Abstract
Chitinase-A (EaChiA), molecular mass 36 kDa, was purified from the vegetative stems of a horsetail (Equisetum arvense) using a series of column chromatography. The N-terminal amino acid sequence of EaChiA was similar to the lysin motif (LysM). A cDNA encoding EaChiA was cloned by rapid amplification of cDNA ends and polymerase chain reaction. It consisted of 1320 nucleotides and encoded an open reading frame of 361 amino acid residues. The deduced amino acid sequence indicated that EaChiA is composed of a N-terminal LysM domain and a C-terminal plant class IIIb chitinase catalytic domain, belonging to the glycoside hydrolase family 18, linked by proline-rich regions. EaChiA has strong chitin-binding activity, however, no antifungal activity. This is the first report of a chitinase from Equisetopsida, a class of fern plants, and the second report of a LysM-containing chitinase from a plant.
Graphical abstract
There are only two sequences (EaChiA and PrChiA) of LysM-containing chitinase from plant. These are limited to fern. PrChiA exhibited antifungal activity, but EaChiA did not.
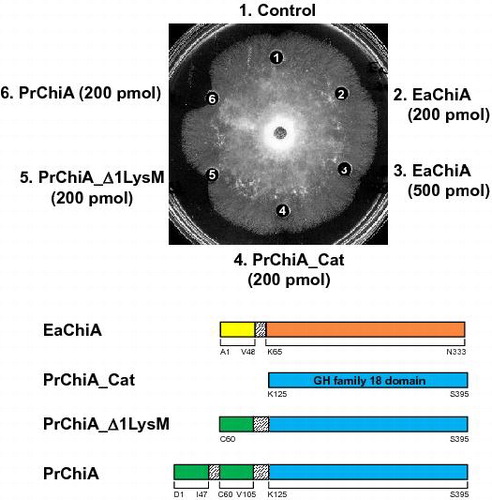
Chitinases (EC 3.2.1.14) catalyze the hydrolysis of chitin, which is a β-1,4-linked homopolymer or oligomer of N-acetylglucosamine (GlcNAc). These enzymes have been divided in two families, the glycoside hydrolase family 18 (GH-18) and 19 (GH-19) based on their catalytic modules, according to the CAZy database (http://www.cazy.org/). GH-18 chitinases are widely distributed in a variety of organisms such as bacteria, fungi, animals, and higher plants. However, GH-19 chitinases have been found mainly in seed plantsCitation1,2) and rarely in bacteria.Citation3) Independent of the CAZy classification, plant chitinases are divided into five classesCitation1,4,5) and two subclassesCitation6,7) based on their amino acid sequences and domain architectures: class I chitinases, consisting of an N-terminal chitin-binding domain and a catalytic domain; class II chitinases, which have only a catalytic domain homologous to that of class I chitinases; class IV chitinases, which share homology with class I chitinases, but are smaller as a result of one deletion in the chitin-binding domain and four deletions of loop regions in the catalytic domain; and class II-L chitinases, which are a low molecular weight subclass of class II due to some deletion(s) of the loop region(s).Citation8) Class III, IIIb, and V chitinases share a DxDxE motif around the catalytic cleft and a (β/α)8 barrel structure, but share very little homology (10–15%). Class III chitinases are majorly GH18 chitinases with three conserved disulfide bonds. Class IIIb chitinases, relatively minor, have no disulfide bond and have a different degradation pattern of chitin oligosaccharides from that of class III chitinases. Class V chitinases have a different chitin oligosaccharide degradation pattern and a big insertion compared to class III and IIIb chitinases.Citation9,10) The catalytic domains of plant class I, II, II-L, and IV belong to GH-19, whereas those of class III, IIIb, and V belong to GH-18. With the exception of one chitinase, plant GH-18 chitinases are only composed of one catalytic domain.
Onaga and TairaCitation11) found a new type of plant chitinase, PrChiA, from Pteris ryukyuensis, a fern. PrChiA is composed of two N-terminal lysin motif (LysM) domains and a C-terminal catalytic domain, belonging to the group of plant class IIIb chitinases, linked by proline, serine, and threonine-rich regions. LysM is a protein domain of about 50 amino acids found initially in several bacterial autolysin proteins.Citation12) These lysin motifs have now been shown to be present in various proteins from bacteria and some eukaryotes.Citation13) According to the CAZy database, LysMs belong to the carbohydrate-binding module family 50 (CBM50). They bind to the GlcNAc residues in bacterial peptidoglycans, in chitin, and/or chitin-related compounds. LysMs of N-acetylglucosaminidase AcmA from Lactococcus lactis were shown to bind to the glycan chain of bacterial peptidoglycan.Citation14) LysM domains from PrChiA were demonstrated to bind to crystal chitin and chitin oligosaccharides.Citation11,15) In the plant kingdom, LysM domains are found in receptors for chitin oligosaccharides and related compounds. It has been reported that these receptors are involved in the interaction between plants and microbes.Citation16–18) However, to date, PrChiA is the only reported plant chitinase-containing LysM domains. In all available databases, LysM-containing plant chitinase genes are not found, except for PrChiA. Whether PrChiA is a special chitinase from a special plant species or PrChiA-like LysM-containing chitinases are widely present in fern plants is of interest. Within fern plants, there are four classes.Citation19) P. ryukyuensis is a species in the Polypodiopsida (major ferns) class. We hypothesized that, if LysM-containing chitinases occur widely throughout fern plants, chitinases will be found in other classes of fern plants. In this study, we determined the presence of chitinase from Equisetum arvense in the Equisetopsida (horsetails), one class of fern plants. Verifying our hypothesis is also very interesting when considering the molecular evolution of plant chitinase.
In this study, we detected chitinase activity in E. arvense. Herein, we describe the successful isolation, characterization, cDNA cloning, and heterologous expression of a chitinase, EaChiA, from E. arvense. This chitinase consists of one LysM domain and a catalytic domain of class IIIb chitinase. This is the second report of a plant GH-18 chitinase having an extra LysM domain. One of the physiological roles of plant chitinases is to protect plants against fungal pathogens by degrading chitin, a major component of the cell wall of many fungi.Citation20) PrChiA exhibited strong antifungal activity and chitin-binding activity. The LysM domains in PrChiA contribute significantly to the antifungal activity of PrChiA through their binding activity to chitin in the cell wall of fungi. In this study, EaChiA exhibited a strong chitin-binding activity, but no antifungal activity. The roles of LysM-containing chitinases in horsetails are discussed.
Materials and methods
Materials
Vegetative stems of E. arvense were collected on the campus of Kinki University in Nara. PrChiA and PrChiA_CatD were prepared as described by Onaga and Taira.Citation11) PrChiA_Δ1LysM was prepared as described by Ohnuma et al.Citation15) Chitin was purchased from Seikagaku Co. (Japan). Escherichia coli (E. coli) BL21(DE3) cells and the expression vector, pET-22b, were purchased from Novagen (Madison, WI, USA). Staphylococcus aureus protease (V8 protease) was purchased from Sigma–Aldrich Co. (St. Louis, MI, USA). All other reagents were of analytic grade.
Protein measurement
Protein concentrations were measured by the bicinchoninic acid method,Citation21) using bovine serum albumin as the standard.
Chitinase activity assay
Chitinase activity was assayed colorimetrically using glycol chitin. Ten microliters of the sample solution was added to 250 μL of 0.2% (w/v) glycol chitin in 0.1 M glycine-HCl buffer, pH 3. After incubation at 37 °C for 15 min, the reducing power of the reaction mixture was measured using ferri-ferrocyanide reagent using the method of Imoto and Yagishita.Citation22) The chitinase activity of each chromatographic fraction was expressed by the absorbance of the reaction mixture at 420 nm (ΔA420). One unit of enzyme activity was defined as the amount of enzyme releasing 1 μmol of GlcNAc per min at 37 °C.
Purification of chitinase from vegetative stems of E. arvense
All procedures were performed in a cold room. Absorbance was measured at 280 nm to monitor the proteins and chitinase activity was measured at pH 3 using glycol chitin during chromatographic separation. Vegetative stems (100 g) of E. arvense were homogenized with 500 mL of deionized water and then left for 4 h at 4 °C. After centrifugation, the supernatant was collected as crude chitinase. The crude chitinase was mixed with an equal volume of 3 M ammonium sulfate solution and the mixture was blended with 10 mL of Butyl-Toyopearl 650M (Tosoh, Japan) resin. After 4 h, the resin with adsorbed proteins was collected with a glass filter, and then packed into a column (1.6 cm × 5 cm). The adsorbed proteins were eluted with 50 mM sodium acetate buffer, pH 5.0. The fractions containing chitinase activity were pooled, applied to a Sephadex G-75 (GE Healthcare) column (2 cm × 140 cm) previously washed with 10 mM sodium acetate buffer, pH 5.0, and developed using the same buffer. The fractions containing chitinase activity were applied to a Mono-Q column (GE Healthcare, 0.5 cm × 5 cm) equilibrated with the dialysis buffer. The column was washed with the same buffer and adsorbed proteins were eluted with a linear gradient of NaCl from 0 to 0.3 M in the same buffer. Several peaks that showed chitinase activity were obtained and the most abundant peak was collected as E. arvense chitinase-A (EaChiA), which was further purified. EaChiA fraction was pooled and mixed with an equal volume of 2 M ammonium sulfate solution. The mixture was applied to a Phenyl Superose column (Pharmacia, UK, 0.5 cm × 5 cm) previously equilibrated with 10 mM sodium acetate buffer, pH 5.0, containing 1 M ammonium sulfate. The column was washed with the same buffer and the adsorbed proteins were then eluted with a linear gradient of ammonium sulfate from 1 to 0 M in the same buffer. The active fraction was collected as EaChiA. The purified EaChiA fraction gave almost single band at a molecular mass of 36 kDa with very minor bands on SDS-polyacrylamide gel electrophoresis (PAGE) (Fig. , lane 1). We judged these minor bands do not get affected to the interpretation of experimental results. One hundred grams of E. arvense vegetative stems yielded 0.01 mg of EaChiA.
Electrophoresis
SDS-PAGE was performed using the method of LaemmliCitation23) using a 15% acrylamide gel. Proteins on the gel were stained with Coomassie brilliant blue R250 (CBB). The molecular mass was measured in the presence of 2-mercaptoethanol using a molecular weight marker kit (Apro Science, Japan).
N-terminal and internal amino acid sequence analysis
N-terminal amino acid sequence analysis was performed by Edman degradation with a protein sequencer (Model PPSQ-23A; Shimadzu, Japan). To obtain internal peptides, in-gel digestion was performed using the method of Cleveland et al.Citation24) Five micrograms of purified EaChiA was applied to the well of SDS-polyacrylamide gel and 0.5 μg of V8 protease was then added to the same well. The EaChiA and protease were electrophoresed to the interface between the stacking and separating gels and then stopped. After 30 min, the electrophoresis was restarted and completed. The digested products were blotted onto a PVDF membrane and detected with CBB staining. To obtain internal sequences of EaChiA, the peptide bands on membrane were applied to a protein sequencer.
HPLC analysis of the hydrolysis products of chitin oligosaccharide [(GlcNAc)n] by chitinases
The products of (GlcNAc)n hydrolysis by chitinases were analyzed by HPLC on a TSKgel amide-80 column (Tosoh, 0.46 cm × 25 cm) using the method of Koga et al.Citation25) After incubation for 30 min at 25 °C, the reaction mixture, consisting of 20 pmol of chitinase and 10 nmol of (GlcNAc)n in 100 μL of 4 mM glycine-HCl buffer, pH 3, was immediately cooled in an ice bath and 5 μL of reaction mixture was applied to the column. Hydrolysis products were eluted with 70% acetonitrile at a flow rate of 0.7 mL/min.
cDNA cloning
The sequences of all primers are presented in Table . Total RNA was isolated from vegetative stems of E. arvense using an RNeasy kit (Qiagen, Valencia, CA, USA). First strand cDNA synthesis was performed on 5 μg of total RNA using a GeneRacer kit (Invitrogen, Carlsbad, CA, USA) with oligo(dT) adapter primer. The resulting cDNA was used as a template for polymerase chain reaction (PCR) amplification with degenerate primers. The PCR was performed with primers P1 (a forward primer designed on the basis of the N-terminal amino acid sequence, ACTSYYTVKSGDICYNIAQT) and P2 (a reverse primer designed on the basis of an internal peptide sequence, HFQSTNEQFA). The resulting PCR products were cloned into a pGEM-T vector (Promega, Madison, WI, USA) and sequenced using the ABI Prism system (Model 3100; Applied Biosystems, Foster City, CA, USA). To obtain the full-length cDNA of EaChiA, both 5′ and 3′ rapid amplification of cDNA ends (RACE) were performed using a GeneRacer kit according to the manufacturer’s instructions. The gene-specific primers P3 (first PCR) and P4 (nested PCR) were used for the 5′ RACE and the gene-specific primers P5 (first PCR) and P6 (nested PCR) for the 3′ RACE. Finally, a cDNA fragment containing the entire coding region of EaChiA cDNA was amplified using the forward primer P7 (designed from the 5′ RACE products) and reverse primer P8 (designed from the 3′ RACE products). The sequences of the resulting PCR products were analyzed using the above-mentioned procedure.
Table 1. Primers for PCR, RACE, and expression.
Expression and purification of recombinant EaChiA in E. coli
We expressed recombinant EaChiA (Ala1–Asn333), an open reading frame without a putative signal sequence) in E. coli. The corresponding cDNA region was amplified by PCR using EaChiA cDNA as the template, with the primers P9 and P10 (P9 is a forward primer containing the NdeI recognition site and P10 is a reverse primer containing the BamHI recognition site). The amplified DNA fragment was digested with NdeI and BamHI and ligated in an expression vector, pET 22b (Novagen), previously digested with the same enzymes. The resulting recombinant plasmids were designated pET-EaChiA. The pET-EaChiA was introduced into E. coli BL21(DE3). E. coli cells harboring the plasmid were grown to A600 = 0.6 before induction with 1 mM IPTG. After induction, growth was continued for 24 h at 15 °C. The cells were harvested by centrifugation, suspended in 20 mM Tris-HCl buffer (pH 7.5), and disrupted with a sonicator. After cell debris were removed by centrifugation (10,000 × g, 10 min), the supernatant was dialyzed against 10 mM sodium acetate buffer, pH 4.0. After dialysis, the resulting insoluble proteins were eliminated by centrifugation at 10,000 × g for 15 min. The supernatant was dialyzed against 10 mM sodium acetate buffer, pH 5.0. The dialysate was applied to a Mono-Q column (0.5 cm × 5 cm) equilibrated with the dialysis buffer. The column was washed with the same buffer and adsorbed proteins were eluted with a linear gradient of NaCl from 0 to 0.3 M in the same buffer. After confirmation of the purity of the corresponding fraction by SDS-PAGE, the fraction was collected as purified recombinant protein.
Chitin binding assay
The chitin-binding ability of the chitinase and its mutant was examined using a chitin column (0.7 cm × 2.5 cm), as described by Yamagami and Funatsu.Citation26) Twenty-five micrograms of the chitinase was dissolved in 10 mM sodium phosphate buffer, pH 7.0, and applied to a chitin column previously equilibrated with the same buffer. After washing the column with the same buffer, the chitin-binding protein was eluted first with 1 M NaCl in the same buffer, secondly with 0.1 M acetic acid, thirdly with 1 M acetic acid, and lastly with a 5 M solution.
Antifungal assay
The antifungal assay was performed according to Schlumbaum et al.Citation20) with modification. An agar disk (6 mm in diameter) containing the fungus Trichoderma viride, prepared from actively growing fungus that had previously been cultured on a potato dextrose broth with 1.5% (w/v) agar (PDA), was placed in the center of a Petri dish containing PDA. The plates were incubated at room temperature for 12 h. Wells were subsequently punched into the agar at a distance of 15 mm from the center of the Petri dish. The samples to be tested were placed into the wells containing 10 μL of sterile water. The plates were incubated for 24 h at room temperature and then photographed.
Results
Properties and the N-terminal amino acid sequence of purified E. arvense chitinase-A
The enzyme was purified to homogeneity by a series of column chromatography (supplementary Fig. 1). Using SDS-PAGE in the presence of 2-mercaptoethanol, the molecular mass of purified EaChiA was found to be 36 kDa (Fig. , lane 1). The optimum pH and the optimum temperature for EaChiA were 2–3 and 50–60 °C, respectively, using glycol chitin as the substrate (Fig. (A) and (C)). pH and temperature stability ranges of EaChiA were from pH 3–9 and up to 50 °C, respectively (Fig. (B) and (D)).
Fig. 2. Effect of pH and temperature on the chitinase activity and stability of the native and recombinant EaChiA.
Notes: (A) The effect of pH on chitinase activity was examined after incubation at 37 °C for 15 min. (B) The effect of pH on stability was examined by measuring residual activity after incubation at various pH at 37 °C for 24 h. (C) The effect of temperature on chitinase activity was examined after incubation for 15 min. (D) The effect of temperature on stability was examined by measuring residual activity after incubation in 0.1 M sodium phosphate buffer, pH 7.0, for 1 h. Methods are described in Materials and Methods. Closed and open circles indicate native and recombinant EaChiA, respectively.
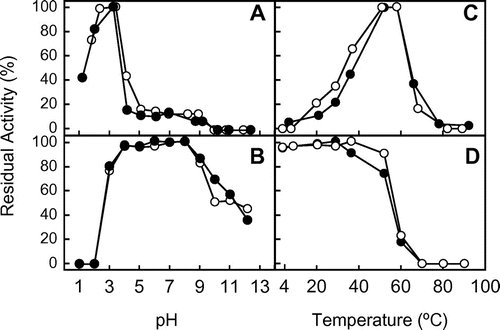
The cleavage patterns of (GlcNAc)n produced by EaChiA and the anomeric form of the products were determined as described in Materials and Methods (Fig. ). The major hydrolysis products from (GlcNAc)6 were (GlcNAc)4, (GlcNAc)3, and (GlcNAc)2, and those from (GlcNAc)5 were mainly (GlcNAc)3 and (GlcNAc)2. A small amount of (GlcNAc)3, (GlcNAc)2, and GlcNAc were detected during (GlcNAc)4 hydrolysis by EaChiA. In this condition, (GlcNAc)3 and (GlcNAc)2 were not degraded by EaChiA (data not shown). Since the newly created reducing end of the products was largely β (e.g. (GlcNAc)4 in Fig. (A) and (B)), it indicated that EaChiA is a retaining enzyme. The amounts and α/β anomer ratio of the products indicated that EaChiA hydrolyzed the third and fourth linkage from the non-reducing end-side of the substrate. These cleavage patterns produced by EaChiA were very similar to those produced by PrChiA and tulip bulb chitinase-1 (TBC-1).Citation11) These enzymes contain a catalytic domain belonging to plant class IIIb chitinase. The results suggest that EaChiA has a class IIIb-like catalytic domain.
Fig. 3. HPLC analysis of the hydrolysis products of (GlcNAc)n by EaChiA.
Notes: S, Standards (GlcNAc)1–6; (A) hydrolysis products of (GlcNAc)6; (B) hydrolysis products of (GlcNAc)5; (C) hydrolysis products of (GlcNAc)4. I to VI indicate (GlcNAc)1–6; α and β indicate the α- and β-anomer, respectively. Standard (GlcNAc)1–6 and the reaction mixtures were analyzed by HPLC on a TSKgel amide-80 column as described in Materials and Methods.
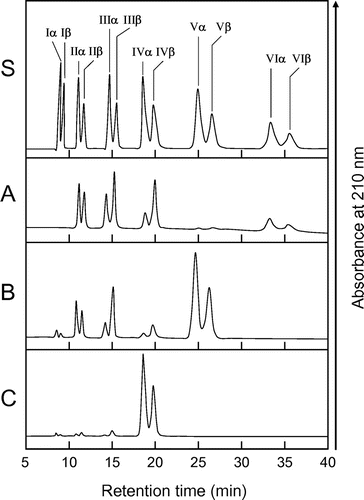
The N-terminal sequence of EaChiA, which was reduced and S-pyridylethylated, was analyzed up to the 20th position with a protein sequencer. The N-terminal sequence of EaChiA was found to be ACTSYYTVKSGDICYNIAQT. This sequence does not share any homology with the sequences of plant chitinases without PrChiA, but shares homology with the LysM of other proteins from several organisms.
The N-terminal sequence and cleavage pattern of the (GlcNAc)n suggested that EaChiA consists of LysM domain(s) at the N-terminal end and a class IIIb-like catalytic domain at the C-terminal end. These results suggest that EaChiA is a LysM-containing plant chitinase.
Cloning and sequence analysis of EaChiA cDNA
A 593-bp putative EaChiA PCR product was obtained using degenerate primers based on the N-terminal amino acid sequence of the purified EaChiA and the internal peptide amino acid sequences obtained by V8 protease digestion of EaChiA. Using gene-specific primers based on the sequence of this fragment, a 324-bp 5′-cDNA fragment and a 732-bp 3′-cDNA fragment were then amplified by RACE and sequenced. The resulting full-length EaChiA cDNA consisted of 1320 nucleotides and encoded an open reading frame coding for 361 amino acid residues (Fig. ). An amino acid sequence corresponding to the N-terminal sequence (Ala1–Thr20) of the purified protein was found in the deduced amino acid sequence from the open reading frame of the cDNA. Thus, the 28 amino acids detected prior to the N-terminal amino acid of the purified protein were predicted to be a signal peptide. Analysis using the computer program signalP (http://www.cbs.dtu.dk/services/SignalP/)Citation27) confirmed that the pre-sequence of 28 amino acids is a signal peptide. The remaining 333 amino acids (Ala1–Asn333) were considered to constitute the EaChiA mature protein.
Fig. 4. Cloning strategy and primary structure of EaChiA.
Notes: (A) Cloning strategy for EaChiA cDNA. Three fragments were overlapped to obtain the complete nucleotide sequence of EaChiA cDNA. The open box represents the coding region and the solid lines represent the 5′- and 3′-noncoding regions. The relative position and direction of each primer are indicated by arrows. The solid lines indicate cDNA fragments amplified by each PCR. SP, signal peptide. (B). The nucleotide sequence of EaChiA cDNA with its deduced amino acid sequence. N-terminal amino acid sequences of native EaChiA and an internal peptide obtained by V8 protease digestion are underlined with dotted lines. The primers used for the cloning of EaChiA cDNA are shown by lines above their sequences. The putative signal peptide at the N-terminal is underlined. The putative poly(A) additional signal is underlined with a double line. (C) Schematic representation of EaChiA. GH indicates glycoside hydrolase.
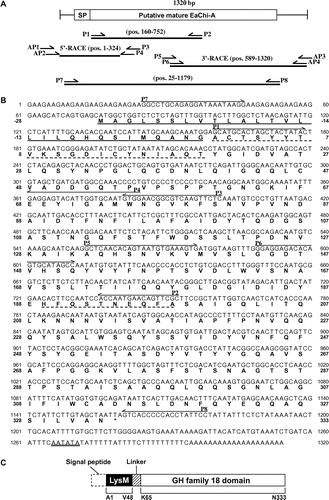
A search of the deduced amino acid sequence of EaChiA against conserved domain database (http://www.ncbi.nlm.nih.gov/Structure/cdd/cdd.shtml)Citation28) showed that it possesses a LysM domain at the N-terminal end and a glycoside hydrolase family-18 domain at the C-terminal end. Multiple alignments of LysM domains from EaChiA and LysM-containing proteins were performed using the ClustalX programCitation29) and the results are shown in Fig. (A). EaChiA LysM domain is homologous to two LysM domains from PrChiA (54% identity) and LysM domain proteins from fungi and nematode (Myceliophthora thermophila, 54%; Podospora anserina, 54%; and Caenorhabditis brenneri, 48%). The alignment of the catalytic domain of EaChiA with PrChiA and TBC-1 is shown in Fig. (B). EaChiA catalytic domain presents some homology with the catalytic domains of PrChiA (60%) and TBC-1 (55%), which are members of the plant class IIIb chitinases (belonging to GH 18 chitinases). Thus, EaChiA consists of an N-terminal LysM domains and a C-terminal catalytic domain that belongs to the group of plant class IIIb chitinases, linked by proline-rich regions. No other chitinase consisting of this domain combination is known among the plant chitinases.
Fig. 5. Alignment of amino acid sequences from domains of EaChiA with those from corresponding regions of several proteins.
Notes: (A) Alignment of sequences from LysM domains of EaChiA with those of LysM-containing proteins. (B) Alignment of the sequence from full length of EaChiA with that from PrChiA to TBC-1, which present a catalytic domain belonging to class IIIb chitinases. Identical residues are shown in white with a black background; dashes indicate gaps. An underline indicates the consensus region around the catalytic site in family-18 glycoside hydrolase. An arrow indicates an expected catalytic residue. PrChi-A 1st and 2nd indicate the first LysM domain and the second LysM domain, respectively, from the N-terminal of PrChiA. EaChiA, E. arvense chitinase-A (NCBI Accession # BAI22848); Mt-LysM, LysM domain-containing protein from M. thermophila (NCBI Accession # XP_003660096); Pa-LysM, LysM-containing protein from P. anserina (NCBI Accession # XP_001907459); Cb-LysM, LysM-containing protein from C. brenneri (NCBI Accession # EGT32879); TBC-1, plant class IIIb chitinase from Tulipa bakeri (NCBI Accession # BAA88408).
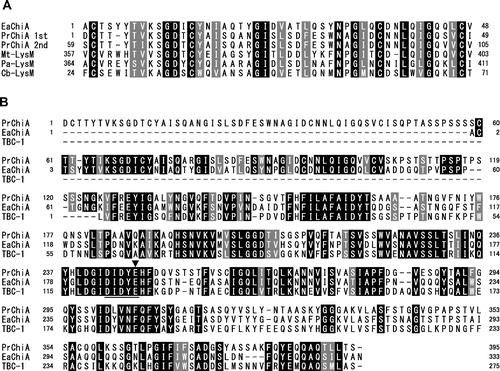
Preparation and characterization of recombinant EaChiA
To determine some of EaChiA properties, we prepared a recombinant EaChiA as described in Materials and Methods. The heterologous expression and isolation of recombinant EaChiA were successful. The molecular mass of recombinant EaChiA was slightly lower than that of native EaChiA (Fig. , lane 2). This result suggested native EaChiA presents some posttranslational modification such as glycosylation. EaChiA does not present any N-glycosylation site with a consensus sequence Asn-Xaa-Thr/Ser, where Xaa can be any amino acid except proline. However, some potential O-glycosylation sites were detected with several O-glycosylation site prediction programs such as NetOGlyc 4.0 Server.Citation30) The specific activities of the native and recombinant EaChiA were 8.9 × 109 and 9.4 × 109 units/mol enzyme, respectively, using glycol chitin as the substrate. As shown in Fig. , the effects of pH and temperature on chitinase activity as well as the pH and thermal stability of the recombinant enzyme were almost similar to that of the native chitinase. These results indicate that the recombinant enzyme was correctly folded in E. coli cells. The yield of recombinant EaChiA was about 10 mg/L of culture.
Antifungal activity of EaChiA
The antifungal activity of EaChiA, PrChiA (two LysM domain with a catalytic domain), PrChiA_Δ1LysM (single LysM domain with a catalytic domain), and PrChiA_CatD (a catalytic domain only) was determined using the hyphal extension inhibition assay on agar plates with T. viride as the test fungus (Fig. ). PrChiA and PrChiA_Δ1LysM inhibited hyphal extension, but EaChiA and PrChiA_CatD did not.
Fig. 6. Antifungal activity of EaChiA, PrChiA, and PrChiA mutants against T. viride.
Notes: Samples to be tested were placed in wells in 10 μL of distilled water. (1) blank (distilled water); (2) EaChiA (200 pmol); (3) EaChiA (500 pmol); (4) PrChiA_CatD (200 pmol); (5) PrChiA_Δ1LysM (200 pmol); (6) PrChiA (200 pmol).
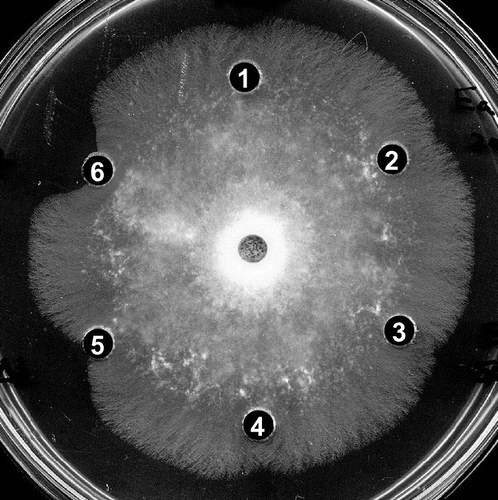
Chitin-binding activities of EaChiA
EaChiA, PrChiA, PrChiA_Δ1LysM, and PrChiA_CatD were applied to a chitin affinity column chromatography. EaChiA, PrChiA, and PrChiA_Δ1LysM were adsorbed to the chitin column and were then eluted with a 5, 1, and 0.1 acetic acid solution, respectively (Fig. (A)–(C)). The results indicate that the chitin-binding ability of EaChiA is higher than that of PrChiA and PrChiA_Δ1LysM. Under the same conditions, PrChiA_CatD was found in the flow-through fraction. These results imply that the LysM domain and/or the catalytic domain of EaChiA present a stronger affinity for chitin than those of PrChiA.
Fig. 7. Elution profiles of EaChiA, PrChiA, and PrChiA mutants in affinity chromatography on a chitin column.
Notes: The proteins were applied to a chitin column equilibrated with 10 mM phosphate buffer, pH 7.0. After washing out the unadsorbed proteins with the same buffer, the adsorbed proteins were eluted successively with 1 M NaCl, 0.1, 1, and 5 acetate. (A) EaChiA; (B) PrChiA; (C) PrChiA_Δ1LysM; (D) PrChiA_CatD.
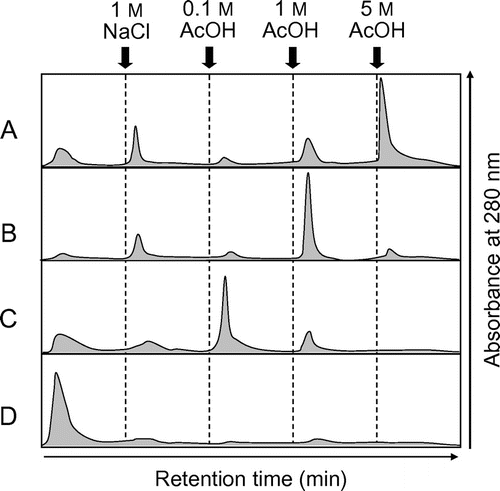
Discussion
EaChiA primary structure
In this study, we demonstrated the existence of a LysM-containing plant chitinase, EaChiA, found in a horsetail. The chitinase consists of an N-terminal LysM domain and a C-terminal catalytic domain of GH family-18 chitinases. This domain combination is extremely rare in the plant kingdom. According to the results of our search of the web database using the conserved domain architecture retrieval tool (CDART)Citation31) service, there are 1141 sequences of LysM-containing GH family18 chitinase in all organisms. Of these, 864 and 277 proteins are from bacteria and fungi, respectively. In other organisms, the number of proteins from nematode, ameba, and green algae are 4, 2, and 1, respectively. LysM-containing chitinase has not been found in available sequence data from seed plants, lycophytes, and moss plants, including model plants such as Arabidopsis thaliana, Oryzae sativa, Selaginella moellendorffii, and Physcomitrella patens for which the whole-genome sequences have been published. There are only two sequences of LysM-containing GH family18 chitinase from plant. One is EaChiA, the other is PrChiA. Pryer et al.Citation32) showed that there are three monophyletic groups of extant vascular plants: seed plants, lycophytes, and ferns (seedless vascular plants without lycophytes). This refutes the notion that ferns are transitional evolutionary grades between bryophytes and seed plants. Smith et al.Citation19) described that, within ferns, there are four classes, Polypodiopsida (major ferns), Equisetopsida (horsetail), Psilotopsida (whisk fern and grapeferns), and Marattiopsida (marattioid ferns). E. arvense and P. ryukyuensis are species in the Equisetopsida and the Polypodiopsida classes, respectively. It is interesting to note that the existence of LysM-containing chitinases is limited to fern plants. LysM-containing chitinase might be gained solely in fern plants during plant evolution.
EaChiA antifungal activity
PrChiA has antifungal activity, but EaChiA does not. The major difference in structure between PrChiA and EaChiA is the number of LysM domains. PrChiA has two LysM domains at the N-terminal end, while EaChiA has only one LysM domain. We first thought that the lack of antifungal activity of EaChiA was due to the number of LysM domains. However, the PrChiA mutant PrChiA_Δ1LysM, consisting of one LysM domain and one catalytic domain, certainly exhibited antifungal activity (Fig. , well 5). Chitinase activity of EaChiA using glycol chitin was almost the same as that of PrChiA (EaChiA, 0.94 × 1010 units/mol; PrChiA, 1.32 × 1010 units/mol). (GlcNAc)n degradation patterns obtained with EaChiA are almost similar to those obtained with PrChiA. Onaga and TairaCitation11) showed that chitin-binding ability of PrChiA is almost dependent on LysM domains. They described that the LysM domains contribute significantly to the antifungal activity of PrChiA through their binding activity to chitin in the cell wall of fungi. In this study, the affinity of PrChiA_Δ1LysM to chitin was lower than that of PrChiA (Fig. ). These findings suggest that the LysM domain has chitin-binding activity and higher numbers of LysM domains exhibit higher chitin-binding ability. Surprisingly, chitin-binding of EaChiA was stronger than that of PrChiA, despite a lower number of LysM domains. We do not know the cause of this strong chitin-binding ability. However, we speculate that high chitin-binding ability of EaChiA may influence its antifungal activity. Now, to clarify this conflicting phenomenon, we are trying domain-swapping analysis between EaChiA and PrChiA.
Possible physiological role of EaChiA
Considering that EaChiA has no antifungal activity, but a strong chitin-binding activity, its physiological roles remain unclear. Chitin-binding and chitin-degrading ability of EaChiA may relate to symbiosis with microbes such as mycorrhizal fungi. Some family-18 chitinases in Medicago truncatula have been reported to be specifically transcribed during interactions with mycorrhizal fungi.Citation33) De Jonge et al.Citation34) showed that the LysM domain-containing effector protein Ecp6 of the fungal plant pathogen Cladosporium fulvum sequesters chitin oligosaccharides that are released from the cell walls of invading hyphae to prevent elicitation of host immunity. The LysM domain-containing effector protein MgLysM of fungus Mycosphaerella graminicola blocked the elicitation of chitin-induced plant defenses and also protected fungal hyphae against plant-derived hydrolytic enzymes.Citation35) EaChiA, having no antifungal activity, but a strong chitin-binding activity and high homology to fungal LysM protein, may contribute to symbiosis with mycorrhizal fungi by preventing plant defense response through binding to the chitin in the fungal cell walls and protect them against other chitinases. Chitinase-active fractions without EaChiA were obtained from the chitinase purification process in this study (Supplementary Fig. 1). The physiological roles of LysM-containing chitinases in ferns should be clarified by future structural and physiological studies on fern chitinases containing EaChiA and PrChiA.
Supplemental material
The supplemental material for this paper is available at http://dx.doi.org/10.1080/09168451.2015.1025693.
RENG_A_1010436.pdf
Download PDF (80.9 KB)Supplementary Material
Download PDF (50.5 KB)Acknowledgments
We thank Chihiro Kato (University of the Ryukyus) for technical assistance.
Disclosure statement
No potential conflict of interest was reported by the authors.
Notes
Abbreviations: EaChi, Equisetum arvense chitinase; PrChi, Pteris ryukyuensis chitinase; PAGE, polyacrylamide gel electrophoresis; CBB, Coomassie brilliant blue; RACE, rapid amplification of cDNA ends; PDA, potato dextrose broth with agar; GlcNAc, N-acetylglucosamine; LysM, lysine motif; TBC, tulip bulb chitinase; HPLC, high performance liquid chromatography.
References
- Collinge DB, Kragh KM, Mikkelsen JD, Nielsen KK, Rasmussen U, Vad K. Plant chitinases. Plant J. 1993;3:31–40.10.1046/j.1365-313X.1993.t01-1-00999.x
- Graham LS, Sticklen MB. Plant chitinases. Can. J. Bot. 1994;72:1057–1083.10.1139/b94-132
- Kawase T, Yokokawa S, Saito A, Fujii T, Nikaidou N, Miyashita K, Watanabe T. Comparison of enzymatic and antifungal properties between family 18 and 19 chitinases from S. coelicolor A3(2). Biosci. Biotechnol. Biochem. 2006;70:988–998.10.1271/bbb.70.988
- Shinshi H, Neuhaus J-M, Ryals J, Meins F, Neuhas JM, Ryals J, Meins F. Structure of a tobacco endochitinase gene: Evidence that different chitinase genes can arise by transposition of sequences encoding a cysteine-rich domain. Plant Mol. Biol. 1990;14:357–368.10.1007/BF00028772
- Melchers L, Groot M. A new class of tobacco chitinases homologous to bacterial exo-chitinases displays antifungal activity. Plant J. 1994;5:469–480.10.1046/j.1365-313X.1994.5040469.x
- Yamagami T, Mine Y, Ishiguro M. Complete amino acid sequence of chitinase-a from bulbs of gladiolus (Gladiolus gandavensis). Biosci. Biotechnol. Biochem. 1998;62:386–389.10.1271/bbb.62.386
- Taira T. Structures and antifungal activity of plant chitinases. J. Appl. Glycosci. 2010;57:167–176.10.5458/jag.57.167
- Taira T, Mahoe Y, Kawamoto N, Onaga S, Iwasaki H, Ohnuma T, Fukamizo T. Cloning and characterization of a small family 19 chitinase from moss (Bryum coronatum). Glycobiology. 2011;21:644–654.10.1093/glycob/cwq212
- Taira T, Hayashi H, Tajiri Y, Onaga S, Uechi G, Iwasaki H, Ohnuma T, Fukamizo T. A plant class V chitinase from a cycad (Cycas revoluta): biochemical characterization, cDNA isolation, and posttranslational modification. Glycobiology. 2009;19:1452–1461.10.1093/glycob/cwp119
- Ohnuma T, Numata T, Osawa T, Mizuhara M, Lampela O, Juffer AH, Skriver K, Fukamizo T. A class V chitinase from Arabidopsis thaliana: gene responses, enzymatic properties, and crystallographic analysis. Planta. 2011;234:123–137.10.1007/s00425-011-1390-3
- Onaga S, Taira T. A new type of plant chitinase containing LysM domains from a fern (Pteris ryukyuensis): roles of LysM domains in chitin binding and antifungal activity. Glycobiology. 2008;18:414–423.10.1093/glycob/cwn018
- Joris B, Englebert S, Chu CP, Kariyama R, Daneo-Moore L, Shockman GD, Ghuysen JM. Modular design of the Enterococcus hirae muramidase-2 and Streptococcus faecalis autolysin. FEMS Microbiol. Lett. 1992;91:257–264.10.1111/fml.1992.91.issue-3
- Bateman A, Bycroft M. The structure of a LysM domain from E. coli membrane-bound lytic murein transglycosylase D (MltD). J. Mol. Biol. 2000;299:1113–1119.10.1006/jmbi.2000.3778
- Steen A, Buist G, Leenhouts KJ, El Khattabi M, Grijpstra F, Zomer AL, Venema G, Kuipers OP, Kok J. Cell wall attachment of a widely distributed peptidoglycan binding domain is hindered by cell wall constituents. J. Biol. Chem. 2003;278:23874–23881.10.1074/jbc.M211055200
- Ohnuma T, Onaga S, Murata K, Taira T, Katoh E. LysM domains from Pteris ryukyuensis chitinase-A: a stability study and characterization of the chitin-binding site. J. Biol. Chem. 2008;283:5178–5187.10.1074/jbc.M707156200
- Limpens E, Franken C, Smit P, Willemse J, Bisseling T, Geurts R. LysM domain receptor kinases regulating rhizobial nod factor-induced infection. Science. 2003;302:630–633.10.1126/science.1090074
- Radutoiu S, Madsen LH, Madsen EB, Felle HH, Umehara Y, Grønlund M, Sato S, Nakamura Y, Tabata S, Sandal N, Stougaard J. Plant recognition of symbiotic bacteria requires two LysM receptor-like kinases. Nature. 2003;425:585–592.10.1038/nature02039
- Kaku H, Nishizawa Y, Ishii-Minami N, Akimoto-Tomiyama C, Dohmae N, Takio K, Minami E, Shibuya N. Plant cells recognize chitin fragments for defense signaling through a plasma membrane receptor. Proc. Nat. Acad. Sci. USA. 2006;103:11086–11091.10.1073/pnas.0508882103
- Smith AR, Pryer KM, Schuettpelz E, Korall P, Schneider H, Wolf PG. A classification for extant ferns. Taxon. 2006;55:705–731.
- Schlumbaum A, Mauch F, Vögeli U, Boller T. Plant chitinases are potent inhibitors of fungal growth. Nature. 1986;324:365–367.10.1038/324365a0
- Smith PK, Krohn RI, Hermanson GT, Mallia AK, Gartner FH, Provenzano MD, Fujimoto EK, Goeke NM, Olson BJ, Klenk DC. Measurement of protein using bicinchoninic acid. Anal. Biochem. 1985;150:76–85.10.1016/0003-2697(85)90442-7
- Imoto T, Yagishita K. A simple activity measurement of lysozyme. Agric. Biol. Chem. 1971;35:1154–1156.10.1271/bbb1961.35.1154
- Laemmli UK. Cleavage of structural proteins during the assembly of the head of bacteriophage T4. Nature. 1970;227:680–685.10.1038/227680a0
- Cleveland D, Fischer S, Kirschner M, Laemmli U. Peptide mapping by limited proteolysis in sodium dodecyl sulfate and analysis by gel electrophoresis. J. Biol. Chem. 1977;252:1102–1106.
- Koga D, Yoshioka T, Arakane Y. HPLC analysis of anomeric formation and cleavage pattern by chitinolytic enzyme. Biosci. Biotechnol. Biochem. 1998;62:1643–1646.10.1271/bbb.62.1643
- Yamagami T, Funatsu G. Limited proteolysis and reduction-carboxymethylation of rye seed chitinase-a: role of the chitin-binding domain in its chitinase action. Biosci. Biotechnol. Biochem. 1996;60:1081–1086.
- Bendtsen JD, Nielsen H, von Heijne G, Brunak S. Improved prediction of signal peptides: SignalP 3.0. J. Mol. Biol. 2004;340:783–795.10.1016/j.jmb.2004.05.028
- Marchler-Bauer A, Anderson JB, Cherukuri PF, DeWeese-Scott C, Geer LY, Gwadz M, He S, Hurwitz DI, Jackson JD, Ke Z, Lanczycki CJ, Liebert CA, Liu C, Lu F, Marchler GH, Mullokandov M, Shoemaker BA, Simonyan V, Song JS, Thiessen PA, Yamashita RA, Yin JJ, Zhang D, Bryant SH. CDD: a conserved domain database for protein classification. Nucleic Acids Res. 2005;33:D192–D196.
- Thompson JD, Gibson TJ, Plewniak F, Jeanmougin F, Higgins DG. The CLUSTAL_X windows interface: flexible strategies for multiple sequence alignment aided by quality analysis tools. Nucleic Acids Res. 1997;25:4876–4882.10.1093/nar/25.24.4876
- Steentoft C, Vakhrushev SY, Joshi HJ, Kong Y, Vester-Christensen MB, Schjoldager KT, Lavrsen K, Dabelsteen S, Pedersen NB, Marcos-Silva L, Gupta R, Bennett EP, Mandel U, Brunak S, Wandall HH, Levery SB, Clausen H. Precision mapping of the human O-GalNAc glycoproteome through SimpleCell technology. EMBO J. 2013;32:1478–1488.10.1038/emboj.2013.79
- Geer LY, Domrachev M, Lipman DJ, Bryant SH. CDART: protein homology by domain architecture. Genome Res. 2002;12:1619–1623.10.1101/gr.278202
- Pryer K, Schneider H, Smith A, Cranfill R. Horsetails and ferns are a monophyletic group and the closest living relatives to seed plants. Nature. 2001;409:618–622.10.1038/35054555
- Salzer P, Bonanomi A, Beyer K, Vögeli-Lange R, Aeschbacher RA, Lange J, Wiemken A, Kim D, Cook DR, Boller T. Differential expression of eight chitinase genes in Medicago truncatula roots during mycorrhiza formation, nodulation, and pathogen infection. Mol. Plant Microbe Interact. 2000;13:763–777.10.1094/MPMI.2000.13.7.763
- de Jonge R, van Esse HP, Kombrink A, Shinya T, Desaki Y, Bours R, van der Krol S, Shibuya N, Joosten MHAJ, Thomma BPHJ. Conserved fungal LysM effector Ecp6 prevents chitin-triggered immunity in plants. Science. 2010;329:953–955.10.1126/science.1190859
- Marshall R, Kombrink A, Motteram J, Loza-Reyes E, Lucas J, Hammond-Kosack KE, Thomma BPHJ, Rudd JJ. Analysis of two in planta expressed LysM effector homologs from the fungus Mycosphaerella graminicola reveals novel functional properties and varying contributions to virulence on wheat. Plant Physiol. 2011;156:756–769.10.1104/pp.111.176347