Abstract
We isolated a novel yellow pigment from a model Maillard reaction system containing l-cysteine, l-lysine, and glucose and identified it as 6-hydroxy-3[R],7a[S]-dimethyl-7-oxo-2,3-dihydropyrrolo[2,1-b]thiazole-3-calboxylic acid. This compound was a novel pyrrolothiazole derivative carrying a carboxy group and was named pyrrolothiazolate. This compound showed the absorption maxima at 300 and 360 nm under acidic and neutral conditions, while 320 and 400 nm did under alkaline conditions. Pyrrolothiazolate formed from cysteine and glucose was the major low-molecular-weight Maillard pigment in the reaction mixture, and its formation was stimulated by adding lysine to the reaction cocktail. After heating at 110 °C for 2 h, 1–2 mg/mL of pyrrolothiazolate was formed in a reaction mixture containing 100 mM cysteine, 200 mM lysine, and 300 mM glucose dissolved in 0.5 m acetate buffer (pH 6.0) or 0.5 m phosphate buffer (pH 6.5).
Graphical Abstract
A novel Maillard pigment, pyrrolothiazolate, was identified in the reaction mixture containing l-cysteine and d-glucose.
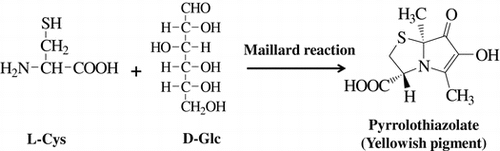
The Maillard reaction between reducing sugars and amino acids or proteins makes a critical influence on the quality of cooked or stored foods, because most foods contain sugars and amino acids or proteins, and this reaction occurs during the process of heating or storage. Browning is the most apparent result of the Maillard reaction. Various heated or long-stored foods show brown or dark color. One typical example of browning by the Maillard reaction is the color of soy sauce, which is a traditional and indispensable seasoning in Japanese cuisines and is made of wheat and soybean in the presence of salt and koji (fungus Aspergillus oryzae). Its color is formed during the production process involving heating, fermentation, and pasteurization. The color of soy sauce is mainly due to melanoidin, which is a brownish, hydrophilic, acidic, and nitrogen-containing compound formed by the Maillard reaction. But as melanoidin is heterogeneous and high-molecular-weight polymers, it is difficult to determine the chemical structure.Citation1) On the other hand, several low-molecular-weight colored compounds formed from amino acids have been reported. For example, Hayase et al.Citation2) and Shirahashi et al.Citation3) reported a blue and red Maillard pigments formed from a glycine-xylose model solution. Our group also reported some yellow Maillard pigments such as furpipateCitation4,5) and dilysyldipyrrolones.Citation6−8) Even if the contribution of each low-molecular-weight colored compound to total color is small, it is meaningful to identify low-molecular-weight colored compounds in foods and solutions of the Maillard reaction to understand and regulate the Maillard pigments, because various compounds are formed by the Maillard reaction and each compound is considered to cumulatively contribute to the total color. Accordingly, our group has examined a low-molecular-weight pigment in soy sauce. Recently, we isolated and identified 2,4-dihydroxy-2,5-dimethyl-3(2H)-thiophenone (DHDMT) from soy sauce as a low-molecular-weight yellow compound,Citation9,10) although its contribution to the total color of soy sauce was very low. DHDMT is formed by reaction between reducing hexose and cysteine.Citation11) There is no report on Maillard pigments containing sulfur except for DHDMT, while several Maillard pigments containing nitrogen are reported as mentioned above.
The aim of this study was to find a low-molecular-weight Maillard pigment formed from cysteine, a sulfur containing amino acid. Here, we describe isolation and identification of a novel Maillard yellowish pigment, named pyrrolothiazolate, as well as its formation conditions.
Materials and methods
Preparation of model solutions
Amino acids (10 mM l-cysteine, 30 mM l-lysine, or 10 mM l-cysteine plus 30 mM l-lysine) were dissolved in a solution containing 100 mM d-glucose and 500 mM acetate buffer (pH 5.0). Each solution was put into a test tube with a cap and heated for 3 h at 110 °C. Solutions containing various concentrations of amino acids (0–200 mM l-cysteine and 0–500 mM l-lysine) and glucose (0–1000 mM) and having different initial pH (pH 4.0–7.5, 500 mM acetate buffer and pH 6.0–8.0, 500 mM phosphate buffer) were also prepared and heated for 0–9 h at 110 °C.
HPLC analysis
Model solutions were analyzed with a reversed-phase HPLC system equipped with a photodiode-array detector under the following conditions: system, Agilent 1100 series (Palo Alto, CA, USA); column, TSK gel ODS-100 V (4.6 mm i.d. × 250 mm, Tosoh, Tokyo, Japan); eluent, solution A (0.1% AcOH-water, v/v) and solution B (MeOH), 0% B for 0–10 min and 0–70% B (v/v) for 10–40 min with a linear gradient; flow rate, 1.0 mL/min; column temp., 50 °C; detection, 220–500 nm. Pyrrolothiazolate was detected at a retention time of about 12 min under this condition. For quantification of pyrrolothiazolate, 2% AcOH/water (v/v) was used for eluent and 370 nm was used for detection. Pyrrolothiazolate was detected at a retention time of about 10 min in this condition.
Isolation of pyrrolothiazolate
A solution (1.2 L) containing 100 mM l-cysteine, 30 mM l-lysine, 500 mM d-glucose, and 500 mM acetate buffer (pH 5.0) was autoclaved at 120 °C for 3 h. After cooling, the heated solution was washed by ethyl acetate (1.2 L, 3 times). The pH of the solution was adjusted to less than 2 by adding 6 M HCl, before pyrrolothiazolate was extracted with ethyl acetate (1.2 L, 3 times). The ethyl acetate layer was concentrated in vacuo, before being applied to a column of silica gel (6 cm i. d. × 16 cm; Silica gel 60, Merck, Darmstadt, Germany), which was successively developed with dichloromethane/AcOH (20:1, v/v), ethyl acetate/AcOH (40:1, v/v), and MeOH. The fractions containing pyrrolothiazolate (dichloromethane: AcOH = 20:1) were combined, which was concentrated in vacuo and applied to a preparative HPLC under the following conditions: pump, L-6000 (Hitachi, Tokyo, Japan); column, YMC-pack R&D ODS-A (20 mm i.d. × 250 mm, YMC, Kyoto, Japan); eluent, 0.1% AcOH/water (v/v); flow rate, 10 mL/min; detector, L-4200 (Hitachi, Tokyo); and wavelength for detection, 280 nm. A peak at a retention time of about 47 min was collected. After the pH of the solution was adjusted to less than 2, pyrrolothiazolate was extracted with ethyl acetate. The ethyl acetate layer was concentrated in vacuo, before a pale yellow powder (ca. 76 mg) being obtained. The powder was dissolved in a little amount of MeOH and placed on refrigerator. A colorless prism crystal of pyrrolothiazolate was obtained.
Instrumental analyses
Spectroscopic measurements were done by using the following instruments: spectrophotometer (Multispec-1500, Shimadzu, Kyoto, Japan), polarimeter (P-1010, JASCO, Tokyo), NMR (Bruker Advance 600, Bruker Biospin, Karlsruhe, Germany), and MS (Triple TOF 4600, AB Sciex, Foster, CA, USA).
X-ray analysis
A colorless prism crystal of C9H11NO4S having approximate dimensions of 0.50 × 0.40 × 0.20 mm was mounted on a glass fiber. All measurements were made on a Rigaku R-AXIS RAPID diffractometer (Rigaku, Tokyo) using graphite-monochromated Cu-Kα radiation. Indexing was performed from three oscillations that were exposed for 40 s, the crystal-to-detector distance being 127.40 mm.
Physicochemical properties of pyrrolothiazolate
Pyrrolothiazolate was soluble in water and MeOH, slightly soluble in acetone and ethyl acetate, and insoluble in dichloromethane and diethyl ether. M.p.: 146 °C (dec). UV λmax nm (ε): 300 (4,400) and 360 (5,000) in 0.01 M HCl; 300 (3,500) and 360 (5,800) in water; 320 (2,600) and 400 (4,800) in 0.01 M NaOH. [α]25D = +187.4° (H2O, c = 0.2). MS (m/z): 230.0485 (M + H+), calcd. for C9H11NO4S, 230.0482. 1H and 13C NMR data are summarized in Table .
Table 1. NMR Data for pyrrolothiazolate.
Evaluation of the color intensity
The color intensity was estimated by the color dilution method,Citation12) aqueous solution of pyrrolothiazolate in 0.5 M acetate buffer (pH 6.0) being successively diluted. An aliquot (200 μL) was put into a 96-well microplate. The detection limits for pyrrolothiazolate in 0.5 M acetate buffer (pH 6.0) and a test solution were visually estimated by the triangle difference test, using eight panelists. A test solution was prepared by heating a solution containing 0.1 M cysteine, 0.2 M lysine, 0.3 M glucose, and 0.5 M acetate buffer (pH 6.0) at 110 °C for 2 h. The color activity (unit) of pyrrolothiazolate in a solution was calculated as [pyrrolothiazolate concentration (mg/mL)]/[its detection limit (mg/mL)]. The contribution of pyrrolothiazolate to total color (%) was calculated by [color activity of pyrrolothiazolate in a test solution]/[detection limit of the test solution (dilution ratio)] × 100.
Results and discussion
Analysis of cysteine–glucose model Maillard solutions
At first, we prepared three kinds of model Maillard solutions containing l-cysteine and glucose (A); l-lysine and glucose (B); and l-cysteine, l-lysine, and glucose (C) and examined them by a reversed-phase HPLC equipped with a diode-array detector to find a unique peak in the cysteine system showing a clear absorption maximum at or near the visible region. The concentrations of cysteine and lysine, and glucose in these model solutions were set at the levels of half cysteine and lysine in total amino acids and that of free glucose in Japanese soy sauce.Citation13) Fig. shows typical chromatograms of these reaction mixtures detected by an absorbance at 400 nm. Although several small peaks appeared on the chromatogram of the Maillard solutions containing cysteine and glucose, only two peaks (X and Y in Fig. (A)) at the retention times of about 12 and 23 min showed the specific absorption maxima at or near the visible region. As the latter peak was DHDMT, we focused on the former peak showing absorption maxima at 300 and 360 nm. This peak was enhanced by addition of lysine to the reaction mixture (Fig. (C)) but did not appear on the chromatogram of the reaction mixture of lysine and glucose (Fig. (B)). These results strongly suggest that this peak is the major low-molecular-weight Maillard pigment formed from cysteine. So, we decided to isolate and identify this compound, named pyrrolothiazolate as described later.
Fig. 1. Typical profiles of reversed-phase HPLC of model Maillard solutions of cysteine and glucose (A), lysine and glucose (B) and cysteine, lysine, and glucose (C).
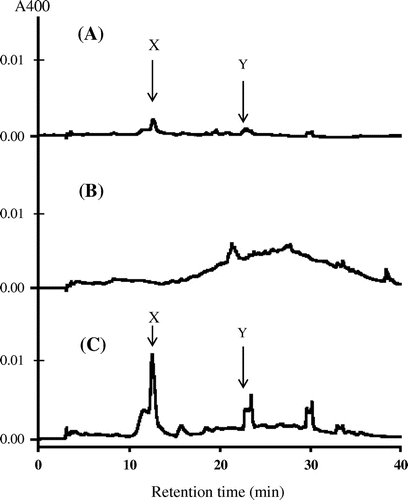
Isolation and identification of pyrrolothiazolate
In a preliminary experiment, we found that the pigment was transferred into an acidic substance fraction with ethyl acetate from a reaction mixture. Then, we purified it from an acidic substance fraction of a reaction mixture containing l-cysteine, l-lysine, and glucose using such chromatographic procedures as silica gel column and preparative HPLC. As a result, pale yellow powder showing a single peak on HPCL was obtained (Fig. ). Fig. shows the UV–vis spectra of pyrrolothiazolate. This compound showed two absorption maxima at 300 and 360 nm under neutral and acidic conditions, while at 320 and 400 nm did under alkaline conditions. Yellow color of the solution turned dense in alkaline region. This result suggests this compound has an enol structure formed by the Maillard reaction. The MS data showed its molecular weight, molecular formula, and degree of unsaturation to be 229, C9H11NO4S, and 5, respectively. The molecular formula suggests that pyrrolothiazolate was formed from 1 molecular of cysteine and 1 molecular of glucose by dehydration of 4 molecules of H2O. The NMR data are summarized in Table . The 1H NMR data show two singlet methyl peaks (δH 2.12 and 1.59 ppm) and three methine peaks (δH 4.66, 3.62, and 3.20 ppm). The methine peaks were coupled each other, which was shown by coupling constants and 1H–1H correlation spectroscopy (COSY). The 13C NMR data showed 9 kinds of carbons, among which the C–H bonds of four carbons were assigned by a heteronuclear single quantum correlation (HSQC) analysis. The HSQC data show that five carbons at δC 77.9, and 134.9 (an olefinic carbon), 161.7 (an olefinic carbon carrying a hydroxy group), 173.5 (a carboxy group), and 197.5 (a carbonyl group) were quaternary. Among residual carbons, the carbons at δC 11.7 and 24.6 were methyl groups and the carbon at δC 36.5 was bound to two protons at δH 3.62 and 3.20, indicating that these protons are methylene protons. These results suggest a partial structure of –S–CH2–CH(N–)–COOH derived from l-cysteine. The heteronuclear multiple bond correlation analysis (HMBC) showing that the protons at δH 3.20 and 4.66 were correlated with carbons at δC 173.5 supported this partial structure (Fig. (A)). Other six carbons containing two methyl groups were considered to be derived from glucose. The HMBC analysis showed a partial structure of CH3–C–C(=O)–C=C–CH3 of the six carbons as well as the connection between this six carbons and another partial structure derived from cysteine (Fig. (A)). As we could obtain a crystal of pyrrolothiazolate from cold methanol, the crystal was applied to X-ray analysis. X-ray crystal data were as follows: C9H11NO4S, Mr = 229.25, triclinic, space group P1 (#1), μ(Cu-Kα) = 28.765 cm−1, T = 123 K, a = 7.4558(2) Å, b = 7.8544(2) Å, c = 9.7079(2) Å, α = 90.107(2)°, β = 106.239(2)°, γ = 112.839(2)°, V = 499.10(2) Å3, Z = 2, Dc = 0.763 g cm−3, and F(000) = 240. A total of 5345 reflections were collected, with 2970 being unique (Rint = 0.0626). R1, R, and wR2 were, respectively, 0.0502[I > 2σ(I)], 0.0585 (all data), and 0.1721 (all data). Further details of the crystal structure investigation are deposited in the Cambridge Crystallographic Data Center as supplementary publication no. CCDC1040569. Copies of the data can be obtained free of charge by an application to CCDC at 12 Union Road, Cambridge CB2 1EZ, UK [Fax: + 44 1223 336 033, E-mail: [email protected]]. The X-ray analysis of pyrrolothiazolate showed its chemical structure to be 6-hydroxy-3[R],7a[S]-dimethyl-7-oxo-2,3-dihydropyrrolo[2,1-b]thiazole-3-calboxylic acid (Fig. (B)). This structure is consistent with all the instrumental data. This compound was optically active, and its specific rotation [α]D was +189° in water. As this structure showed no replacement reaction on the chiral carbon of l-cysteine occurred and that the stereo-chemical configuration of l-cysteine was kept in pyrrolothiazolate, the absolute configuration was thus determined. This compound was a novel pyrrolothiazole derivative having a carboxy group and was named pyrrolothiazolate. There is no report on pyrrolothiazole skeleton as a pigment and a Maillard reaction product, while several pyrrolothiazole derivatives are synthesized.Citation14−16) Haleva-Toledo et al. suggested an unidentified yellow pigment in the heated solution containing cysteine and glucose.Citation17) Pyrrolothiazolate may have been formed in the solution.
Fig. 2. Typical profiles of reversed-phase HPLC of a reaction mixture before isolation (A) and an isolated peak (B).
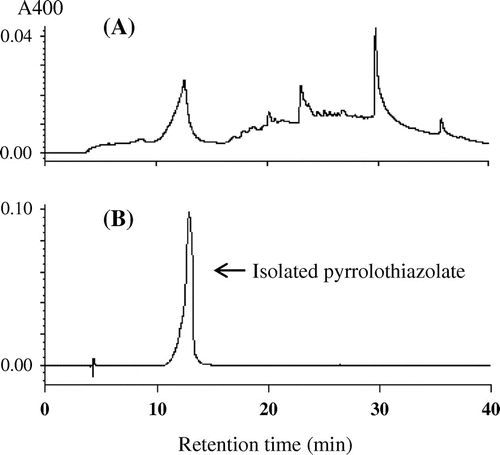
Formation of pyrrolothiazolate in model systems
Conditions for pyrrolothiazolate formation were then examined in model systems. Although pyrrolothiazolate was formed from cysteine and glucose, in a preliminary experiment, we found that addition of lysine to a reaction mixture containing cysteine and glucose enhanced its formation. So, lysine was added to a model system. Fig. (A) shows the effect of the cysteine on the formation of pyrrolothiazolate. The concentration of cysteine influenced the production of pyrrolothiazolate, and about 25–100 mM of cysteine was optimal for its formation in the presence of 100 mM glucose and 40 mM lysine. The concentrations of glucose and lysine also influenced the production of pyrrolothiazolate. Fig. (B) shows that about 200–500 mM of glucose was optimal in the presence of 100 mM cysteine and 40 mM lysine. Further, Fig. (C) shows that about 100–300 mM was optimal in the presence of 100 mM cysteine and 300 mM glucose for the formation of pyrrolothiazolate.
Fig. 5. Effects of concentrations of cysteine (A) glucose (B) and lysine (C) on the formation of pyrrolothiazolate.
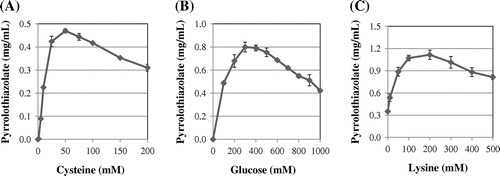
Fig. (A) shows the effect of initial pH and buffer types on the formation. The optimal initial pH was around 6.0 in the acetate buffer and was around 6.5 in the phosphate buffer. More pyrrolothiazolate was formed in the phosphate buffer than in the acetate buffer at the same pH. In the phosphate buffer, about 2.5 mg/mL of pyrrolothiazolate was formed. But judging from the shape of chromatograms, more melanoidin was formed in the phosphate buffer than in the acetate buffer (data not shown). Fig. (B) shows the effect of reaction time on the pyrrolothiazolate formation in the acetate buffer. The concentration of pyrrolothiazolate reached the maximum (about 1.5 mg/mL) at 2 h of incubation and then gradually decreased. Polymerization or degradation seems to occur for a longer incubation.
Fig. 6 Effect of initial pH and buffer types (A) and heating time (B) on the formation of pyrrolothiazolate.
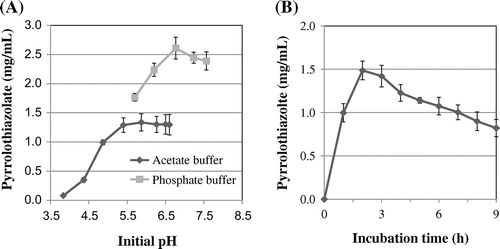
Color contribution of pyrrolothiazolate
As mentioned above, pyrrolothiazolate was the major peak showing the absorption maximum at or near the visible region on a chromatogram of reversed-phase HPLC. The detection limit for pyrrolothiazolate visually estimated was about 0.6 mg/mL. The test solution prepared from cysteine, lysine, and glucose contained 1.5 mg/mL of pyrrolothiazolate, and the detection limit for this solution was 325-fold dilution. These results show that pyrrolothiazolate contributed to about 0.8% of the total color of the solution. As the contribution of DHDMT to the total color of soy sauce was about 0.02%,Citation9) this value seems to be high as a low-molecular-weight pigment containing sulfur. Considering that the color of Maillard reaction is composed of a wide range of chromophores including melanoidins and that each chromophore contributes to the total color cumulatively, this partial contribution would be meaningful.
In conclusion, a novel pyrrolothiazole derivative, pyrrolothiazolate, was isolated and identified, which was the major low-molecular-weight Maillard pigment in a model system containing cysteine and glucose.
Additional information
Funding
References
- Wang H-Y, Qian H, Yao W-R. Melanoidins produced by the Maillard reaction: structure and biological activity. Food Chem. 2011;128:573–584.10.1016/j.foodchem.2011.03.075
- Hayase F, Takahashi Y, Tominaga S, Miura M, Gomyo T, Kato H. Identification of blue pigment formed in a D-xylose-glycine reaction system. Biosci. Biotechnol., Biochem. 1999;63:1512–1514.10.1271/bbb.63.1512
- Shirahashi Y, Watanabe H, Hayase F. Identification of red pigments formed in a D-xylose-glycine reaction system. Biosci. Biotechnol. Biochem. 2009;73:2287–2292.10.1271/bbb.90382
- Murata M, Totsuka H, Ono H. Browning of furfural and amino acids, and a novel yellow compound, furpipate, formed from lysine and furfural. Biosci. Biotechnol., Biochem. 2007;71:1717–1723.10.1271/bbb.70129
- Totsuka H, Tokuzen K, Ono H, Murata M. A novel yellow compound and furpipate derivatives formed from furfural or 5-hydroxymethylfurfural in the presence of lysine. Food Sci. Technol. Res. 2009;15:45–50.10.3136/fstr.15.45
- Sakamoto J, Takenaka M, Ono H, Murata M. Novel yellow compounds, dilysyldipyrrolones A and B, formed from xylose and lysine by the Maillard reaction. Biosci. Biotechnol. Biochem. 2009;73:2065–2069.10.1271/bbb.90257
- Nomi Y, Sakamoto J, Takenaka M, Ono H, Murata M. Conditions for the formation of dilysyl-dipyrrolones A and B, and novel yellow dipyrrolone derivatives formed from xylose and amino acids in the presence of lysine. Biosci. Biotechnol. Biochem. 2011;75:221–226.10.1271/bbb.100390
- Nomi Y, Masuzaki R, Terasawa N, Takenaka M, Ono H, Otsuka Y, Murata M. Formation mechanism and characterization of dilysyldipyrrolones, the Maillard-type yellow pigments. Food Funct. 2013;4:1067–1075.10.1039/c3fo30366g
- Sato M, Nomi Y, Yamada S, Takenaka M, Ono H, Murata M. Identification of 2,4-dihydroxy-2,5-dimethyl-3(2H)-thiophenone as a low molecular weight yellow pigment in soy sauce. Biosci. Biotechnol., Biochem. 2011;75:1240–1244.10.1271/bbb.100867
- Furusawa R, Goto C, Sato M, Nomi Y, Murata M. Formation and distribution of 2,4-dihydroxy-2,5-dimethyl-3(2H)-thiophenone, a pigment, an aroma, and a biological active compound formed by the Maillard reaction, in foods and beverages. Food Funct. 2013;4:1076–1081.10.1039/c3fo30367e
- Shu C-K, Hagedorn ML, Mookherjee BD, Ho C-T. Two novel 2-hydroxy-3(2H)-thiophenones from the reaction between cystine and 2,5-dimethyl-4-hydroxy-3(2H)-furanone. J. Agric. Food. Chem. 1985;33:638–641.10.1021/jf00064a017
- Hofmann T. Characterization of the most intense colored compounds from Maillard reactions of pentoses by application of color dilution analysis. Carbohydr. Res. 1998;313:203–213.10.1016/S0008-6215(98)00279-1
- Standard table of food composition in Japan; [cited 2014 Dec 25] Available from: http://fooddb.mext.go.jp/.
- Donald B, Elzey TK, Hatfield LD, Kinnick MD, Marin Jr JM. Ganma-lactam analogues of the carbapenems. Tetrahedron Lett. 1986;27:3453–3456.
- Lalezari I, Schwartz EL. Synthesis and antineoplastic activity of 5-aryl-2,3-dihydropyrrolo[2,1-b]thiazole-6,7-dimethanol 6,7-bis(isopropylcarbamates). J. Med. Chem. 1988;31:1427–1429.10.1021/jm00402a030
- Karthikeyan SV, Bala BD, Raja VPA, Perumal S, Yogeeswari P, Sriram D. A highly atom economic, chemo-, regio- and stereoselective synthesis and evaluation of spiro-pyrrolothiazoles as antitubercular agents. Bioorg. Med. Chem. Lett. 2010;20:350–353.10.1016/j.bmcl.2009.10.107
- Haleva-Toledo E, Naim M, Zehavi U, Rouseff RL. Effects of L-cysteine and N-acetyl-l-cysteine on 4-hydroxy-2,5-dimethyl-3(2H)-furanone (furaneol), 5-(hydroxymethyl)furfural, and 5-methylfurfural formation and browning in buffer solutions containing either rhamnose or glucose and arginine. J. Agric. Food. Chem. 1999;47:4140–4145.10.1021/jf9813788