Abstract
Matrix metalloproteinases (MMPs) are zinc-dependent endopeptidases that degrade many extracellular matrix components and that have been implicated in the pathogenesis of various human diseases including cancer metastasis. Here, we screened MMP-9 inhibitors using photo-cross-linked chemical arrays, which can detect small-molecule ligand–protein interactions on a chip in a high-throughput manner. The array slides were probed sequentially with His-MMP-9, anti-His antibody, and a Cy5-labeled secondary antibody and then scanned with a microarray scanner. We obtained 27 hits among 24,275 compounds from the NPDepo library; 2 of the identified compounds (isoxazole compound 1 and naphthofluorescein) inhibited MMP-9 enzyme activity in vitro. We further explored 17 analogs of 1 and found that compound 18 had the strongest inhibitory activity. Compound 18 also inhibited other MMPs, including MMP-2, MMP-12, and MMP-13 and significantly inhibited cell migration in human fibrosarcoma HT1080 cells. These results suggest that 18 is a broad-spectrum MMP inhibitor.
Graphical abstract
We identify novel MMP inhibitors using chemical arrays, which detect small molecule–protein interactions on a chip in a high-throughput manner.
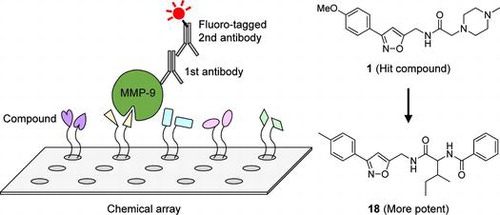
Matrix metalloproteinases (MMPs) are a family of zinc-dependent endopeptidases that degrade multiple components of the extracellular matrix (ECM), including collagens, fibronectin, laminin, proteoglycans, and elastin.Citation1,2) MMPs play a role in many physiological processes, such as ECM homeostasis, tissue remodeling, and organogenesis. However, MMPs can also participate in a wide range of pathological processes, such as cancer metastasis, rheumatoid arthritis, and chronic obstructive pulmonary disease.Citation3−8)
In humans, 23 MMP proteins have been identified, which can be roughly classified based on substrate specificity into collagenases, gelatinases, stromelysins, matrilysins, membrane-type MMPs, and others. MMPs all possess an N-terminal signal peptide that targets them to the secretory pathway, a propeptide domain for enzyme latency, and a catalytic domain with a highly conserved zinc-binding site. The propeptide domain contains the conserved “cysteine switch” motif PRCGXPD at the N-terminus, which binds to the catalytic Zn2+ ion to block its activity. Most MMPs also possess a C-terminal hemopexin domain, which is coupled to the catalytic domain by a flexible hinge region and which is an important mediator of protein–protein interactions. MMPs are secreted as inactive zymogens, and they are activated via removal of the propeptide domain from the active site, for example, by proteolysis of other MMPs or furin-like serine proteases.Citation1,2)
Many small-molecule MMP inhibitors (MMPIs) have been designed to target MMPs in cancer.Citation9−11) The first generation of MMPIs were peptidomimetics, which mimic the structure of collagen. They act as competitive inhibitors and chelate the zinc ion present at the active site. To improve specificity and oral bioavailability, non-peptidomimetics were synthesized, based on the 3D conformation of the MMP active site. Other MMPIs include tetracycline derivatives and novel mechanism-based MMPIs such as SB-3CT (2-((4-phenoxyphenylsulfonyl)methyl)thiirane). After the failure of early broad-spectrum MMPIs in the treatment of cancer in clinical trials, drug development strategies focused on more specific MMPIs. In addition, recent studies have shown that several MMPs have non-proteolytic functions, for example, through the hemopexin domain of MMPs.Citation12−15) Furthermore, several MMPs have been shown to provide tumor-suppressing functions in different stages of cancer progression.Citation16) Thus, much work remains for the elucidation of each MMP function and the development of new MMPIs.
Small-molecule microarrays represent a powerful new platform for facilitating target-based screens and reverse chemical genetics studies.Citation17) Thousands of small molecules are arrayed on a glass slide and used to screen for protein–small molecule interactions. This small-molecule microarray format has great advantages of miniaturization, throughput, and generality with regard to screening protocols. Typically, solid surfaces are derivatized with certain functional groups, and small molecules with a reactive functional group readily attach to the surface upon printing via a selective-coupling reaction. For example, the first small-molecule microarrays were prepared using the Michael addition reaction, involving small molecules containing free thiols printed on slides coated with vinyl sulfone or maleimide groups.Citation18) However, such selective-coupling approaches may limit the number of analyzable compounds and may mask the potential protein binding site.
We developed a rapid and universal coupling method that enables the introduction of a variety of small molecules to a solid support using a photoaffinity reaction.Citation19−21) In contrast to conventional methods, this method immobilizes a small molecule on a solid support in a functional group-independent (or non-selective) manner. Therefore, a wide variety of small molecules can be immobilized without chemical modification because chemical immobilization is achieved by UV irradiation. This method also allows small molecules to bind to all potential binding proteins, because the immobilized small molecules display virtually all of their surfaces. The utility of this photo-cross-linked small-molecule microarray has been proven in the discovery of ligands of many type of targets, including prokaryotic proteins,Citation22) viral proteins,Citation23,24) and disease-related mammalian proteins.Citation25−29)
In this study, we describe the identification of MMPIs using chemical arrays. The chemical array-based screen developed here, followed by an enzyme assay, identified isoxazole compound 1 as an MMP-9 inhibitor. By exploring analogs of 1, we identified compound 18, which was approximately 60-fold stronger than 1. Compound 18 inhibited various MMPs, including MMP-2, MMP-9, MMP-12, and MMP-13 and inhibited cell migration in human fibrosarcoma HT1080 cells, suggesting that 18 is a broad-spectrum MMPI.
Materials and methods
Materials
Compound 1 and naphthofluorescein were obtained from the RIKEN Natural Products Depository (NPDepo, Saitama, Japan).Citation30−32) Analogs of 1 were purchased from Namiki Shoji (Tokyo, Japan). All compounds were dissolved in dimethylsulfoxide (DMSO) as stock solutions, which were stored at −20 °C. His-Myc-tagged human recombinant MMP-9 was purified as previously described.Citation33)
Chemical array screening
The chemical arrays were prepared according to our previous reports.Citation20,25) Solutions of the 24,275 compounds (2.5 mg mL−1 in DMSO) from NPDepo were arrayed onto 9 separate photoaffinity linker-coated glass slides using a chemical arrayer developed at RIKEN. The slides were incubated with TBS-T [10 mM Tris–HCl (pH 8.0), 150 mM NaCl, 0.05% Tween-20] containing 1% skim milk for 1 h at room temperature. Then, the slides were probed at 30 °C for 1 h with 1 μM purified His-Myc-tagged human recombinant MMP-9 in TBS-T containing 1% skim milk. After washing with TBS-T, the probed slides were incubated with anti-His antibody (mouse IgG, 1/100 diluted, GE Healthcare) in TBS-T containing 1% skim milk at 30 °C for 1 h. This incubation was followed by another washing step and incubation with a second antibody (goat anti-mouse IgG, Cy5 conjugate, 50 μg mL−1, Millipore) at 30 °C for 1 h. After the final wash step, slides were scanned with a GenePix microarray scanner (Molecular Devices) using the Cy5 channel (an excitation wavelength of 635 nm and an emission wavelength of 675 nm). The fluorescence signals were quantified using the GenePixPro 6.1 software (Molecular Devices) with local background subtraction. We used data from slides treated with antibodies alone as a reference. The images from 2 slides treated with and without His-Myc-tagged MMP-9 were tinted red and green, respectively, using Photoshop 5.5 software (Adobe Photoshop) and were merged into a composite image.
MMP enzyme assays
MMP enzyme assays were performed according to the manufacturer’s instructions (R&D Systems). Activated human recombinant MMP-1 (R&D Systems), MMP-2 (R&D Systems), MMP-7 (R&D Systems), MMP-9 (R&D Systems), MMP-9 with a truncated C-terminal hemopexin domain (MMP-9/ΔHemopexin, Calbiochem), MMP-12 (R&D Systems), or MMP-13 (R&D Systems) was preincubated with the compounds for 30 min at 37 °C, and then, the fluorogenic peptide substrate was added (Mca-PLGL-Dpa-AR-NH2, R&D Systems). Fluorescence emission at 405 nm with excitation at 320 nm was measured in kinetic mode for 30 min at 37 °C, using a fluorescent microplate reader (SpectraMax M2e, Molecular Devices).
Cell culture and cell growth assays
The human fibrosarcoma cell line HT1080 (RIKEN Cell Bank) was cultured in D-MEM (Invitrogen), supplemented with 10% fetal calf serum (Nichirei Biosciences Inc., Tokyo, Japan), 50 U mL−1 penicillin (Invitrogen), and 50 μg mL−1 streptomycin (Invitrogen), at 37 °C in a humidified atmosphere containing 5% CO2. Cell growth was measured using Cell Count Reagent SF (WST-8 solution, Nacalai Tesque, Kyoto, Japan) as described previously.Citation34)
Cell migration assay
Cell migration was determined using a wound-healing scratch assay. HT1080 cells were seeded at high densities (1 × 105 cells/well) into 24-well culture plates and grown overnight. After replacement of the culture medium without serum for 2 h, cells were scratched with a pipette tip to generate a scratch wound and then incubated with or without compound 18 for 24 h. The healing rate was quantified using image analysis of post-culture measurements of the gap size (Adobe Photoshop). Statistical analysis was performed using one-way ANOVA followed by Bonferroni’s post hoc test. A p-value <0.05 was considered statistically significant.
Results
Chemical array-based screening for MMP-9 inhibitors
To identify novel MMP-9 inhibitors, we first performed chemical array-based screening to obtain small molecules that interacted with MMP-9. The chemical arrays of immobilized NPDepo library compounds were probed sequentially with or without purified His-Myc-tagged human recombinant MMP-9, anti-His antibody, and a Cy5-labeled secondary antibody and then scanned with a microarray scanner (Fig. (A)). The fluorescence signals from two slides treated with and without His-Myc-tagged MMP-9 were quantified. In this first screening, 27 hits were obtained among the 24,275 compounds (Fig. (B)).
Fig. 1. Chemical array-based screening for MMP-9 inhibitors.
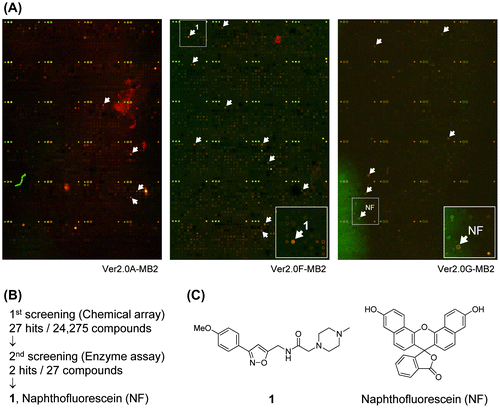
We next performed an in vitro enzyme assay to examine the inhibitory activity of these 27 compounds on MMP-9. In this second screening, two of the compounds (isoxazole compound 1 and naphthofluorescein) inhibited MMP-9 enzyme activity (Fig. (B) and (C)). The IC50 values of 1 and naphthofluorescein against MMP-9 were 233 (Table ) and 15 μM, respectively.
Table 1. Inhibition of MMP-9 by analogs of 1.
Inhibition of MMP-9 by analogs of 1
To obtain more potent inhibitors of MMP-9, we further explored analogs of 1. An in vitro MMP-9 enzyme assay showed that compound 18 had the strongest inhibitory activity, with an IC50 value of 3.8 μM; it was approximately 60-fold stronger than 1 (Table ). Overall, analogs with an amide (12, 16, and 18), a sulfonamide (13 and 17), or a carbamate (14) showed increased potency of enzyme inhibition.
Compound 18 is a broad-spectrum MMPI
We next examined the selectivity and potency of 18 on various MMPs. As shown in Table , 18 inhibited the enzyme activities of MMP-2, MMP-12, and MMP-13, as well as MMP-9 and MMP-9/ΔHemopexin, with almost identical potency. Compound 18 did not inhibit the enzyme activities of MMP-1 or MMP-7 up to 100 μM (Table ). These results suggest that 18 is a broad-spectrum MMPI.
Table 2. Inhibition of various MMPs by 18.
Compound 18 inhibits cell migration in HT1080 cells
Some MMPIs are known to inhibit migration of human fibrosarcoma HT1080 cells.Citation35,36) To examine the effect of 18 on cells, we monitored migration of HT1080 cells treated with 18. As shown in Fig. , 18 significantly inhibited migration in HT1080 cells with almost no cell toxicity.
Fig. 2. Compound 18 inhibits cell migration in HT1080 cells.
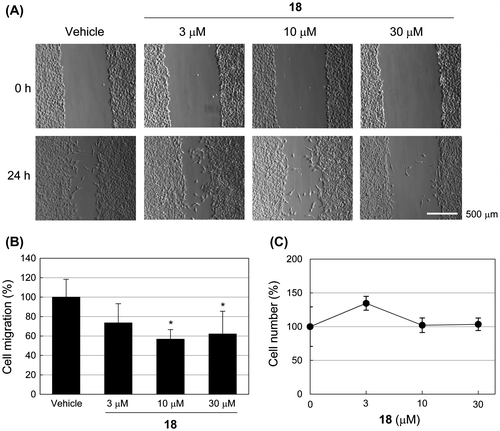
Discussion
Using a chemical array-based screen for MMP-9 inhibitors, we identified a compound (18) that is a broad-spectrum MMPI, as evidenced by its inhibition of various MMPs in vitro (Table ) and the inhibition of HT1080 cell migration (Fig. ). The catalytic Zn2+ ion at the active site of MMPs is surrounded by subsite pockets designated as S1, S2, S3, S1′, S2′, and S3′. Of these, the S1′ pocket provides the basis of selectivity for many MMPIs.Citation11) MMP-3, -11, -12, and -13 have deep S1′ pockets; MMP-2, -8, and -9 have intermediate S1′ pockets, but MMP-1 and -7 have shallow S1′ pockets, which are occluded by Arg214 and Tyr214, respectively. Compound 18 inhibited the enzyme activities of MMP-2, -9, -12, and -13, but not MMP-1 and -7, suggesting that this compound might target near the S1′ pocket of the active site.
We also found that naphthofluorescein inhibits the enzyme activity of MMP-9 with an IC50 value of 15 μM (Fig. ). Naphthofluorescein is known as a fluorescent indicator or a pH indicator and has several biological activities, such as inhibition of furin.Citation37) Therefore, derivatization would be required to use naphthofluorescein as a specific MMPI.
Recently, small-molecule inhibitors targeting the hemopexin domain of MMPs have been reported.Citation38,39) For example, Dufour et al. identified small-molecule compounds that specifically bind to the hemopexin domain of MMP-9 using an in silico docking approach.Citation38) These compounds abrogated MMP-9 homodimerization without affecting its catalytic activity and inhibited MMP-9-mediated cell migration. Chemical array screening for MMP-9 ligands identified 27 candidate compounds (Fig. ). Of these 25 compounds (excluding 1 and naphthofluorescein), some scarcely affected the catalytic activity of MMP-9 (data not shown). Although false positives are possible, such compounds might bind a domain other than the catalytic domain, for example, the hemopexin domain, and might affect MMP-9 function. In this regard, further analyses, such as evaluation of inhibition of MMP-9 dimerization, would be needed to characterize the effect of the compounds.
Chemical array platforms are based on interactions between proteins and small molecules and are adaptable to any type of protein, including non-enzymes and artificial proteins. Therefore, it would be possible to identify small-molecule compounds specifically targeting each MMP domain, using various deletion mutant proteins. Further, chemical array screens for a series of MMPs would allow identification of both broad-spectrum and specific MMPIs in a high-throughput manner.
In summary, we have demonstrated that 18 is a broad-spectrum MMPI using a chemical array-based screen followed by a secondary assay and derivatization. Our study has also shown that our photo-cross-linked chemical arrays are useful for high-throughput drug screening and reverse chemical genetics studies.
Authors contribution
M.K., Y.Y., N.T., and H.O. designed the research plan; M.K., Y.F., and Y.Y. performed the biochemical and cellular experiments and analyzed data; Y.K., K.H., and T.S. performed the chemical array screening and analyzed data; M.K., Y.K., and H.O. wrote the manuscript with assistance from all authors; and N.T. and H.O. supervised the research.
Acknowledgments
We wish to thank Y. Oku (RIKEN), M. Uchida (RIKEN), H. Aono (RIKEN), C. Gao (Osaka University), M. M. Khan (EPFL), T. Nogawa (RIKEN), M. Muroi (RIKEN), N. Watanabe (RIKEN), and T. Saito (RIKEN) for their help with the experiments. This work was supported in part by the Program for Promotion of Fundamental Studies in Health Sciences of the National Institute of Biomedical Innovation (NIBIO) and Grant-in-Aid for Scientific Research (KAKENHI).
Disclosure statement
The authors declare no conflicts of interest.
References
- Sternlicht MD, Werb Z. How matrix metalloproteinases regulate cell behavior. Annu. Rev. Cell Dev. Biol. 2001;17:463–516.10.1146/annurev.cellbio.17.1.463
- Nagase H, Visse R, Murphy G. Structure and function of matrix metalloproteinases and TIMPs. Cardiovasc. Res. 2006;69:562–573.10.1016/j.cardiores.2005.12.002
- Page-McCaw A, Ewald AJ, Werb Z. Matrix metalloproteinases and the regulation of tissue remodelling. Nat. Rev. Mol. Cell Biol. 2007;8:221–233.10.1038/nrm2125
- Kessenbrock K, Plaks V, Werb Z. Matrix metalloproteinases: regulators of the tumor microenvironment. Cell. 2010;141:52–67.10.1016/j.cell.2010.03.015
- Vandenbroucke RE, Dejonckheere E, Libert C. A therapeutic role for matrix metalloproteinase inhibitors in lung diseases? Eur. Respir. J. 2011;38:1200–1214.10.1183/09031936.00027411
- Szarvas T, vom Dorp F, Ergün S, Rübben H. Matrix metalloproteinases and their clinical relevance in urinary bladder cancer. Nat. Rev. Urol. 2011;8:241–254.10.1038/nrurol.2011.44
- Huntley GW. Synaptic circuit remodelling by matrix metalloproteinases in health and disease. Nat. Rev. Neurosci. 2012;13:743–757.10.1038/nrn3320
- Khokha R, Murthy A, Weiss A. Metalloproteinases and their natural inhibitors in inflammation and immunity. Nat. Rev. Immunol. 2013;13:649–665.10.1038/nri3499
- Sang QX, Jin Y, Newcomer RG, Monroe SC, Fang X, Hurst DR, Lee S, Cao Q, Schwartz MA. Matrix metalloproteinase inhibitors as prospective agents for the prevention and treatment of cardiovascular and neoplastic diseases. Curr. Top. Med. Chem. 2006;6:289–316.10.2174/156802606776287045
- Hu J, Van den Steen PE, Sang QX, Opdenakker G. Matrix metalloproteinase inhibitors as therapy for inflammatory and vascular diseases. Nat. Rev. Drug Discovery. 2007;6:480–498.10.1038/nrd2308
- Devel L, Czarny B, Beau F, Georgiadis D, Stura E, Dive V. Third generation of matrix metalloprotease inhibitors: gain in selectivity by targeting the depth of the S1’ cavity. Biochimie. 2010;92:1501–1508.10.1016/j.biochi.2010.07.017
- Sakamoto T, Seiki M. Cytoplasmic tail of MT1-MMP regulates macrophage motility independently from its protease activity. Genes Cells. 2009;14:617–626.10.1111/gtc.2009.14.issue-5
- Houghton AM, Hartzell WO, Robbins CS, Gomis-Rüth FX, Shapiro SD. Macrophage elastase kills bacteria within murine macrophages. Nature. 2009;460:637–641.
- Glasheen BM, Kabra AT, Page-McCaw A. Distinct functions for the catalytic and hemopexin domains of a Drosophila matrix metalloproteinase. Proc. Natl. Acad. Sci. U.S.A. 2009;106:2659–2664.10.1073/pnas.0804171106
- Redondo-Muñoz J, Ugarte-Berzal E, Terol MJ, Van den Steen, PE, Hernández del Cerro M, Roderfeld M, Roeb E, Opdenakker G, García-Marco JA, García-Pardo A. Matrix metalloproteinase-9 promotes chronic lymphocytic leukemia B cell survival through its hemopexin domain. Cancer Cell. 2010;17:160–172.10.1016/j.ccr.2009.12.044
- López-Otín C, Matrisian LM. Emerging roles of proteases in tumour suppression. Nat. Rev. Cancer. 2007;7:800–808.10.1038/nrc2228
- Vegas A, Fuller J, Koehler A. Small-molecule microarrays as tools in ligand discovery. Chem. Soc. Rev. 2008;37:1385–1394.10.1039/b703568n
- MacBeath G, Koehler A, Schreiber S. Printing small molecules as microarrays and detecting protein−ligand interactions en masse. J. Am. Chem. Soc. 1999;121:7967–7968.10.1021/ja991083q
- Kanoh N, Kumashiro S, Simizu S, Kondoh Y, Hatakeyama S, Tashiro H, Osada H. Immobilization of natural products on glass slides by using a photoaffinity reaction and the detection of protein–small-molecule interactions. Angew. Chem. Int. Ed. Engl. 2003;42:5584–5587.10.1002/(ISSN)1521-3773
- Kanoh N, Asami A, Kawatani M, Honda K, Kumashiro S, Takayama H, Simizu S, Amemiya T, Kondoh Y, Hatakeyama S, Tsuganezawa K, Utata R, Tanaka A, Yokoyama S, Tashiro H, Osada H. Photo-cross-linked small-molecule microarrays as chemical genomic tools for dissecting protein–ligand interactions. Chem. Asian J. 2006;1:789–797.10.1002/(ISSN)1861-471X
- Kawatani M, Osada H. Affinity-based target identification for bioactive small molecules. Med. Chem. Commun. 2014;5:277–287.10.1039/c3md00276d
- Minagawa S, Kondoh Y, Sueoka K, Osada H, Nakamoto H. Cyclic lipopeptide antibiotics bind to the N-terminal domain of the prokaryotic Hsp90 to inhibit the chaperone activity. Biochem. J. 2011;435:237–246.10.1042/BJ20100743
- Hagiwara K, Kondoh Y, Ueda A, Yamada K, Goto H, Watanabe T, Nakata T, Osada H, Aida Y. Discovery of novel antiviral agents directed against the influenza A virus nucleoprotein using photo-cross-linked chemical arrays. Biochem. Biophys. Res. Commun. 2010;394:721–727.10.1016/j.bbrc.2010.03.058
- Hagiwara K, Murakami T, Xue G, Shimizu Y, Takeda E, Hashimoto Y, Honda K, Kondoh Y, Osada H, Tsunetsugu-Yokota Y, Aida Y. Identification of a novel Vpr-binding compound that inhibits HIV-1 multiplication in macrophages by chemical array. Biochem. Biophys. Res. Commun. 2010;403:40–45.10.1016/j.bbrc.2010.10.107
- Miyazaki I, Simizu S, Ichimiya H, Kawatani M, Osada H. Robust and systematic drug screening method using chemical arrays and the protein library: identification of novel inhibitors of carbonic anhydrase II. Biosci. Biotechnol. Biochem. 2008;72:2739–2749.10.1271/bbb.80383
- Miyazaki I, Simizu S, Okumura H, Takagi S, Osada H. A small-molecule inhibitor shows that pirin regulates migration of melanoma cells. Nat. Chem. Biol. 2010;6:667–673.10.1038/nchembio.423
- Burger M, Zimmermann TJ, Kondoh Y, Stege P, Watanabe N, Osada H, Waldmann H, Vetter IR. Crystal structure of the predicted phospholipase LYPLAL1 reveals unexpected functional plasticity despite close relationship to acyl protein thioesterases. J. Lipid Res. 2012;53:43–50.10.1194/jlr.M019851
- Zimmermann TJ, Bürger M, Tashiro E, Kondoh Y, Martinez NE, Görmer K, Rosin-Steiner S, Shimizu T, Ozaki S, Mikoshiba K, Watanabe N, Hall D, Vetter IR, Osada H, Hedberg C, Waldmann H. Boron-based inhibitors of acyl protein thioesterases 1 and 2. ChemBioChem. 2013;14:115–122.10.1002/cbic.201200571
- Noguchi T, Oishi S, Honda K, Kondoh Y, Saito T, Kubo T, Kaneda M, Ohno H, Osada H, Fujii N. Affinity-based screening of MDM2/MDMX–p53 interaction inhibitors by chemical array: identification of novel peptidic inhibitors. Bioorg. Med. Chem. Lett. 2013;23:3802–3805.10.1016/j.bmcl.2013.04.094
- Osada H. Introduction of new tools for chemical biology research on microbial metabolites. Biosci. Biotechnol. Biochem. 2010;74:1135–1140.10.1271/bbb.100061
- Osada H, Nogawa T. Systematic isolation of microbial metabolites for natural products depository (NPDepo). Pure Appl. Chem. 2012;84:1407–1420.
- Kato N, Takahashi S, Nogawa T, Saito T, Osada H. Construction of a microbial natural product library for chemical biology studies. Curr. Opin. Chem. Biol. 2012;16:101–108.10.1016/j.cbpa.2012.02.016
- Khan M, Simizu S, Suzuki T, Masuda A, Kawatani M, Muroi M, Dohmae N, Osada H. Protein disulfide isomerase-mediated disulfide bonds regulate the gelatinolytic activity and secretion of matrix metalloproteinase-9. Exp. Cell Res. 2012;318:904–914.10.1016/j.yexcr.2012.02.021
- Kawatani M, Takayama H, Muroi M, Kimura S, Maekawa T, Osada H. Identification of a small-molecule inhibitor of DNA topoisomerase II by proteomic profiling. Chem. Biol. 2011;18:743–751.10.1016/j.chembiol.2011.03.012
- Ikejiri M, Bernardo MM, Bonfil RD, Toth M, Chang M, Fridman R, Mobashery S. Potent mechanism-based inhibitors for matrix metalloproteinases. J. Biol. Chem. 2005;280:33992–34002.10.1074/jbc.M504303200
- Sounni NE, Rozanov DV, Remacle AG, Golubkov VS, Noel A, Strongin AY. Timp-2 binding with cellular MT1-MMP stimulates invasion-promoting MEK/ERK signaling in cancer cells. Int. J. Cancer. 2010;126:1067–1078.
- Coppola JM, Bhojani MS, Ross BD, Rehemtulla A. A small-molecule furin inhibitor inhibits cancer cell motility and invasiveness. Neoplasia. 2008;10:363–370.10.1593/neo.08166
- Dufour A, Sampson NS, Li J, Kuscu C, Rizzo RC, DeLeon JL, Zhi J, Jaber N, Liu E, Zucker S, Cao J. Small-molecule anticancer compounds selectively target the hemopexin domain of matrix metalloproteinase-9. Cancer Res. 2011;71:4977–4988.10.1158/0008-5472.CAN-10-4552
- Remacle AG, Golubkov VS, Shiryaev SA, Dahl R, Stebbins JL, Chernov AV, Cheltsov AV, Pellecchia M, Strongin AY. Novel MT1-MMP small-molecule inhibitors based on insights into hemopexin domain function in tumor growth. Cancer Res. 2012;72:2339–2349.10.1158/0008-5472.CAN-11-4149