Abstract
Oxidative stress can cause injury in retinal endothelial cells. Salidroside is a strong antioxidative and cytoprotective supplement in Chinese traditional medicine. In this study, we investigated the effects of salidroside on H2O2-induced primary retinal endothelial cells injury. Salidroside decreased H2O2-induced cell death, and efficiently suppressed cellular ROS production, malondialdehyde generation, and cell apoptosis induced by H2O2 treatment. Salidroside induced the intracellular mRNA expression, protein expression, and enzymatic activities of catalase and Mn-SOD and increased the ratio of Bcl2/Bax. Our results demonstrated that salidroside protected retinal endothelial cells against oxidative injury through increasing the Bcl2/Bax signaling pathway and activation of endogenous antioxidant enzymes. This finding presents salidroside as an attractive agent with potential to attenuate retinopathic diseases.
Salidroside protected primary-cultured retinal endothelial cells against H2O2-induced apoptosis and oxidation.
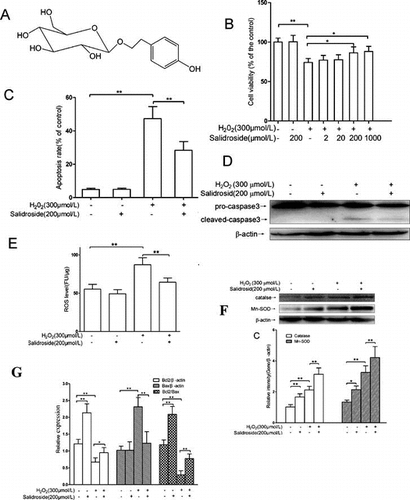
Introduction
The generation of molecular oxygen in the form of ROS is a natural part of aerobic life that is responsible for the manifestation of cellular functions ranging from signal transduction pathways, defense against invading microorganisms, and gene expression to the promotion of growth or death.Citation1) Nevertheless, an excessive amount of ROS is highly toxic to cells. Cells contain numerous antioxidant defenses to minimize fluctuations in ROS; however, ROS generation often exceeds the cell’s defense capacity, resulting in a condition termed oxidative stress.Citation2) Oxidative stress affects the major cellular components: proteins, lipids, and DNA. The importance of oxidative stress is commonly emphasized in the pathogenesis of various degenerative diseases, such as diabetes, cancer, cardiovascular disorders, or neurodegenerative diseases.Citation3) Therefore, the development of an effective antioxidant strategy to reduce oxidative stress, which has attracted considerable interest from investigators, may provide insights into delaying or preventing cell necrosis and apoptosis-induced oxidative stress.Citation4)
Salidroside is a phenylpropanoid glycoside (Fig. ), which is extracted from Rhodiola rosea L, a popular medicinal plant that grows in regions of high altitude such as Tibet.Citation5) Salidroside is known as an medicinal supplement, which could possibly help the body adapt to stress, treat altitude sickness, fatigue, and depression.Citation6) In addition, salidroside has been shown to protect against tissues damage and dysfunction induced by excess oxidative stress.Citation6–9)
ROS can cause damages to the RECs.Citation10) It is generally believed that oxidative stress directly activates a cascade of biochemical and molecular events, including polyol flow and the hexosamine pathway, advanced glycation end products and their receptor, pro-inflammatory factors, and protein kinase C signaling, which lead to retinal vascular endothelial cell injury.Citation11) Despite being not a free radical by itself, hydrogen peroxide (H2O2), a main source of ROS, can alter the intracellular redox state of cells, inducing oxidative damage by its conversion into a highly reactive hydroxyl radical, . H2O2 stimulation has been extensively used to furnish a cell culture model of oxidative stress for studying the biological and pathological processes. In addition, the level of H2O2 and
in mitochondria is elevated during the pathogenesis of diabetic retinopathy.Citation12–14) However, the mechanism of action of salidroside on H2O2-induced RECs injury remains unclear. In this study, the primary-cultured RECs were challenged by H2O2 to study the potential mechanisms of salidroside-mediated actions in blunting redox injury. Our results indicated salidroside significantly inhibits H2O2-induced apoptosis and ROS production in RECs.
Materials and methods
Reagents
Salidroside (purity ≥ 98%) was from Yuanye Biotech CO. LTD (Shanghai, China); Cell proliferation reagents including WST-1 and TUNEL were from Roche (Roche Applied Science, Indianapolis, IN, USA); TRIzol® Reagent and DNaseI were from Invitrogen (Carlsbad, CA, USA); dNTP was from Takara Bio Inc (Shiga, Japan); iQTM SYBR® Green Supermix from Bio-Rad Laboratories, Inc. (Hercules, CA, USA); Oligo (dT) 15 primer and SuperScriptTM II reverse transcriptase were from Promega; Kits for MDA content detection, activity assays of catalase and Mn-SOD were from Nanjing Jiancheng Bioengineering Institute (Nanjing, China); Caspase-3, Bcl-2, Bax, Mn-SOD, catalase, and β-actin antibodies (species cross-reactivity: Human, Mouse and Rat) were from Cell Signaling Technologies (Beverly, MA, USA); PECAM-1 and vWF antibodies were from Santa Cruz Biotechnology, Inc. (USA); Dynabeads coated with IgG (5 × 108 beads/ml) was from Miltenyi Biotec (Bergisch Gladbach, Germany); All pairs of real-time PCR primers were synthesized by Shenggong Biotechnology (Shanghai, China). This study was approved by the Ethics Review Committee of Sichuan University and was in adherence to the tenets of the Declaration of Helsinki.
Isolation and treatment of primary-cultured retinal endothelial cells
Three-week-old male Sprague–Dawley (SD) rats were obtained from the Institute of Laboratory Animal Sciences, West China School of Medicine of Sichuan University. Primary RECs were isolated from SD rats and cultured as previously described.Citation15,16) Briefly, eyes were enucleated and hemisected, and the retinas were removed under a dissecting microscope, placed in HBSS containing penicillin/streptomycin (Sigma, St. Louis, MO) and rinsed. Twelve retinas were digested with 0.5% collagenase type I (Worthington, Lakewood, NJ) in serum-free DMEM, for 40–45 min at 37 °C. Then, endothelial cell medium containing 1% ECM (ScienCell Research Laboratories, San Diego, CA), which contained 5% fetal bovine serum, 100 mg/mL streptomycin, and 100 U/mL penicillin, was added. After filtering through 70 and 40-μm nylon mesh (Becton, Dickinson and Company, USA), the collected retinal cells were resuspended in ECM and incubated with goat anti-mouse magnetic beads pre-coated with mouse anti-rat PECAM-1. Then, the beads bound cells were washed with HBSS for at least 6 times. After the addition of ECM, the cell suspension was seeded on 6-well plates pre-coated with human fibronectin (Prospecbio, Israel). The identity of REC was confirmed by a positive immunofluorescence staining of vWF antibody. The cells were refreshed every 2 days, and passage 2–4 of RECs was used for all experiments. Cells were treated with either 300 μmol/L H2O2 (dissolved in normal saline) for 4 h, with or without pre-incubation of 200 μmol/L salidroside (dissolved in normal saline) for 12 h. The concentrations of H2O2 and salidroside were determined in the pilot studies, the incubation periods were decided according to the previously reported method.
Measurement of cell viability
The WST assay was used to detect cell viability as described previously.Citation17) In brief, RECs were incubated with 10 μL of reconstituted WST-1 mixture for 1 h at 37 °C, and the values of absorption were measured at 440 nm (background at 630 nm was subtracted) by use of a synergyTM Multi-mode Microplate reader (Biotek, VT, USA). All experiments were performed in triplicates. Cell viabilities were presented as percentage of the controls.
Measurement of intracellular ROS
The level of intracellular ROS was quantified using a ROS assay kit. DCFH-DA, a fluorescent probe, is oxidized by ROS in viable cells to 2′,7′-dichlorofluorescein (DCF). Following treatment with H2O2 alone or H2O2 and salidroside, RECs were incubated with 10 μM H2DCFDA (Invitrogen) for 30 min at 37 °C. The intracellular fluorescence intensity was immediately recorded on a synergyTM Multi-mode Microplate reader (Biotek).
MDA and SOD analysis
RECs were lyzed with the help of an ultrasonic device. The protein concentration of the lysate was measured by a BCA protein assay kit (Shanghai Generay Biotech Co., Ltd). The MDA content, activities of catalase, and Mn-SOD were detected following the reagent manufacturers’ instructions. Both Mn-SOD and catalase activities were expressed as units per milligram protein, and MDA content was expressed as nmol per milligram protein, respectively.
Evaluation of cell apoptosis by TUNEL staining
Apoptosis of RECs fixed by acetone was determined by the TUNEL method (Roche Applied Scientific, IN, USA) following reagent manufacturers’ instructions. High-power fields (12–20 at ×400 magnification) were obtained to measure the number of TUNEL-positive nuclei, respectively. Only nuclei that were clearly purplish red were scored. The percentage of TUNEL-positive cells was calculated as the ratio between TUNEL-positive and DAPI (Roche, Germany)-stained nuclei × 100.
Evaluation of cell apoptosis and necrosis by AV-FITC and PI staining
Apoptosis and necrosis were further detected by use of an annexin-V (AV)–fluorescein isothiocyanate (FITC)/propidium iodide (PI) stain detection kit (KeyGen Biotech, Nanjing, China). In brief, RECs incubated in 96-well plates were incubated with AV-FITC and PI for 10 min at room temperature in the dark. Cells were photographed under both phase-contrast and fluorescence microscopy, and total cells were counted. Apoptotic cells were identified as AV-FITC positive and PI negative (FITC+PI−, green-stained cells), and necrotic cells as positive for PI (PI+, red-stained cells). About 200–300 randomly selected cells in each group were counted by an independent investigator who did not know the treatment. Each experiment was conducted in triplicate, and data were averaged.
Quantitative real-time PCR assay
Total RNA was extracted from retinal endothelial cells using Trizol reagent (Invitrogen). We performed real-time quantitative PCR analysis as previously describedCitation18) (primers in Table ). The expressions of GAPDH were used as endogenous reference in normalizing the relative expression of other genes.
Table 1. Sequences of the primers used for real-time PCR amplification.
Western blot analysis
Western blot was performed following the method we previously used.Citation17) RECs were lyzed with RIPA buffer at 4 °C. The supernatant was collected, and the protein concentration was determined using a BCA protein assay kit (Shanghai Generay Biotech Co., Ltd). Equal amounts of protein were loaded onto 12% sodium dodecyl sulfate-polyacrylamide gels for electrophoresis (SDS-PAGE) and transferred onto 0.22-μm PVDF membrane. The membranes were probed with antibodies against caspase-3, catalase, Mn-SOD, Bcl-2, Bax, and β-actin, respectively. Following washing, the membranes were incubated with horseradish peroxidase-conjugated secondary antibody. Proteins were detected using an enhanced chemiluminescence (ECL) system (EMD Millipore, MA, USA). All experiments were repeated three times.
Statistical analysis
Results were shown as mean ± SD. Statistical analyses between groups of two were done by unpaired t-test. Groups of three or more were analyzed by use of one-way ANOVA, followed by the Newman–Keuls multiple comparison test. A p-value <0.05 was considered statistically significant.
Results
Salidroside alleviated H2O2-induced primary-cultured RECs damage
The WST cell viability assay has been shown to be a sensitive and accurate method for cytotoxicity.Citation19) WST assay revealed the dose-dependent cytotoxicity of H2O2 (0–700 μmol/L) on primary-cultured RECs (Fig. (A)). Compared to the controls, stimulation with 300 μmol/L H2O2 for 4 h resulted in 25% loss of cell viability (p < 0.01); therefore, exposure to 300 μmol/L H2O2 for 4 h was used in the subsequent experiments to induce cell insults.
Fig. 2. Salidroside protected primary-cultured RECs against H2O2-induced apoptosis.
Notes: (A) After incubation with H2O2 (0–700 μmol/L) for 4 h, REC viability was measured by WST-1 assay. (B) Effects of salidroside on cell viability measured by WST-1 assay (n = 5/group). (C) Intracellular apoptotic nuclei detected by TUNEL assay. Yellow arrows indicate the purplish red-stained TUNEL-positive nuclei. Scale bars = 50 μm, Original magnifications ×400 (n = 5/group). (D) Quantitative analysis of RECs apoptosis. (E) Pro- and cleaved caspase 3 proteins detected by Western blotting. RECs were treated by salidroside alone for 12 h, or H2O2 alone for 4 h, or salidroside pre-incubation for 12 h and then co-incubation with H2O2 for another 4 h. (F) Densitometric analysis of cleaved caspase-3 (n = 5/group). *p < 0.05, **p < 0.01.
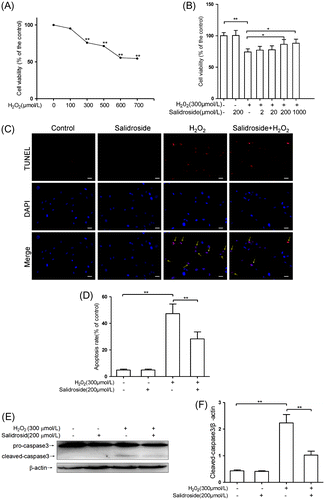
As shown in Fig. (B), salidroside (200 μmol/L) treatment alone had no significant effect on the viability of REC compared with the control (p > 0.05). However, REC cells were pre-treated with various concentrations of salidroside (2, 20, 200 or 1000 μmol/L) for 12 h before treatment with H2O2 for 4 h, cell viability increased in a dose-dependent manner. Pre-treatment with 200 μmol/L salidroside significantly raised the cell viability up to 86.1% of the levels observed in control cells (p < 0.01). Therefore, the 200 μmol/L salidroside was used in the subsequent experiments.
Salidroside protected REC against H2O2-induced apoptosis
The REC apoptosis was evaluated by TUNEL staining. As shown in Fig. (C) and (D), compared with the control, salidroside (200 μmol/L) treatment alone had no significant effect on REC apoptosis (p > 0.05), whereas 4-h stimulation of H2O2-induced apoptosis, with 8.7-fold more apoptotic cells than that of the control (p < 0.01). Pre-incubation with salidroside for 12 h, and then together with H2O2 for another 4 h, significantly attenuated the apoptosis induced by H2O2, with 40.2% less apoptotic cells compared to the cells treated with H2O2 alone (p < 0.01). In addition, we examined the RECs apoptosis and necrosis using AV-FITC and PI staining. As illustrated in supplementary Fig. , compared to the control group, stimulation of H2O2 for 4 h dramatically elevated the ratios of necrotic (PI+) and apoptotic (FITC+PI−) cells by 5.0-fold and 4.0-fold, respectively (both p < 0.01). Pre-treatment with 200 μmol/L salidroside for 12 h significantly decreased the ratios of PI+ (34.4%) and FITC+PI− (32.8%) cells than the treatment with H2O2 alone (p < 0.01).
Caspase-3 is the one of the major proteases in apoptotic pathways.Citation20) We detected the expression of cleaved caspase-3 by Western blotting. As shown in Fig. (E) and (F), compared with the control, 200 μmol/L salidroside treatment alone had no effect on cleaved caspase-3 (p > 0.05), whereas 4 h H2O2 stimulation induced a 4.2-fold increase in cleaved caspase-3 (p < 0.01). Pre-incubation with salidroside for 12 h followed by co-incubation with H2O2 for 4 h caused a significant reduction in cleaved caspase-3, with a 54.3% decrease as compared to stimulation with H2O2 alone (p < 0.01), suggesting the suppressive effect of salidroside on H2O2-induced cell death.
Salidroside ameliorated H2O2-induced oxidative stress
MDA is widely used as an indicator of lipid peroxidation.Citation21) As shown in Fig. (A), compared with the control, 200 μmol/L salidroside treatment alone did not affect MDA levels, whereas exposure to H2O2 for 4 h induced a 3.0-fold increase in MDA levels (p < 0.01). Salidroside pre-treatment for 12 h caused an apparently significant decrease in MDA levels (p < 0.01), with 27.6% lower than that in cells treated with H2O2 alone for 4 h.
Fig. 3. Effects of salidroside on oxidative stress markers, MDA, and ROS in primary-cultured RECs.
Notes: (A) MDA contents in retinal endothelial cells (n = 5/group). (B) Intracellular ROS was measured using H2DCFDA and normalized to the protein contents in cell lysate (n = 6/group). **p < 0.01.
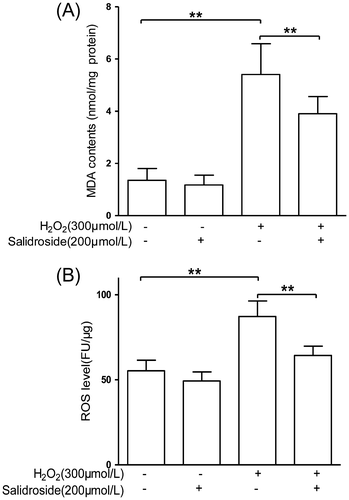
The ROS levels in RECs were further measured directly using of DCFH-DA staining. As shown in Fig. (B), compared to the control cells, salidroside treatment alone had no effect on intracellular ROS levels, whereas H2O2 exposure for 4 h induced a significantly elevated intracellular ROS production, with 58.2% higher than that in the control cells (p < 0.01). However, the ROS elevation was effectively reduced by 26.4% (p < 0.05) with salidroside pretreatment for 12 h followed by co-incubation with H2O2 for 4 h.
Salidroside enhanced the expressions and activities of catalase and Mn-SOD
Catalase and Mn-SOD are the endogenous antioxidative enzymes, which protect against the damage induced by ROS.Citation22) As shown in Fig. (A), compared with the controls, RECs were treated with 200 μmol/L salidroside alone for 12 h, intracellular mRNA expressions of catalase and Mn-SOD elevated by 77.5% (p < 0.01) and 37.8% (p < 0.05), respectively; 300 μmol/L H2O2 treatment alone for 4 h could induce intracellular mRNA expressions of catalase and Mn-SOD increase by 113.1% (p < 0.01) and 79.7% (p < 0.01), respectively. RECs were pre-treated with salidroside for 12 h and then co-incubated with H2O2 for another 4 h, intracellular mRNA expressions of catalase and Mn-SOD increased by 36.8% (p < 0.01) and 38.8% (p < 0.01), respectively, compared with H2O2 treatment alone. The expressions of catalase and Mn-SOD were further confirmed by Western blotting. As shown in Fig. (B) and (C), compared to the control groups, treatment with salidroside alone for 12 h could enhance intracellular catalase and Mn-SOD proteins by 63.7% (p < 0.01) and 59.7% (p < 0.05), respectively; H2O2 alone treatment for 4 h could elevate intracellular catalase and Mn-SOD proteins 107.8% (p < 0.01) and 142.5% (p < 0.01), respectively. Compared with H2O2 treatment alone, salidroside pre-treatment for 12 h and then co-incubation with H2O2 for 4 h could increase intracellular catalase and Mn-SOD proteins by 47.6% (p < 0.01) and 29.5% (p < 0.01), respectively.
Fig. 4. Activities and expression of catalase and Mn-SOD in primary-cultured RECs.
Notes: mRNA expression (A), protein levels (B and C), and activities (D) of catalase and Mn-SOD in RECs treated by either salidroside alone or H2O2 with or without salidroside (n = 4/group). (*p < 0.05, **p < 0.01).
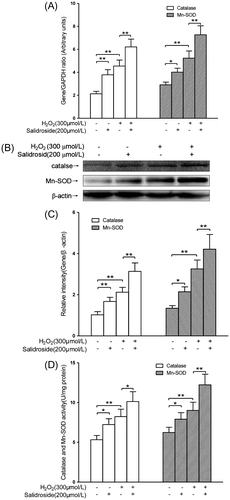
As shown in Fig. (D), compared with the control groups, salidroside alone treatment for 12 h enhanced intracellular catalase and Mn-SOD activities by 36.3% (p < 0.05) and 27.0% (p < 0.05), respectively; H2O2 alone treatment for 4 h increased intracellular catalase and Mn-SOD activities by 54.7% (p < 0.01) and 44.8% (p < 0.01), respectively. When RECs were pre-treated with salidroside for 12 h and then co-incubated with H2O2 for another 4 h, intracellular catalase and Mn-SOD activities increased by 22.7% (p < 0.01) and 35.6% (p < 0.01), respectively, compared with H2O2 alone treatment groups.
Salidroside increased Bcl2 expression and reduced Bax expression
Bcl2 could bind to and impair Bax and other proapoptotic proteins, while the ratio of Bcl2/Bax is an index to determine which cells undergo apoptosis.Citation20) As shown in Fig. , compared with the controls, the Bcl2 protein concentration significantly decreased by 44.6%, whereas Bax expression dramatically increased by 1.3-fold (both p < 0.01), respectively. This resulted into the Bcl2/Bax ratio significantly decreased by 74.4% (p < 0.01). Pre-treatment with salidroside for 12 h followed by co-incubation with H2O2 for another 4 h resulted in increased levels of Bcl2 expression but decreased levels of Bax expression. In addition, Bax/β-actin ratios decreased by 46.8% (p < 0.01), and Bcl2/β-actin and Bcl2/Bax ratios increased by 41.8% (p < 0.05) and 1.67-fold (p < 0.01), respectively, compared with H2O2 alone. However, incubation with salidroside alone for 12 h significantly increased Bcl2/β-actin ratios and Bcl2/Bax by 76.0% (p < 0.01) and 75.6% (p < 0.01), compared with controls.
Discussion
Oxidative stress is induced by excessive ROS production, which can be ameliorated by endogenous antioxidative defense mechanisms.Citation2,22–24) ROS can cause damages to the retinal endothelial cells.Citation10) H2O2, which is known as a major factor to induce oxidative damage, is transformed from a superoxide and leaks from the mitochondria.Citation22,25) The level of H2O2 and in mitochondria is elevated during the pathogenesis of diabetic retinopathy.Citation12–14) A few extracts from R. rosea are protective against tissue damages induced by H2O2.Citation6–9) Salidroside has a special neuroprotective effect of alleviate H2O2-induced apoptosis in rat hippocampal neurons by suppressing nitric oxide synthase activities and caspase-3.Citation26) In addition, Cai et al.Citation27) found that salidroside deceased apoptosis caused by H2O2 in PC12 cells through regulation of Bcl-2/Bax ratios and cytochrome c release. Castilho et al.Citation12) reported that H2O2 could induce a highly apoptosis ratios in retinal endothelial cell. However, the effects and the potential mechanisms of salidroside on oxidative injury in RECs are unclear.
In the present study, primary RECs were challenged with H2O2 to induce oxidative stress, which mimics the pathogenesis of oxidative stress in diabetic retinopathy by increasing in H2O2 concentration, lipid peroxidation level, cell death, and apoptosis. The exposure to 300 μmol/L H2O2 decreased RECs viability by 25% (Fig. (A)) and induced RECs apoptosis about 50% (Fig. (D)). The WST assay examines the succinate-tetrazolium reductase existing in viable cells, allowing a direct colorimetric measurement of cell viability and proliferation. The succinate–tetrazolium reductase is still active in the early apoptotic cells, so the cells display high viability and relatively low apoptotic ratio. The TUNEL method identifies apoptotic cells in situ using terminal deoxynucleotidyl transferase (TdT) to transfer biotin-dUTP to the free 3′-OH of cleaved DNA. The DNA damage even in early apoptotic cells can be monitored by the TUNEL assay, thus the ratio of apoptotic cell is higher than that measured in the WST assay. Salidroside (200 μmol/L) treatment showed obvious inhibitory effects on ROS generation, MDA production, and apoptosis of retinal endothelial cells induced by H2O2 stimulation.
Oxidative stress levels in tissues and cells are regulated by the balance between ROS generation and the enzymatic activity and non-enzymatic antioxidant defenses.Citation22,28,29) Mn-SOD is known as one of the most crucial intracellular antioxidant enzymes, while catalase is also the most significant enzyme to degrade exogenous H2O2.Citation28) In RECs, H2O2 stimulation increased mRNA expression, protein expression, and enzymatic activities of Mn-SOD and catalase, which was also reported similarly by Rohrdanz et al.Citation28) Moreover, salidroside treatment alone also obviously upregulated the intracellular mRNA expression, protein expression, and enzymatic activities of Mn-SOD and catalase, these results were comparable with those reported by Huang et al.Citation30) that treatment with R. rosea extracts upregulated the expression of MnSOD, Cu/Zn-SOD, and catalase in rat livers. Pre-treatment with salidroside, followed by co-incubation with H2O2, augmented the mRNA expression, protein expression, and enzymatic activities of MnSOD and catalase, in compared with those groups treated with H2O2 alone. Our results showed that salidroside was an adaptogen with antioxidative action. It strengthened the endogenous antioxidant defense system by decreasing the retinal endothelial oxidative stress through by increase of intracellular antioxidant enzymes.
To demonstrate further biochemical phenomenon sustaining the function of salidroside to protect RECs against H2O2-induced damage, we proved that salidroside was able to adjust intracellular Bcl2/Bax signaling pathwayCitation20), which was similarly found in retinal endothelial cells. We demonstrated that H2O2 exposure allowed the role to decease the protein expression level of Bcl2, while augmented the protein expression level of Bax in retinal endothelial cells compared with the control groups. Furthermore, the protein ratios of Bcl2 to Bax were obviously decreased, which was similarly to the increased apoptosis showed by TUNEL stain and of the expression of cleaved caspase-3. In RECs, salidroside co-incubation obviously decreased the H2O2-induced Bax expression and increased H2O2-suppressed Bcl2 and Bcl2/Bax ratios in the protein expression levels. These results were connected by a protection in apoptotic levels. The results indicated that salidroside significantly protected against damage induced by H2O2 in retinal endothelial cells, which showing an upregulation of Bcl2 and downregulation of Bax. Nevertheless, there is little evidence about the activation of salidroside on the Bcl2/Bax signaling pathway. It is crucial to identify the underlying mechanism of salidroside in the regulation of Bcl2 and Bax under the excessive oxidative stress.
In summary, our present study has shown that salidroside protects RECs against oxidative injury by suppressing the lipid peroxidation generation and cell apoptosis. The mechanisms could potentially involve the upregulation of the Bcl2/Bax signaling pathway and induction of endogenous antioxidant enzymes. This finding presents salidroside as an attractive agent with potential to attenuate retinopathic diseases.
Author contributions
Shi K, Zhang K, and Su Z conceived and designed the experiments. Shi K, Wang X, Zhu J, and Cao G performed the experiments. Shi K and Su Z analyzed the data. Shi K and Su Z wrote the paper.
Supplemental material
The supplemental material for this paper is available at http://dx.doi.org/10.1080/09168451.2015.1038212.
Supplemental Materials
Download TIFF Image (603.6 KB)Additional information
Funding
Notes
Abbreviation: H2O2, hydrogen peroxide; ROS, reactive oxygen species; RECs, retinal endothelial cells; WST, water soluble tetrazolium; TUNEL, TdT-mediated dUTP nick end labeling; MDA, malondialdehyde; Mn-SOD, Mn-superoxide dismutases; PECAM-1, Platelet/endothelial cell adhesion molecule-1; vWF, von Willebrand’s Factor; ECM, endothelial cell growth supplement; GAPDH, glyceraldehyde 3-phosphate dehydrogenase; RIPA, radio-immune precipitation assay; PVDF, polyvinylidene fluoride; H2DCFDA, 2′, 7′-dichlorofluorescein-diacetate.
References
- Lee J, Giordano S, Zhang J. Autophagy, mitochondria and oxidative stress: cross-talk and redox signalling. Biochem. J. 2012;441:523–540.10.1042/BJ20111451
- Hirooka Y, Sagara Y, Kishi T, Sunagawa K. Oxidative stress and central cardiovascular regulation. Circ. J. 2010;74:827–835.10.1253/circj.CJ-10-0153
- Apostolova N, Blas-Garcia A, Esplugues JV. Mitochondria sentencing about cellular life and death: a matter of oxidative stress. Curr. Pharm. Des. 2011;17:4047–4060.10.2174/138161211798764924
- Zhu YZ, Huang SH, Tan BK, Sun J, Whiteman M, Zhu YC. Antioxidants in Chinese herbal medicines: a biochemical perspective. Nat. Prod. Rep. 2004;21:478–489.10.1039/b304821g
- Zhang J, Liu A, Hou R, Zhang J, Jia X, Jiang W. Salidroside protects cardiomyocyte against hypoxia-induced death: a HIF-1α-activated and VEGF-mediated pathway. Eur. J. Pharmacol. 2009;607:6–14.10.1016/j.ejphar.2009.01.046
- Kelly GS. Rhodiola rosea: a possible plant adaptogen. Altern. Med. Rev. 2001;6:293–302.
- Zhu Y, Shi YP, Wu D, Ji YJ, Wang X, Chen HL, Wu SS, Huang DJ, Jiang W. Salidroside protects against hydrogen peroxide-induced injury in cardiac H9c2 cells via PI3K-Akt dependent pathway. DNA Cell Biol. 2011;30:809–819.10.1089/dna.2010.1183
- Qian EW, Ge DT, Kong SK. Salidroside protects human erythrocytes against hydrogen peroxide-induced apoptosis. J. Nat. Prod. 2012;75:531–537.10.1021/np200555s
- Xu MC, Shi HM, Wang H, Gao XF. Salidroside protects against hydrogen peroxide-induced injury in HUVECs via the regulation of REDD1 and mTOR activation. Mol. Med. Rep. 2013;8:147–153.
- Busik JV, Mohr S, Grant MB. Hyperglycemia-induced reactive oxygen species toxicity to endothelial cells is dependent on paracrine mediators. Diabetes. 2008;57:1952–1965.10.2337/db07-1520
- Zhang L, Chen B, Tang L. Metabolic memory: mechanisms and implications for diabetic retinopathy. Diabetes Res. Clin. Pract. 2012;96:286–293.10.1016/j.diabres.2011.12.006
- Castilho ÁF, Aveleira CA, Leal EC, Simões NF, Fernandes CR, Meirinhos RI, Baptista FI, Ambrósio AF. Heme oxygenase-1 protects retinal endothelial cells against high glucose- and oxidative/nitrosative stress-induced toxicity. PLoS ONE. 2012;7:e42428.10.1371/journal.pone.0042428
- Kowluru RA. Effect of advanced glycation end products on accelerated apoptosis of retinal capillary cells under in vitro conditions. Life Sci. 2005;76:1051–1060.10.1016/j.lfs.2004.10.017
- Grammas P, Riden M. Retinal endothelial cells are more susceptible to oxidative stress and increased permeability than brain-derived endothelial cells. Microvasc. Res. 2003;65:18–23.10.1016/S0026-2862(02)00016-X
- Su X, Sorenson CM, Sheibani N. Isolation and characterization of murine retinal endothelial cells. Mol. Vis. 2003;9:171–178.
- Matsubara TA, Murata TA, Wu GS, Barron EA, Rao NA. Isolation and culture of rat retinal microvessel endothelial cells using magnetic beads coated with antibodies to PECAM-1. Curr. Eye Res. 2000;20:1–7.10.1076/0271-3683(200001)2011-HFT001
- Kuang J, Hou X, Zhang J, Chen Y, Su Z. Identification of insulin as a novel retinoic acid receptor-related orphan receptor α target gene. FEBS Lett. 2014;588:1071–1079.10.1016/j.febslet.2014.02.029
- Su Z, Leduc MS, Korstanje R, Paigen B. Untangling HDL quantitative trait loci on mouse chromosome 5 and identifying Scarb1 and Acads as the underlying genes. J. Lipid Res. 2010;51:2706–2713.10.1194/jlr.M008110
- Babich H, Borenfreund E. Cytotoxicity of T-2 toxin and its metabolites determined with the neutral red cell viability assay. Appl. Environ. Microbiol. 1991;57:2101–2103.
- Basu A, Haldar S. The relationship between BcI2, Bax and p53: consequences for cell cycle progression and cell death. Mol. Hum. Reprod. 1998;4:1099–1109.10.1093/molehr/4.12.1099
- Del Rio D, Stewart AJ, Pellegrini N. A review of recent studies on malondialdehyde as toxic molecule and biological marker of oxidative stress. Nutr. Metab. Cardiovasc. Dis. 2005;15:316–328.10.1016/j.numecd.2005.05.003
- Martindale JL, Holbrook NJ. Cellular response to oxidative stress: signaling for suicide and survival. J. Cell. Physiol. 2002;192:1–15.10.1002/(ISSN)1097-4652
- Sachidanandam K, Fagan SC, Ergul A. Oxidative stress and cardiovascular disease: antioxidants and unresolved issues. Cardiovasc. Drug Rev. 2005;23:115–132.
- Violi F, Cangemi R, Brunelli A. Oxidative stress, antioxidants, and cardiovascular disease. Arterioscler. Thromb. Vasc. Biol. 2005;25: e37; author reply e37.
- Miwa S, St-Pierre J, Partridge L, Brand MD. Superoxide and hydrogen peroxide production by Drosophila mitochondria. Free Radic. Biol. Med. 2003;35:938–948.10.1016/S0891-5849(03)00464-7
- Chen X, Zhang Q, Cheng Q, Ding F. Protective effect of salidroside against H2O2-induced cell apoptosis in primary culture of rat hippocampal neurons. Mol. Cell. Biochem. 2009;332:85–93.10.1007/s11010-009-0177-3
- Cai L, Wang H, Li Q, Qian Y, Yao W. Salidroside inhibits H2O2-induced apoptosis in PC 12 cells by preventing cytochrome c release and inactivating of caspase cascade. Acta Biochim. Biophys. Sin. 2008;40:796–802.10.1093/abbs/40.9.796
- Röhrdanz E, Kahl R. Alterations of antioxidant enzyme expression in response to hydrogen peroxide. Free Radic. Biol. Med. 1998;24:27–38.10.1016/S0891-5849(97)00159-7
- Hamilton CA, Miller WH, Al-Benna S, Brosnan MJ, Drummond RD, McBride MW, Dominiczak AF. Strategies to reduce oxidative stress in cardiovascular disease. Clin. Sci. 2004;106:219–234.10.1042/CS20030379
- Huang SC, Lee FT, Kuo TY, Yang JH, Chien CT. Attenuation of long-term Rhodiola rosea supplementation on exhaustive swimming-evoked oxidative stress in the rat. Chin. J. Physiol. 2009;52:316–324.10.4077/CJP.2009.AMH029