Abstract
We investigated the effects of dietary iron deficiency on the redox system in the heart. Dietary iron deficiency increased heart weight and accumulation of carbonylated proteins. However, expression levels of heme oxygenase-1 and LC3-II, an antioxidant enzyme and an autophagic marker, respectively, in iron-deficient mice were upregulated compared to the control group, resulting in a surrogate phenomenon against oxidative stress.
Iron is an essential trace metal for almost all organisms, as it is one of the major heavy metal constituents of enzymes and has biochemical manifestations related to concentrations of some cytochromes in many tissues.Citation1) Excess iron, however, causes oxidative stress through the production of hydroxyl radicals via the Fenton/Haber–Weiss catalytic reaction, which causes tissue damage.Citation2) The level of intracellular iron is regulated by iron transporters and iron-binding proteins under normal physiological conditions.Citation3)
Iron deficiency is a worldwide nutritional problem in more than two billion people, mainly women and children.Citation4) In particular, the iron status of an individual may play an important role in cardiovascular health, not only via excess iron but also via iron deficiency, both of which can lead to significant problems.Citation5,6) Iron deficiency has been considered a common cause of blood loss and anemia.Citation6,7) Our research group previously reported that phosphatidylcholine hydroperoxide (PCOOH), a primary peroxide of biological membranes, is increased in iron-deficient rat liver with copper accumulation,Citation8) which may be due to the flexible coordination chemistry and redox reactivity of iron.Citation1,9) Therefore, the health consequences of anemia are manifold and include increased mortality rates and decreased physical capacity. Accordingly, it is possible that iron deficiency participates in induction of oxidative stress in many tissues, whereas little information is available on the molecular mechanisms underlying the relationship between oxidative stress and heart tissue in iron-deficient conditions. In this study, we focused on the effects of dietary iron deficiency on the redox system in heart tissue and investigated whether the redox system is activated in heart by measuring anti-oxidative markers in mice fed an iron-deficient diet.
Twelve 4-week-old male BALB mice were purchased from Clea Japan (Tokyo, Japan). The Animal Study Committee of the Tokyo University of Agriculture approved this study, and the animals were maintained in accordance with the guidelines of the Committee for the Care and Use of Laboratory Animals of the Tokyo University of Agriculture. After an adaptation period, the mice were randomly divided into two groups: a control group (CTL, n = 6) and an iron deficiency group (ID, n = 6). Mice in the CTL and ID groups were fed AIN-93G diet with or without iron for 4 weeks, ad libitum. After 4 weeks of treatment, all mice were sacrificed by exsanguination, and the blood was analyzed for hemoglobin concentration (Hemoglobin B-test, Wako Pure Chemical Industries, Osaka, Japan). Hearts were removed and weighed, because heart enlargement is one sign of iron-deficient anemia.Citation6) Hearts were homogenized in homogenization buffer (10 mM HEPES (pH 7.6), 1 mM EDTA, 250 mM sucrose, and phosphatase and protease inhibitor cocktails). The samples were resolved by SDS-PAGE and transferred to PVDF membrane (Millipore, Bedford, MA) and were developed using HRP-conjugated anti-rabbit or anti-mouse IgG antibodies and visualized using the GE Healthcare ECL System (Piscataway, NJ). Primary antibodies against Nrf2 (C-20) and actin (C-2) were obtained from Santa Cruz Biotechnology (Dallas, TX). Antibody against LC3-II was from MBL (Woburn, MA). Extraction of total RNA and synthesis of complementary DNA were performed as described previously.Citation10) Quantitative PCR primers and hybridization probes were synthesized for heme oxygenase-1 (HO-1), copper/zinc superoxide dismutase (Cu/ZnSOD), and manganese superoxide dismutase (MnSOD).Citation10) The results are expressed as the mean ± SEM for each group of six mice. After conducting an F-test to determine the homogeneity of values, Student’s t-test was used to determine significant differences between the two groups. Significant differences were considered at a p value of less than 0.05.
In this study, lower final body weight and food intake were observed in the ID group compared to the CTL group, but there were no significant differences in those two parameters. A previous study has shown that an iron-deficient diet decreases hemoglobin concentration and increases heart weight.Citation6) Indeed, this study also indicated that hemoglobin concentration was significantly lower in the ID group than in the CTL group. Heart weight was significantly higher in the ID group than in the CTL group (Table ). There was no significant difference in the liver and kidney weight between the two groups. Therefore, these data suggest that iron-deficient anemia occurred in mice fed the iron-deficient diet.
Table 1. Body weight; food intake; hemoglobin concentration; heart, liver, and kidney weights in mouse fed a control diet or an iron-deficient diet.
Reactive oxygen species (ROS) include superoxide anion, hydrogen peroxide, and hydroxyl radicals, which impair cardiac function and increase susceptibility to cardiac arrhythmia through direct toxic effects, resulting in increased necrosis and apoptosis of cardiomyocytes.Citation11) Additionally, PCOOH is significantly increased in iron-deficient rat liver.Citation8) Therefore, it is possible that oxidative stress is increased not only in liver but also in heart by an iron-deficient diet. We examined whether iron deficiency affects protein oxidation in the heart. Although we did not observe a robust increase in total protein carbonyl content in the CTL group, carbonyl oxidation of proteins was detected in the ID group (Fig. (A)). To determine whether the results from the animal study indicate a direct effect of iron deficiency, we treated NIH 3T3 cells with the iron-specific chelator deferoxamine (DFO) and evaluated the proteins for carbonyl oxidation status. As shown in Fig. (B), DFO dose-dependently increased carbonyl oxidation of proteins. These results suggest that iron deficiency strongly induced oxidative stress in vivo and in vitro.
Fig. 1. Effects of iron-deficiency on oxidation of proteins and autophagy.
Notes: (A) Mouse heart tissues and per mice were homogenized with fourfold of homogenization buffer, after which Western blot analyses were performed to detect the carbonyl proteins using anti-DNP antibody and an autophagic marker, LC3-II antibody. (B) Western blots of 100 μM DFO-treated and DFO-untreated NIH 3T3 cells for 24 h lysates probed for DNP and LC3-II. β-Actin was used as internal control.
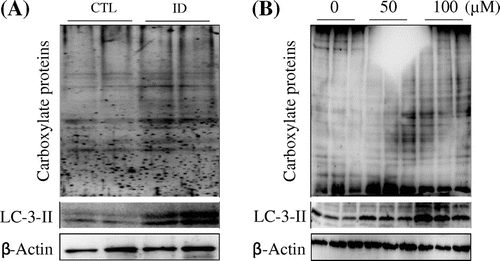
A previous study has indicated that ROS play crucial roles not only as signaling messengers in cell survival and death, but also as signals for the activation of autophagy under oxidative stress.Citation12) Thus, we examined the expression level of LC3-II, which is an autophagy marker, in heart and DFO-treated NIH 3T3 cells. The results show that LC3-II was dramatically increased in the hearts of ID mice and DFO-treated NIH 3T3 cells compared to the CTL group (Fig. (A) and (B), lower panel).
The transcription factor, nuclear factor-E2-related factor-2 (Nrf2), functions as a cellular sensor for oxidative stress and is sequestered in the cytosol by Kelch-like ECH-associated protein 1 (Keap1). In addition, Nrf2 is a critical regulator for maintaining the functional integrity of the heart under abnormally stressful conditions such as hemodynamic stress.Citation13) To determine the effect of iron deficiency on Nrf2 activity, nuclei were extracted from the mice heart tissue. Fig. (A) shows that nuclear Nrf2 accumulation in the ID group was higher than in the CTL group. Subsequently, Nrf2 plays crucial roles in antioxidant responsive element-mediated induction of anti-oxidative stress genes.Citation14) In this study, expression levels of HO-1, which is a detoxifying enzyme regulated by Nrf2, were significantly increased in the ID group compared to the CTL group (Fig. (B)). Additionally, Keap1 is constitutively degraded by autophagy for the maintenance of redox homeostasis.Citation15) Therefore, iron deficiency induced the nuclear translocation of Nrf2 via Keap1 degradation by autophagy and subsequently upregulated expression of HO-1. Another antioxidant enzyme, Cu/ZnSOD, which is a cytoplasmic SOD, was also higher in the ID group than in the CTL group (Fig. (C)). In contrast, MnSOD, which is known as a mitochondrial SOD, exhibited no significant change in expression level (Fig. (D)). In previous studies, oxidative stress was shown to be increased in iron deficiency through an increase in oxidant levels and a decrease in antioxidant enzyme capacity in the liver and erythrocytes.Citation8,16) However, it remains unclear how antioxidant enzymes act against oxidative stress in the heart. Thus, it appears that iron metabolism, ROS generation, and the antioxidant defense system in the heart are different from other tissues. In this study, expression of Cu/ZnSOD mRNA was induced in the heart by 4 weeks of dietary iron deficiency. Indeed, it was previously demonstrated that oxidative stress induces antioxidative enzymes, including SOD.Citation17) Taken together, these results suggest that the induction of antioxidant enzymes in the heart is a sign of defense against iron deficiency-induced oxidative stress.
Fig. 2. Effect of iron deficient on Nrf2 and its target genes in control and iron deficient mice in heart.
Notes: (A) Heart tissues of CTL and ID group were prepared nuclear fraction and subjected to Western blot against anti-Nrf2 antibody. Histone was used as nuclear marker. (B), (C), and (D), After total RNA of heart tissue was extracted using TRIzol reagent, cDNA was synthesized using 1 μg of total RNA and an RNA PCR AMV kit (Takara Bio, Shiga, Japan). Then, the expression levels of HO-1 (B), CuZnSOD (C), and MnSOD (D) mRNA were quantified by qRT-PCR, with β-actin serving as the internal standard. Each value represents the mean ± SEM.
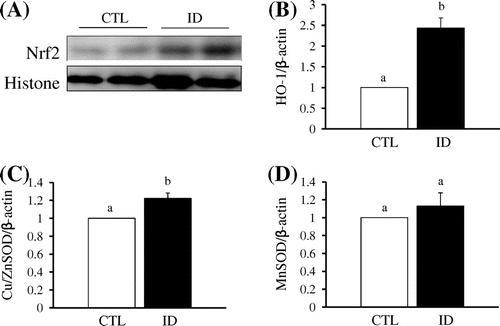
In conclusion, we investigated the effects of dietary iron deficiency on the redox system in the heart by measuring markers of redox-associated molecules. Dietary iron deficiency activated the Nrf2/Keap1 system and autophagy to help survival by removing damaged proteins in the heart. Finally, our results provide insight into the molecular mechanisms underlying the redox system in the heart induced by iron deficiency.
Additional information
Funding
References
- Aisen P, Enns C, Wessling-resnick M. Chemistry and biology of eukaryotic iron metabolism. Int. J. Biochem. Cell Biol. 2001;33:940–959.10.1016/S1357-2725(01)00063-2
- Salahudeen AA, Bruick RK. Maintaining Mammalian iron and oxygen homeostasis: sensors, regulation, and cross-talk. Ann. N. Y. Acad. Sci. 2009;1177:30–38.10.1111/j.1749-6632.2009.05038.x
- Shinoda S, Yoshizawa S, Nozaki E, Tadai K, Arita A. Marginally excessive iron loading transiently blocks mucosal iron uptake in iron-deficient rats. Am. Physiol. Gastrointest Liver Physiol. 2014;307:G89–G97.10.1152/ajpgi.00305.2013
- Harris MM, Houtkooper LB, Stanford VA, Parkhill C, Weber JL, Flint-Wagner H, Weiss L, Going SB, Lohman TG. Dietary iron is associated with bone mineral density in healthy postmenopausal women. J. Nutr. 2003;133:3598–3602.
- Gao X, Qian M, Campian JL, Marshall J, Zhou Z, Roberts AM, Kang YJ, Prabhu SD, Sun XF, Eaton JW. Mitochondrial dysfunction may explain the cardiomyopathy of chronic iron overload. Free Radic. Biol. Med. 2010;49:401–407.10.1016/j.freeradbiomed.2010.04.033
- Katsumata S, Katsumata-Tsuboi R, Uehara M, Suzuki K. Severe iron deficiency decreases both bone formation and bone resorption in rats. J. Nutri. 2009;139:238–243.
- Harvery LJ, Armah CN, Dainty JR, Foxall RJ, Lewis DJ, Langford NJ, Fairweather-Tait SJ. Impact of menstrual blood loss and diet on iron deficiency among women in the UK. Br. J. Nutr. 2005;94:557–564.10.1079/BJN20051493
- Uehara M, Chiba H, Mogi H, Suzuki K, Goto S. Induction of increased phosphatidylcholine hydroperoxide by an iron-deficient diet in rats. J. Nutr. Biochem. 1997;8:385–391.10.1016/S0955-2863(97)00045-4
- Rachmilovich-Calis S, Masarwa A, Meyerstein N, Meyerstein D, van Eldik R. New mechanistic aspects of the Fenton reaction. Chem. Eur. J. 2009;15:8303–8309.10.1002/chem.v15:33
- Inoue H, Maeda-Yamamoto M, Nesumi A, Tanaka T, Murakami A. Low and medium but not high doses of green tea polyphenols ameliorated dextran sodium sulfate-induced hepatotoxicity and nephrotoxicity. Biosci. Biotechnol. Biochem. 2013;77:1223–1228.10.1271/bbb.121003
- Beresewicz A, Horackova M. Alterations in electrical and contractile behavior of isolated cardiomyocytes by hydrogen peroxide: possible ionic mechanisms. J. Mol. Cell. Cardiol. 1991;23:899–918.10.1016/0022-2828(91)90133-7
- Azad MB, Chen Y, Gibson SB. Regulation of autophagy by reactive oxygen species (ROS): implications for cancer progression and treatment. Antioxid. Redox Signal. 2009;11:777–790.10.1089/ars.2008.2270
- Li J, Ichikawa T, Villacorta L, Janicki JS, Brower GL, Yamamoto M, Cui T. Nrf2 protects against maladaptive cardiac responses to hemodynamic stress. Arterioscler Thromb. Vasc. Biol. 2009;29:1843–1850.10.1161/ATVBAHA.109.189480
- Mercado N, Thimmulappa R, Thomas CM, Fenwick PS, Chana KK, Donnelly LE, Biswal S, Ito K, Barnes PJ. Decreased histone deacetylase 2 impairs Nrf2 activation by oxidative stress. Biochem. Biophys. Res. Commun. 2011;406:292–298.10.1016/j.bbrc.2011.02.035
- Taguchi K, Fujikawa N, Komatsu M, Ishii T, Unno M, Akaike T, Motohashi H, Yamamoto M. Keap1 degradation by autophagy for the maintenance of redox homeostasis. Proc. Natl. Acad. Sci. USA. 2012;109:13561–13566.10.1073/pnas.1121572109
- Madhikarmi NL, Murthy KR. Antioxidant enzymes and oxidative stress in the erythrocytes of iron deficiency anemic patients supplemented with vitamins. Iran Biomed. J. 2014;18:82–87.
- Noeman SA, Hamooda HE, Baalassh AA. Biochemical study of oxidative stress markers in the liver, kidney and heart of high fat diet induced obesity in rats. Diabetol. Metab. Syndr. 2011;3:17.10.1186/1758-5996-3-17