightCitation12,13) in human experiments, suppresses hepatic cholesterol synthesis, and increases the fecal excretion of cholesterol and bile acids in animal experiments.Citation14,15) A previous meta-analysis showed that glucomannan appears to beneficially affect plasma lipids, body weight, and fasting blood glucose.Citation16,17) However, whether LK has the same beneficial effects is not known.
The purpose of this study was to investigate the effects of LK on abdominal fat accumulation, and lipid and glucose metabolism in diet-induced obese mice fed a high-fat diet.
Materials and methods
Sample preparation and chemical analysis
LK “NANOKON” was obtained from Konnyakuya-Honpo Co., Ltd (Saitama, Japan). After freeze-drying, 6.6 g of freeze-dried powder was obtained from 100 g of LK. The soluble and insoluble dietary fiber contents of LK powder were analyzed by the method of Prosky et al.Citation18) and were determined to be 38 and 23%, respectively. The viscosity of the 1% solution of LK was determined in vitro by a rotational viscometer (Viscotester VT-04F, RION Co., Ltd, Tokyo, Japan) at 35 °C. The viscosity of 1% LK solution was 35 mPa s, whereas that of the 1% solution of non-treated glucomannan was 50,000 mPa s.
Animals and study design
Male C57BL/6 J mice, 4 week old, were purchased from Charles River (Charles River Laboratories Japan, Inc., Yokohama, Japan). The animals were maintained in a temperature-controlled room on a 12-h light/dark cycle (light was switched on at 08:00 h). The studies were approved by the Otsuma Women’s University Animal Research Committee (Tokyo, Japan) and are in accordance with the Regulation on Animal Experimentation at Otsuma Women’s University. After 7-days acclimatization, the mice were randomized to three groups (n = 8 per group) and shifted to a high-fat diet supplemented with 0 (control), 2.5, or 5% LK powder. The experimental diets were also supplemented with cellulose as necessary to adjust the total dietary fiber content to 5%. Compositions of the experimental diets are shown in Table . Mice were fed the experimental diets ad libitum for 80 days. Food intake and body weights were monitored three times a week throughout the study period. Feces were collected for 7 days before sacrifice, washed with distilled water, and then freeze-dried, milled, and kept at −20 °C until analysis. At the end of the study, mice were fasted for 6 h before sacrifice, and then, the adipose tissue depots: retroperitoneal (including perirenal), mesenteric (including blood vessels and other connective tissues), epididymal, and liver were dissected and then weighed. Livers were freeze-dried, milled, and kept at −20 °C until analysis of lipids. Blood was withdrawn from the heart chambers under ether anesthesia. Blood samples were centrifuged, and serum was collected for analysis of lipids.
Table 1. Composition of the experimental diets (g/kg diet).
Analysis of serum and liver lipids
Serum total cholesterol, triglyceride, glucose, and free fatty acid concentrations were measured using an enzymatic procedure (cholesterol E-test WAKO, triglyceride E-test WAKO, glucose CII-test WAKO, and NEFA C-test WAKO, respectively; Wako Pure Chemical Industries, Osaka, Japan). Serum glycerol concentration was also measured using an enzymatic procedure (Glycerol assay kit, Cayman Chemical Company, MI, USA). Serum leptin, insulin, and adiponectin concentrations were measured using an ELISA kit (Mouse Leptin Immunoassay kit, R&D Systems, Inc. MN, USA; Mouse Insulin ELISA Kit and Mouse/Rat HMW Adiponectin ELISA Kit, Shibayagi Co. Ltd. Gunma, Japan). Homeostasis model assessment-estimated insulin resistance (HOMA-IR) was calculated according to the formula: fasting insulin (μIU) × fasting glucose (mg/dl)/405, where fasting insulin (μIU) = fasting insulin (ng/ml) × 26.
Liver lipids were extracted using chloroform–methanol solution (2:1, v/v) by the method of Folch et al.Citation19) and dissolved in isopropanol containing 10% polyoxyethylene octylphenyl ether (Triton X-100, Wako Pure Chemical Industries, Osaka, Japan). The concentration of liver cholesterol and triglyceride in the lipid extracts was measured enzymatically using cholesterol E-test and triglyceride E-test, respectively (Wako Pure Chemical Industries, Osaka, Japan).
Analysis of total lipids in feces
Feces were collected for 7 days before sacrifice and lyophilized. Total lipids in feces were extracted with a chloroform: methanol mixture (2:1. v/v) under the acidic condition (acetic acid was added to a final concentration of 4%).Citation20) Total lipids in the feces were determined gravimetrically.
Analysis of epididymal adipocyte size
Epididymal adipose tissues were fixed with 2% osmium tetroxide solution for 30 h at 37 °C (Wako Pure Chemical Industries, Osaka, Japan). Fixed adipocytes were collected with 25-μm nylon mesh after being passed through 250-μm mesh. Adipocyte size in epididymal fat was measured using a Coulter Counter (Multisizer 3, Beckman Coulter, Inc. CA, USA).
Oral glucose tolerance test
An oral glucose tolerance test (OGTT) was performed in mice after 6 h fasting on the 73rd day of dietary supplementation. Overnight fasting is commonly used in published studies for OGTTs in mice and rats.Citation21) However, the authors of several recent studies have expressed concern that overnight fasting in mice is not ideal because, as nocturnal animals, they consume most of their daily calories overnight. Andrikopoulos et al.Citation22) concluded that oral glucose administration after 6 h of fasting is best to assess glucose tolerance in mice. Blood glucose was measured using a blood glucose meter (Glutest Ace R, Sanwa Kagaku Kenkyusho Co., Ltd., Aichi, Japan) with blood taken from the tail tip at 0, 15, 30, 60, and 120 min after glucose administration. The glucose load was 1 g/kg orally.
Statistical analysis
Data were presented as mean ± standard deviation. Group means were compared by one-way analysis of variance. Means were considered to be significantly different (p < 0.05) as determined by the Tukey–Kramer honest significant difference test. A linear regression model was used to evaluate the potential dose-dependent effect of LK. Spearman’s rank correlation coefficient was calculated for the relationships among several parameters related to obesity, and the results are examined in the discussion. All statistical analyses were performed using JMP (Version 10.0, SAS Institute Inc., Cary, NC, USA) and IBM SPSS (Version 22.0, SPSS Inc., Chicago, IL, USA).
Results
Food intake, body weight, and weight of organs
There was no significant difference in food intake, but final weight, body weight gain, and food efficiency ratio in the LK groups were significantly different from those in the control group (p < 0.05, Table , Figs. and ). Significant dose-dependent effects of LK were also observed (p < 0.01). The organ weights of mice fed LK are shown in Table . Weights of the liver and mesenteric fat in both of the LK groups were significantly lower than those in the control group (p < 0.05). Retroperitoneal fat weight in the 5% LK group was significantly lower than that in the control group (p < 0.05). Significant dose-dependent effects of LK were observed in reducing liver, retroperitoneal, and mesenteric fat weights (p < 0.01).
Table 2. Body weight gain, food intake, and food efficiency ratio.
Fig. 1. Time-dependent changes in body weight in mice fed the test diets for 80 days.
Error bars represent standard deviation. Means with different superscript letters are significantly different p < 0.05).
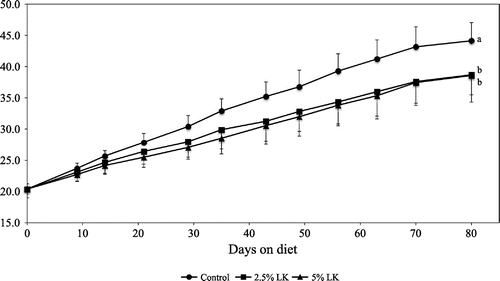
Fig. 2. Time-dependent changes in average daily food intake in mice fed the test diets for 80 days.
Error bars represent standard deviation.
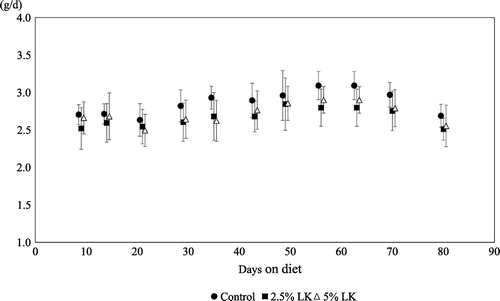
Table 3. Weight of organs.
Fecal total fat excretion and apparent absorption of fat
Fecal total fat excretion in both the LK groups was significantly higher than that in the control group, and apparent absorption of fat in both the LK groups was significantly lower than that in the control group (p < 0.05, Table ). Significant dose-dependent effects of LK on fecal total fat excretion and apparent absorption of fat were also observed (p < 0.01).
Table 4. Fecal fat excretion and apparent absorption of fat.
Adipocyte size
Average adipocyte size in epididymal fat is shown in Table . There were no significant differences in epididymal adipocyte size among the experimental groups.
Table 5. Average adipocyte size of epididymal fat.
Oral glucose tolerance test
Blood glucose levels and areas under the curves (AUC) in OGTT are shown in Table . The blood glucose level at 15 min in the 2.5% LK group was significantly lower than that in the control group (p < 0.05). No significant differences in glucose levels at other time points or AUC were observed among the groups.
Table 6. Blood glucose levels and AUC in OGTT.
Serum and liver lipids
Serum biochemical concentrations are shown in Table . Serum total cholesterol concentration in the 5% LK group was significantly lower than that in the control group (p < 0.05). There were no significant differences in serum triglyceride, free fatty acid, glycerol, and glucose concentrations among the experimental groups. Serum leptin concentration and HOMA-IR in the 5% LK group were significantly lower than those in the control group (p < 0.05). Serum insulin concentrations in both the LK groups were significantly lower than that in the control group (p < 0.05). Significant dose-dependent effects of LK were observed in modifying serum total cholesterol, glycerol, leptin, insulin (p < 0.01), and HOMA-IR (p < 0.05).
Table 7. Serum biochemical levels and hormone concentrations.
Liver lipid accumulation results are shown in Table . Liver cholesterol and triglyceride accumulation (mg/liver) in both the LK groups were significantly lower than those in the control group (p < 0.05). Liver triglyceride concentration (mg/g liver) in both the LK groups and liver cholesterol concentration (mg/g liver) in the 5% LK group were also significantly different from the respective parameter in the control group (p < 0.05). Significant dose-dependent effects of LK were observed for all liver parameters (p < 0.05).
Table 8. Liver lipid accumulation.
Discussion
We investigated the effects of LK on abdominal fat accumulation, lipid, and glucose metabolism in diet-induced obese mice fed a high-fat diet containing either 2.5 or 5% LK powder for 80 days. It is reported that the soluble and insoluble fiber contents in konjac flour were 73.3 and 6.6%, respectively,Citation23) whereas the soluble and insoluble dietary fiber contents in LK powder were 38 and 23%, respectively. Therefore, LK is considered to be a partially alkali-gelled glucomannan.
Growth data suggested that reductions in final body weight, body weight gain, and food efficiency ratio were observed in mice fed a diet containing more than 2.5% LK, and these effects were observed to be dose-dependent. These findings might be explained by reduction of lipid accumulation in liver and abdominal fat organs. The results were consistent with reduction of hepatic triglyceride and cholesterol accumulation in the LK-supplemented mice.
Significant reductions in mesenteric and retroperitoneal fat weight were observed with LK supplementation, but the mean adipocyte size in epididymal fat was not significantly changed. Whether response may differ between mesenteric, retroperitoneal, and epididymal fat tissues is not known. Further experiments are needed to clarify tissue-specific responses in abdominal fats. Table shows that total food intake was relatively low in the 2.5 and 5% LK groups compared with the 0% group (these differences were not statistically significant). Significant positive Spearman’s rank correlation coefficients were observed between total food intake and organ weights, serum insulin, and liver lipids. Therefore, total food intake was treated as a covariate and analyzed using an analysis of covariance test, which showed that the influence of LK supplementation on organ weights, serum insulin, and liver lipids remained statistically significant after adjustment for total food intake (results not shown).
Reduction in serum cholesterol concentrations was observed in LK-supplemented mice, and a significant difference was observed between the 0 and 5% LK groups. Similar results were observed for serum leptin concentration, consistent with the observed reduction in mesenteric fat accumulation. A dose-dependent reduction in serum insulin concentration was observed with LK supplementation, and significant differences were observed between the 0% group and each of the two LK supplementation groups (2.5 and 5% LK). A dose-dependent reduction in HOMA-IR calculated from serum insulin and glucose concentrations was also observed with LK supplementation, and a significant difference was observed between the 0 and 5% LK groups. The blood glucose level 15 min after oral glucose administration was significantly lower in the 2.5% LK group than in the 0% group. This result might be caused by the retardation of gastric emptying time in the LK supplementation groups. Further studies will be needed to clarify the influence of the LK on glucose tolerance.
A dose-dependent reduction in glycerol concentration was also observed with LK supplementation, and a significant positive Spearman’s rank correlation coefficient was observed between serum insulin and glycerol concentrations (r = 0.64, p < 0.01). Therefore, reduction of insulin release by LK supplementation may cause reduction of glycerol and fatty acids release to the blood. Lack of significant differences in the concentrations of free fatty acids among the experimental groups may be caused by their rapid incorporation into the liver. It has been reported that rapidly digested carbohydrate can result in large rises in insulin followed by rapid falls in blood glucose, leading to the suggestion that serum fatty acid release is stimulated resulting in impaired carbohydrate tolerance.Citation24)
It has previously been reported that the serum adiponectin concentration decreased progressively according to the severity of obesity.Citation25) However, in our study, the serum adiponectin concentration was relatively high in all groups, probably because of the early stage of obesity at which measurements were taken. Longer term feeding experiment is needed to fully assess the effect of LK on serum adiponectin concentration.
The reduction of hepatic lipid accumulation might be partially explained by apparent lipid digestibility,Citation26–28) but the other mechanisms such as short-chain fatty acid production by colonic fermentation should be also considered.Citation29) The reduction of total energy availability, including lowered carbohydrates, proteins, and lipids, may contribute to the reduced hepatic lipid accumulation. Further research will be needed to elucidate the mechanisms of the anti-obesity effects of LK and the improvement of lipid metabolism by LK feeding.
In conclusion, a preventive effect of a LK-enriched diet against obesity was observed, involving the reduction of abdominal fat accumulation and serum cholesterol concentrations and inhibition of lipid accumulation in the liver.
Funding
This work was supported by Konnyakuya-Honpo Co., Ltd (Saitama, Japan).
References
- Kiriyama S, Morisaki H, Yoshida A. Changes in hypocholesterolemic activity in rats by various konnyaku powder treatments. Agr. Biol. Chem. 1970;34:641–643.
- Sakurai S. U.S. Patent 7749550. 2010 Jul. 6.
- Doi K. Effect of konjac fibre (glucomannan) on glucose and lipids. Eur. J. Clin. Nutr. 1995;49:S190–S197.
- Keithley J, Swanson B. Glucomannan and obesity: a critical review. Altern. Ther. Health Med. 2005;11:30–34.
- Vuksan V, Sievenpiper JL, Owen R, Swilley JA, Spadafora P, Jenkins DJ, Vidgen E, Brighenti F, Josse RG, Leiter LA, Xu Z, Novokmet R. Beneficial effects of viscous dietary fiber from Konjac-mannan in subjects with the insulin resistance syndrome: results of a controlled metabolic trial. Diabetes Care. 2000;23:9–14.10.2337/diacare.23.1.9
- Vuksan V, Jenkins DJ, Spadafora P, Sievenpiper JL, Owen R, Vidgen E, Brighenti F, Josse R, Leiter LA, Bruce-Thompson C. Konjac-mannan (glucomannan) improves glycemia and other associated risk factors for coronary heart disease in type 2 diabetes. A randomized controlled metabolic trial. Diabetes Care. 1999;22:913–919.10.2337/diacare.22.6.913
- Chearskul S, Sangurai S, Nitiyanant W, Kriengsinyos W, Kooptiwut S, Harindhanavudhi T. Glycemic and lipid responses to glucomannan in Thais with type 2 diabetes mellitus. J. Med. Assoc. Thai. 2007;90:2150–2157.
- Yoshida M, Vanstone CA, Parsons WD, Zawistowski J, Jones PJH. Effect of plant sterols and glucomannan on lipids in individuals with and without type II diabetes. Eur. J. Clin. Nutr. 2006;60:529–537.10.1038/sj.ejcn.1602347
- Arvill A, Bodin L. Effect of short-term ingestion of konjac glucomannan on serum cholesterol in healthy men. Am. J. Clin. Nutr. 1995;61:585–589.
- Zhang MO, Huang CY, Wang X, Hong JR, Peng SS. The effect of foods containing refined Konjac meal on human lipid metabolism. Biomed. Environ. Sci. 1990;3:99–105.
- Chen HL, Huey-Herng Sheu W, Tai TS, Liaw YP, Chen YC. Konjac supplement alleviated hypercholesterolemia and hyperglycemia in type 2 diabetic subjects–a randomized double-blind trial. J. Am. Coll. Nutr. 2003;22:36–42.10.1080/07315724.2003.10719273
- Birketvedt GS, Shimshi M, Thom E, Florholmen J. Experiences with three different fiber supplements in weight reduction. Med. Sci. Monit. 2005;11:PI5–PI8.
- Walsh DE, Yaghoubian V, Behforooz A. Effect of glucomannan on obese patients: a clinical study. Int. J. Obes. 1984;8:289–293.
- Hozumi T, Yoshida M, Ishida Y, Mimoto H, Sawa J, Doi K, Kazumi T. Long-term effects of dietary fiber supplementation on serum glucose and lipoprotein levels in diabetic rats fed a high cholesterol diet. Endocr. J. 1995;42:187–192.10.1507/endocrj.42.187
- Hou YH, Zhang LS, Zhou HM, Wang RS, Zhang YZ. Influences of refined konjac meal on the levels of tissue lipids and the absorption of four minerals in rats. Biomed. Environ. Sci. 1990;3:306–314.
- Onakpoya I, Posadzki P, Ernst E. The efficacy of glucomannan supplementation in overweight and obesity: a systematic review and meta-analysis of randomized clinical trials. J. Am. Coll. Nutr. 2014;33:70–78.10.1080/07315724.2014.870013
- Sood N, Baker WL, Coleman CI. Effect of glucomannan on plasma lipid and glucose concentrations, body weight, and blood pressure: systematic review and meta-analysis. Am. J. Clin. Nutr. 2008;88:1167–1175.
- Prosky L, Asp NG, Schweizer TF, DeVries JW, Furda I. Determination of insoluble, soluble, and total dietary fiber in foods and food products: interlaboratory study. J. Assoc. Off. Anal. Chem. 1988;71:1017–1023.
- Folch J, Lees M, Sloane-Stanley GH. A simple method for the isolation and purification of total lipids from animal tissues. J. Biol. Chem. 1962;226:497–509.
- Kojima M, Arishima T, Shimizu R, Kohno M, Kida H, Hirotsuka M, Ikeda I. Consumption of a structured triacylglycerol containing behenic and oleic acids increases fecal fat excretion in humans. J. Oleo Sci. 2013;62:997–1001.10.5650/jos.62.997
- Muniyappa R, Lee S, Chen H, Quon MJ. Current approaches for assessing insulin sensitivity and resistance in vivo: advantages, limitations, and appropriate usage. Am. J. Physiol. Endocrinol. Metab. 2008;294:E15–E26.
- Andrikopoulos S, Blair AR, Deluca N, Fam BC, Proietto J. Evaluating the glucose tolerance test in mice. Am. J. Physiol. Endocrinol. Metab. 2008;295:E1323–E1332.10.1152/ajpendo.90617.2008
- Ministry of education, culture, sports science and technology of Japan - Standard Tables of Food Composition in Japan. Available fromhttp://www.mext.go.jp/b_menu/shingi/gijyutu/gijyutu3/toushin/05031802/002.htm, [cited 2014 Sep 30].
- Venn BJ, Mann JI. Cereal grains, legumes and diabetes. Eur. J. Clin. Nutr. 2004;58:1443–1461.10.1038/sj.ejcn.1601995
- Nigro E, Scudiero O, Monaco ML, Palmieri A, Mazzarella G, Costagliola C, Bianco A, Daniele A. New insight into adiponectin role in obesity and obesity-related diseases. Biomed. Res. Int. 2014;2014:1–14.10.1155/2014/658913
- Levrat-Verny MA, Behr S, Mustad V, Rémésy C, Demigné C. Low levels of viscous hydrocolloids lower plasma cholesterol in rats primarily by impairing cholesterol absorption. J. Nutr. 2000;130:243–248.
- Shimizu H, Yamauchi M, Kuramoto T, Kubota N, Matsuda M, Hoshita T. Effects of dietary konjac mannan on serum and liver cholesterol levels and biliary bile acid composition in hamsters. J. Pharmacobiodyn. 1991;14:371–375.10.1248/bpb1978.14.371
- Kiriyama S, Enishi A, Yura K. Inhibitory effect of konjac mannan on bile acid transport in the everted sacs from rat ileum. J. Nutr. 1974;104:69–78.
- Venter CS, Vorster HH, Cummings JH. Effects of dietary propionate on carbohydrate and lipid metabolism in healthy volunteers. Am. J. Gastroenterol. 1990;85:549–553.