Abstract
Nano-TiO2 has been reported to be an efficient photocatalyst, which is able to produce reactive oxygen species (ROS) under UVA irradiation. In this study, we investigated the effects of nano-TiO2 on the cytotoxicity, induction of apoptosis, and the putative pathways of its actions in HaCaT cells. We show that nano-TiO2 is a potent inducer of apoptosis and that it transduces the apoptotic signal via ROS generation, thereby inducing mitochondrial permeability transition (MPT) and activating Caspase-3 from HaCaT cells. ROS production, mitochondrial alteration, and subsequent apoptotic cell death in nano-TiO2-treated cells were blocked by the MPT pore-blocker cyclosporin A. Taken together, our data indicate that nano-TiO2 induces the ROS-mediated MPT and resultant Caspase-3 activation.
Graphical abstract
Nano-TiO2 led to a significant decrease of cell viability mediated mitochondrial permeability transition (MPT) pore.
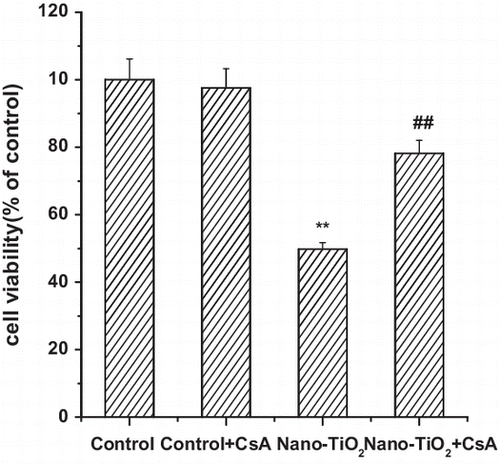
Nano-titanium dioxide (nano-TiO2) has been used in a range of products, including plastic, paper, welding rod-coating material, sunscreen, and food colorant. However, nano-TiO2 is known to be a photobiologically active agent,Citation1) the increasing use of nano-TiO2 has raised public concern about the potential risks to human health. When exposed to UV irradiation, nano-TiO2 was considered to drive the generation of reactive oxygen species (ROS), such as superoxide anion radicals, hydrogen peroxide, free hydroxyl radicals, and singlet oxygen.Citation2) ROS generated following UV irradiation of nano-TiO2 are genotoxic in Chinese hamster and mouse lymphoma cell lines,Citation3) and are cytotoxic to cultured HeLa cells,Citation4) human fibroblasts,Citation5) Chinese hamster ovary cells,Citation6) and human colon carcinoma Ls-174-t cells.Citation7) It was recently demonstrated that the exposure of several cell lineages to nano-TiO2 causes decreased function of the mitochondria,Citation8) which are intracellular organelles and major source of ROS formation, it is possible that this organelle could participate in the oxidative burst.Citation9−11) However, the mechanism involving mitochondrial pathways in nano-TiO2-induced cell death remains unknown. Ca2+overload, oxidative stress, may lead to mitochondrial permeability transition (MPT) pore opening.Citation12) The MPT pore opening is characterized by depolarization of mitochondrial membrane potential (MMP), mitochondrial swelling, and rupture of mitochondrial membrane. These processes lead to the release of cytochrome c and to other factors that play a critical role in apoptotic cell death.Citation13,14)
Thus, based on the hypothesis that exposure of cells to nano-TiO2 may impair mitochondria, the present study was undertaken to identify the contribution of MPT pore to nano-TiO2-induced cell death using the human keratinocyte (HaCaT) cell line, and to investigate the possible mechanisms involved. In this study, we focused on nano-TiO2-induced MPT and its role on HaCaT cell death.
Materials and methods
Nano-TiO2 and reagents
Nano-TiO2 (P25, 25% rutile and 75% anatase) with 21 nm was purchased from Degussa GmbH (Germany). The particle sizes and distribution of nano-TiO2 were measured by Tecnai G2 20 transmission electron microscope (FEI, Holland). Crystal structure was characterized by X’Pert Prox X-ray diffraction instrument (FEI, Holland). HaCaT cells were purchased from China Center for Type Culture Collection (CCTCC, China), Rhodamine 123, 2′,7′-dichlorofluorescin diacetate (DCFH-DA) were purchased from Sigma (USA). All other chemicals were of the highest grade that could be obtained commercially.
Preparation suspension of nano-TiO2
Nano-TiO2 was suspended in the cell culture medium and dispersed using a sonifier cell disruptor equipped with a microprobe for 10–15 min to produce suspension. In each study, the suspension was freshly prepared, and then immediately applied to HaCaT cells.
Cell culture and exposure to nano-TiO2
HaCaT cells were grown in modified Eagle’s medium (MEM) supplemented with 10% FBS in the presence of 5% CO2 in air at 37 °C. Cells were incubated up to about 24 h and grown to about 80% confluence before experiments. Cyclosporin A (CsA) was dissolved in phosphate-buffered saline (PBS) (10.0 μM) and pretreated for 30 min. After treatment, the inhibitor was removed and the cells were washed with PBS and replaced with culture medium, some experiments included treatment with nano-TiO2. All cells were irradiated with the UVA light for 1 h and then cultured for 24 h.
UVA irradiation
UVA light was provided by a UV lamp (Shanghai Jinguang Instrument, China) with continuous emission spectrum with a peak at 365 nm. The UV intensity at 365 nm was determined using a UVA radiometer (Beijing Normal University, China). The UVA lamp had a fluence rate of 3.5 mW/cm2 and is an appropriate substitute for UVA occurring in natural sunlight.
Measurement of intracellular ROS
Intracellular ROS were detected by fluorescent spectrophotometry (Hitachi F4500) using 2′,7′-dichlorofluorescin diacetate (DCFH-DA). After treatment, the cells were washed again with PBS, and then were homogenized in 300 μL 0.1% Triton X-100 (PBS, pH 7.4) through sonication on ice for 10 s. After incubation at 4 °C for 10 min, the homogenates were centrifuged at 4000 × g for 30 min, and the supernatants were used for assay with excitation wavelength at 488 nm and emission wavelength at 525 nm.
Cell viability assay
The cytotoxicity of particles was determined using the MTT assay as described.Citation15)
Evaluation of MMP by flow cytometry
MMP of the cells was monitored using a flow cytometer and the fluorescent dye Rhodamine 123 (Sigma, USA). Briefly, after treatment, cells were washed twice in cold PBS and then treated with trypsin–EDTA for 5 min to yield single cells. After centrifugation at 330 × g for 5 min, the pellets were resuspended in 400 μL PBS and incubated with 100 μL Rhodamine 123 (10 mg/mL) at 37 °C for 30 min. Then, the cells were washed twice with PBS and resuspended in 400 L PBS. The samples were analyzed for fluorescence using a flow cytometer.
Measurement of cellular ATP content
Intracellular ATP levels in the presence or absence of CsA (10 μM) were measured with an ATP bioluminescent luciferin/luciferase assay kit in combination with a luminometer plate reader (Veritas Microplate Luminameter, Beijing, China) according to the manufacturer’s instructions.
Flow cytometric analysis of cell apoptosis
Apoptotic cells were quantified using an Annexin V-FITC apoptosis detection kit (A9210, Sigma-Aldrich, USA). After treatment, cells were washed twice in cold PBS, and then treated with trypsin–EDTA for 5 min. Cells were centrifuged at 330 × g for 5 min and the pellets were resuspended in binding buffer at a density of 1 × 106 cells/mL. 100 μL of the solution was transferred to a culture tube and double-stained with 5 μL of Annexin V-FITC and 10 μL of Propidium Iodide(PI) (Sigma, USA), and the cells were incubated at room temperature for 15 min in the dark. Cell suspensions were readjusted to a final volume of 500 μL with 1 × Annexin V binding buffer for further analysis by flow cytometry (FACScan, Becton Dickinson LSR, USA).
Caspase-3 activity assay
Caspase-3 activity was measured as described.Citation12) Briefly, Caspase-3 assay kit (BD Biosciences Pharmingen, San Diego, CA, USA) was used and fluorescence was measured in the fluorimeter (F4500, Hitachi, Japan) with excitation at 380 nm and emission at 460 nm.
RT-PCR
Total RNA was prepared using Trizol reagent (Invitrogen, Carlsbad, CA) following manufacturer’s instruction. The OD260/OD280 ratio was detected to determine the purity of the RNA samples. Reverse transcription-polymerase chain reaction (RT-PCR) was conducted using a two-step RT-PCR kit (Fermentas, Canada) according to the manufacturer’s instruction. Amplification conditions were as follows: at 94 °C for 2 min, followed by 30 cycles at 94 °C for 30 s, 60 °C for 30 s, and 72 °C for 30 s, and then 72 °C for 5 min. One microgram of total RNA was used in each amplification. Primer designed for Keratine 6 (K 6) and GAPDH were synthesized by Shanghai Sangon Co. Ltd (Shanghai, China), as follows: K6 sense: 5′-acatcatgatgtaatcaccac-3′ and K6 antisense: 5′-atacaggctttgtacatcata-3′ (the product size is 270 bp); GAPDH sense: 5′-aatgaccccttcattgac-3′ and GAPDH antisense: 5′-tccacgacgtactcagcgc-3′ (the product size is 200 bp). The resulting PCR product was separated on a 2% agarose gel and visualized with ethidium bromide under ultraviolet light. Gel images were captured with GDS81000 Image System (UVP, USA) and quantified with Quantity System (Image J, USA). The level of GAPDH was used to normalize the results.
Statistical analysis
Statistical analyses were performed using Origin software (version 6.1, OriginLab, USA). Experimental results were expressed as mean ± standard deviation (SD) for three different replicates at each test concentration. Statistical significance was established at p < 0.01.
Results
Involvement of MPT pore in nano-TiO2-induced ROS formation and cell viability
First, we study the effect of UVA irradiation time on the viability of HaCaT cells. Time-response experiments were performed using UVA source. Cells treated under UVA irradiation for 1 h showed no significant loss of cell viability as that in the control cells. However, when the cells were treated under UVA irradiation for more than 1 h, cell viability decreased (Fig. (A)). So, for the next experiments, UVA irradiation time was chosen as 1 h. Pretreatment with 10.0 μM CsA did not significantly affect cell viability. Pretreatment of cells with 10.0 μM CsA for 30 min, then with nano-TiO2 for 24 h led to a significant increase in cell viability from 49.7 to 78.2% compared to cells treated with nano-TiO2 alone (Fig. (B)). Mitochondria are recognized as important players in the mechanisms of cytotoxicity due to their essential functions in the cell,Citation16) providing the major energy, generating ROS, regulating the intracellular Ca2+ homeostasis, and cell death mechanisms.Citation13,14) To investigate the upstream regulatory mechanisms leading to nano-TiO2-induced mitochondrial dysfunction, we examined the effect of nano-TiO2 on intracellular ROS levels by fluorescent spectrophotometry. As shown in Fig. (C), exposure of HaCaT cells to 200 μg/mL nano-TiO2 for 24 h led to a 1.8-fold accumulation of intracellular ROS compared with that of control group. Next, we explored if the mitochondrial membrane dysfunction is mediated by MPT pore. We treated HaCaT cells with 10.0 μM CsA, a MPT pore inhibitor, and found it blocks increased intracellular ROS level from 375 ± 7.8 to 250 ± 16.5 (Fig. (C)), indicating 33.3% inhibition of ROS production, while in the control group, it is not elevated and CsA alone has no effect on the ROS level in the cells (Fig. (C)).
Fig. 1. Effects of MPT pore in nano-TiO2-induced cell viability and ROS formation of HaCaT cells.
Notes: Effects of MPT pore in nano-TiO2 induced-(B) cell viability and (C) ROS formation of HaCaT cells. Cells were treated with 200 μg/mL nano-TiO2 only, or pretreated with CsA (10.0 μM) for 30 min, followed by treatment with 200 μg/mL nano-TiO2. Control was received culture medium only. All samples were irradiated with the UVA light for 1 h and then cultured for 24 h. Results are expressed as mean ± SEM of at least four different experiments (**p < 0.01 represents the comparison with the control group; ##p < 0.01 represents the comparison with the nano-TiO2 group).
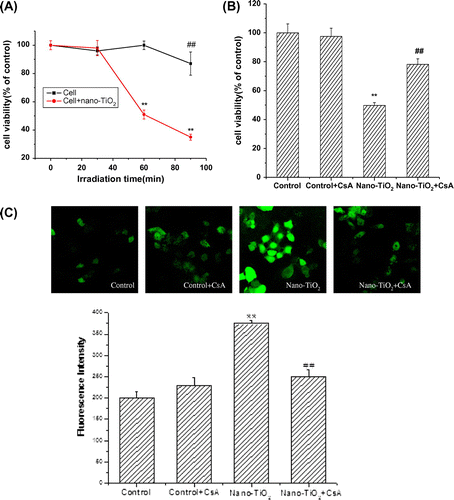
Involvement of MPT pore in nano-TiO2-induced mitochondrial dysfunction
Theoretically, mitochondrial dysfunction should occur as a result of ROS generation in cells. In our previous study, we have observed that HaCaT cells treated with nano-TiO2 (200 μg/mL) for 24 h showed a significant decrease in MMP.Citation8) In many pathophysiological models, but not all, MMP also involves the MPT pore, which mediates a non-selective permeabilization of the inner membrane (IM) and outer membrane (OM) to molecules of molecular mass under 1.5 kDa.Citation17,18) Here, to test the role of MPT pore in the ROS generation induced by nano-TiO2, we took advantage of the demonstrated inhibition of MPT pore by CsA. A significant decrease in MMP occurred when the cells were treated with 200 μg/mL nano-TiO2 compared to the control group (30.6%). Pretreatment with CsA reversed the reduction of MMP induced by nano-TiO2 (Fig. (A)). The loss of MMP may only occur when supplies of ATP are depleted.Citation12) In this study, the production of ATP was decreased upon nano-TiO2 treatment (Fig. (B)) and restored by CsA. These data imply the importance of MPT pore in nano-TiO2-induced mitochondrial dysfunction.
Fig. 2. Effects of MPT pore on MMP decrease and ATP depletion of HaCaT cells.
Notes: Effects of MPT pore on (A) MMP decrease and (B) ATP depletion of HaCaT cells. Cells were treated with 200 μg/mL nano-TiO2 only, or pretreated with CSA (10 μM) for 30 min, followed by treatment with 200 μg/mL nano-TiO2. Control was received culture medium only. All samples were irradiated with the UVA light for 1 h and then cultured for 24 h. Results are expressed as mean ± SEM. of at least four different experiments (**p < 0.01 represents the comparison with the control group; ##p < 0.01 represents the comparison with the nano-TiO2 group).
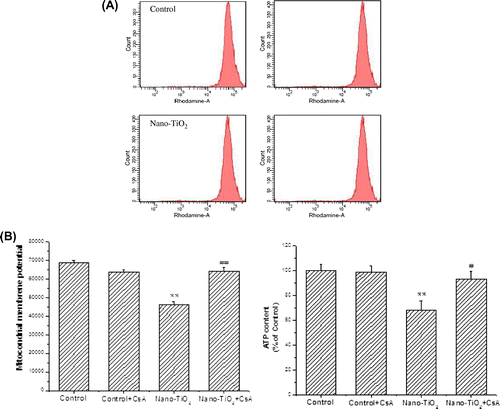
Involvement of MPT pore in nano-TiO2-induced-Caspase-3 activation and cell apoptosis
Caspase-3 is a protease known to be involved in apoptotic cell death. To address whether nano-TiO2 induce apoptosis, we measured Caspase-3 activity in HaCaT cells in the presence of nano-TiO2 (Fig. (A)). The Caspase-3 activity of cells treated with nano-TiO2 for 24 h was two times higher than that of control cells cultured without nano-TiO2. Meanwhile, all of these effects could be blocked by adding CsA to the culture medium. To further confirm whether CsA was capable of inhibiting nano-TiO2-induced apoptosis, the effect of CsA was analyzed by flow cytometry. After 24 h, the apoptotic cells increased from 6.3% (control) to 17.2% at 200 μg/mL nano-TiO2. On the other hand, pretreatment with CsA resulted in protection against nano-TiO2-induced cell apoptosis, with the apoptotic rate to 8.6% (Fig. (B)).
Fig. 3. Effects of MPT pore in nano-TiO2-induced Caspase-3 activation and cell apoptosis of HaCaT cells.
Notes: Effects of MPT pore in nano-TiO2-induced (A) Caspase-3 activation and (B) cell apoptosis of HaCaT cells. Cells were treated with 200 μg/mL nano-TiO2 only, or pretreated with CsA (10.0 μM) for 30 min, followed by treatment with 200 μg/mL nano-TiO2. Control was received culture medium only. All samples were irradiated with the UVA light for 1 h and then cultured for 24 h. Results are expressed as mean ± SEM of at least four different experiments (**p < 0.01 represents the comparison with the control group; ##p < 0.01 represents the comparison with the nano-TiO2 group).
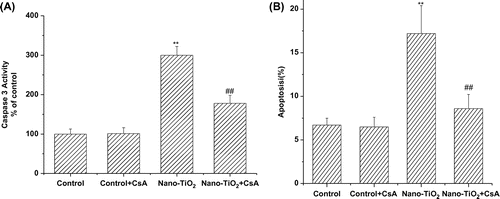
Involvement of MPT pore in nano-TiO2-induced Keratin 6 mRNA expression
Keratin 6 (K6) is induced in several skin diseases, including abnormal differentiation or hyperproliferative stratified epithelium such as in psoriasis. In order to assess whether nano-TiO2 induced decrease in K6 mRNA expression and the effect of MPT pore, cells were pretreated with the inhibitor CsA. K6 mRNA expression was significantly decreased when the cells were treated with 200 μg/mL nano-TiO2 for 24 h. Pretreatment with 10 μM CsA blocked the decrease in keratin mRNA expression induced by nano-TiO2 (Fig. (A) and (B)).
Fig. 4. Effects of MPT pore in nano-TiO2-induced K6 mRNA expression.
Notes: Effects of MPT pore in nano-TiO2-induced K6 mRNA expression. Cells were treated with 200 μg/mL nano-TiO2 only, or pretreated with CsA (10.0 μM) for 30 min, followed by treatment with 200 μg/mL nano-TiO2. Control was received culture medium only. All samples were irradiated with the UVA light for 1 h and then cultured for 24 h. Results are expressed as mean ± SEM of at least four different experiments (*p < 0.05 represents the comparison with the control group; #p < 0.05 represents the comparison with the nano-TiO2 group).
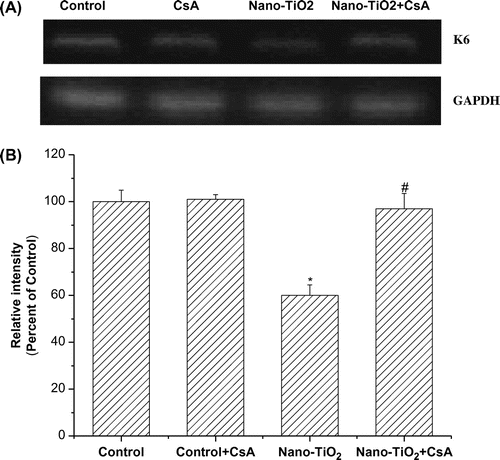
Discussion
Several lines of evidences suggest that toxicity by nano-TiO2 is due to the formation of ROS and RNS and damage of biological targets through oxidative stress.Citation1,19) The MMP is a vital component of respiring mitochondria and is linked to many mitochondrial functions.Citation20) Nano-TiO2-induced oxidative damage of HaCaT cells is likely through a complex mechanism involving ROS-related decrease in MMP.Citation18) This maybe because the properties of small size and large surface area render high reactivity in nanoparticles to generate ROS.Citation18) It was found that nanoscale TiO2 (P25) particles could stimulate brain microglia to produce ROS through the oxidative burst and interfere with mitochondrial energy production in vitro. The mitochondrial membrane dysfunction further results in the release of mitochondrial proteins and eventually activates Caspase-3 and PARP, leading to cell death. The resulting mitochondrial membrane dysfunction also induces the ROS production, leading to further mitochondrial damage due to ROS.Citation21) It appears that the MMP collapse is caused by the MPT pore opening and mitochondrial swelling.Citation22) Opening of the MPT pores, in turn, leads to release of proapoptotic proteins that play a determinant role in both necrosis and apoptosis mechanisms.Citation23) The observed MMP decrease following nano-TiO2 treatment in the present study could have resulted in changes in the physicochemical properties of mitochondrial membranes or rupture of the mitochondrial OM with subsequent release of cytochrome c and other proapoptotic factors from mitochondria that promote Caspase activation and apoptotic cell death.Citation24) Moreover, pretreatment with CsA attenuated both the rapid increase in apotosis and membrane potential collapse following nano-TiO2 administration. Therefore, it is possible to postulate that one or all of these events may be responsible for the toxicity and apoptosis treated with nano-TiO2. Our result is in agreement with other reports suggesting that nano-TiO2-induced ROS production not only leads to lipid peroxidation, but also to GSH oxidation, which could damage mitochondrial membrane integrity and open the MPT pores.Citation25) Furthermore, mitochondrial IM has high protein content and these proteins are one of the primary targets of mitochondrial-generated ROS. In fact, ROS could promote the oxidation of membrane protein thiol groups and thiol cross-linkage that finally could induce MPT and MPT pore opening. MPT pore opening causes unlimited proton movement across the inner mitochondrial membrane which results in mitochondrial swelling, MMP disruption, and uncoupling of oxidative phosphorylation. It could be suggested that release of cytochrome c and activation of Caspase 3 is due to mitochondrial swelling as a result of increased mitochondrial matrix volume, which might lead to the subsequent rupture of the OM or permeability of the outer mitochondrial membrane which leads to leakage of proapoptotic proteins.
Keratins are the major structural proteins of vertebrate epidermis and play critical roles in shaping the functions of the skin.Citation26) Some reports strongly suggest that aberrant expression of K6 disrupts keratin intermediate filament (KIF) ultrastructure and subsequently affects the mechanical resilience of keratinocytes. The expression of K6 may correspond to a modification of the terminal programme of differentiation after chronic UVB exposures.Citation27) In this study, nano-TiO2 decreases K6 mRNA expression in HaCaT cells, to determine if this is downstream of MPT pore opening, we pretreated HaCaT cells with CsA. Our results reveal that pretreatment with CsA mitigates the suppression of nano-TiO2 on K6 mRNA expression, thus broadening the spectrum of known mechanisms by which nano-TiO2 can impair HaCaT cells function.
In summary, we studied the effects of MPT pore on the generation of ROS and cytotoxicity in HaCaT cells to extend our insights to the mechanisms of nano-TiO2 toxicity. The experimental results suggest that nano-TiO2 induced mitochondrial oxidative stress and subsequently caused a gradual opening of MPT pore leading to collapse of MMP, Caspase-3 activation, which start cell death signaling and contribute to nano-TiO2-induced toxicity. Secondly, nano-TiO2 could induce decrease in K6 mRNA, since K6 is the initial step in shaping the functions of the skin. The present study suggests that MPT pore plays an important role in nano-TiO2 toxicity in HaCaT cells.
Disclosure statement
No potential conflict of interest was reported by the authors.
Additional information
Funding
References
- Gurr JR, Wang AS, Chen CH, Jan KY. Ultrafine titanium dioxide particles in the absence of photoactivation can induce oxidative damage to human bronchial epithelial cells. Toxicol. 2005;213:66–73.10.1016/j.tox.2005.05.007
- Hirakawa K, Mori M, Yoshida M, Oikawa S, Kawanishi S. Photo-irradiated titanium dioxide catalyzes site specific DNA damage via generation of hydrogen peroxide. Free Radical Res. 2004;38:439–447.10.1080/1071576042000206487
- Nakagawa Y, Wakuri S, Sakamoto K, Tanaka N. The photogenotoxicity of titanium dioxide particles. Mutat. Res. 1997;394:125–132.10.1016/S1383-5718(97)00126-5
- Cai R, Kubota Y, Shuin T, Sakai H, Hashimoto K, Fujishima A. Induction of cytotoxicity by photoexcited TiO2 particles. Cancer Res. 1992;52:2346–2348.
- Wamer WG, Yin JJ, Wei RR. Oxidative damage to nucleic acids photosensitized by titanium dioxide. Free Radical Biol. Med. 1997;23:851–858.10.1016/S0891-5849(97)00068-3
- Chino TU, Okunaga HT, Andoa M, Utsumi H. Quantitative determination of OH radical generation and its cytotoxicity induced by TiO2–UVA treatment, Toxicol. in Vitro. 2002;16:629–635.
- Zhang AP, Sun YP. Photocatalytic killing effect of TiO2 nanoparticles on Ls-174-t human colon carcinoma cells. World J. Gastroenterol. 2004;10:3191–3193.
- Xue CB, Wu JH, Liu W, Lan FL, Yang XL, Zeng FD, Xu HB. Nano titanium dioxide induces the generation of ROS and potential damage in HaCaT cells under UVA irradiation. J. Nanosci. Nanotechnol. 2010;10:8500–8507.10.1166/jnn.2010.2682
- Braydich-Stolle L, Hussain S, Schlager JJ, Hofmann MC. In vitro cytotoxicity of nanoparticles in mammalian germline stem cells. Toxicol. Sci. 2005;88:412–419.10.1093/toxsci/kfi256
- Hussain MS, Javorina AK, Schrand AM, Duhart HM, Ali SF, Schlager JJ. The interaction of manganese nanoparticles with PC-12 cells induces dopamine depletion. Toxicol. Sci. 2006;92:456–463.10.1093/toxsci/kfl020
- Calabrese V, Scapagnini G, Giuffrida-Stella AM, Bates TE, Clark JB. Mitochondrial involvement in brain function and dysfunction: relevance to aging, neurodegenerative disorders and longevity. Neurochem. Res. 2001;26:739–764.10.1023/A:1010955807739
- Qiong D, Xiao LB, Xu XL, Zhu B, Yu B, Zhai Q. Role of mitochondrial permeability transition in human hepatocellular carcinoma Hep-G2 cell death induced by rhein. Fitoterapia. 2013;91:68–73.
- Silva FSG, Ribeiro MPC, Santos MS, Pereira PR, Santos-Silva A, Custódio JBA. Acitretin affects bioenergetics of liver mitochondria and promotes mitochondrial permeability transition: potential mechanisms of hepatotoxicity. Toxicol. 2013;306:93–100.10.1016/j.tox.2013.01.020
- Lemasters JJ, Theruvath TP, Zhong Z, Nieminen AL. Mitochondrial calcium and the permeability transition in cell death. Biochim. Biophys. Acta. 2009;1787:1395–1401.10.1016/j.bbabio.2009.06.009
- Young FM, Phungtamdet W, Sanderson BJS. Modification of MTT assay conditions to examine the cytotoxic effects of amitraz on the human lymphoblastoid cell line, WIL2NS. Toxicol. in Vitro. 2005;19:1051–1059.10.1016/j.tiv.2005.05.001
- Labbe G, Pessayre D, Fromenty B. Drug-induced liver injury through mitochondrial dysfunction: mechanisms and detection during preclinical safety studies. Fundam. Clin. Pharmacol. 2008;22:335–353.10.1111/fcp.2008.22.issue-4
- Kroemer G, Galluzzi L, Brenner C. Mitochondrial membrane permeabilization in cell death. Physiol. Rev. 2007;87:99–163.10.1152/physrev.00013.2006
- Indran RI, Tufo G, Pervaiz S, Brenner C. Recent advances in apoptosis, mitochondria and drug resistance in cancer cells. Biochim. Biophys. Acta. 2011;1807:735–745.10.1016/j.bbabio.2011.03.010
- Long TC, Saleh N, Tilton RD, Lowry GV. Titanium dioxide (P25) produces reactive oxygen species in immortalized brain microglia (BV2): implications for nanoparticle neurotoxicity. Environ. Sci. Technol. 2006;40:4346–4352.10.1021/es060589n
- Yang YX, Zheng ZL, Gan JL, Hong LH, Xiu ZW, Zhen HL, Guang BJ, Yun JL. DNA interaction, cytotoxicity, apoptotic activity, cell cycle arrest, reactive oxygen species and mitochondrial membrane potential assay induced by ruthenium(II) polypyridyl complexes. Inorg. Chim. Acta. 2014;405:228–234.
- Ma Y, Zhang J, Zhang Q, Chen P, Song J, Yu S, Liu H, Liu F, Song C, Yang D. Adenosine induces apoptosis in human liver cancer cells through ROS production and mitochondrial dysfunction. Biochem. Biophys. Res. Commun. 2014;448:8–14.10.1016/j.bbrc.2014.04.007
- Shaki F, Hosseini MJ, Ghazi KM, Pourahmad J. Depleted uranium induces disruption of energy homeostasis and oxidative stress in isolated rat brain mitochondria. Metallomics. 2013;5:736–744.10.1039/c3mt00019b
- Shaki F, Hosseini MJ, Ghazi KM, Pourahmad J. Toxicity of vanadium on isolated rat liver mitochondria: a new mechanistic approach. Metallomics. 2013;5:152–166.
- Mashayekhi V, Eskandari MR, Kobarfard F, Khajeamiri A, Hosseini MJ. Induction of mitochondrial permeability transition (MPT) pore opening and ROS formation as a mechanism for methamphetamine-induced mitochondrial toxicity. Naunyn-Schmiedeberg’s Arch. Pharmacol. 2014;387:47–58.10.1007/s00210-013-0919-3
- Xue CB, Liu W, Wu JH, Yang XL, Zeng FD, Xu HB. Chemoprotective effect of N-acetylcysteine (NAC) on cellular oxidative damages and apoptosis induced by nano titanium dioxide under UVA irradiation. Toxicol. in Vitro. 2011;25:110–116.10.1016/j.tiv.2010.09.014
- Fuchs E. Keratins and the skin. Annu. Rev. Cell Dev. Biol. 1995;11:123–154.10.1146/annurev.cb.11.110195.001011
- Sano T, Kume T, Fujimura T, Kawada H, Higuchi K, Iwamura M, Hotta M, Kitahara T, Takema Y. Long-term alteration in the expression of keratins 6 and 16 in the epidermis of mice after chronic UVB exposure. Arch. Dermatol. Res. 2009;301:227–237.10.1007/s00403-008-0914-6