Abstract
Stabilization of l-ascorbic acid (l-AA) is a challenging task for food and pharmaceutical industries. The study was conducted to prepare monodisperse aqueous microspheres containing enhanced concentrations of l-AA by using microchannel emulsification (MCE). The asymmetric straight-through microchannel (MC) array used here constitutes 11 × 104 μm microslots connected to a 10 μm circular microholes. 5–30% (w/w) l-AA was added to a Milli-Q water solution containing 2% (w/w) sodium alginate and 1% (w/w) magnesium sulfate, while the continuous phase constitutes 5% (w/w) tetraglycerol condensed ricinoleate in water-saturated decane. Monodisperse aqueous microspheres with average diameters (dav) of 18.7–20.7 μm and coefficients of variation (CVs) below 6% were successfully prepared via MCE regardless of the l-AA concentrations applied. The collected microspheres were physically stable in terms of their dav and CV for >10 days of storage at 40°C. The aqueous microspheres exhibited l-AA encapsulation efficiency exceeding 70% during the storage.
Straight-through microchannel emulsification has ability to produce monodisperse emulsions. l-AA is encapsulated in aqueous microspheres and has encapsulation efficiency of over 70% after 10 days of storage at 40oC.
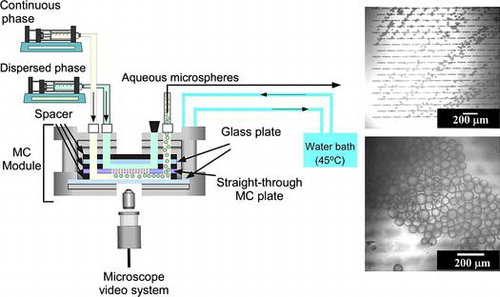
There are increasing demands for products which are expected to optimize health and target-delivery of supplements to the optimum absorption sites in the gastrointestinal tract. During last two decades, microspheres have been widely used in food, pharmaceutical and chemical industries.Citation1–3) Monodisperse microspheres have numerous advantages such as efficient drug delivery, higher bioavailability, high dispersibility, and fast permeability.Citation4–6) Microspheres are generated by different methods including ionic gelation,Citation7) cross-linked emulsions,Citation8) sieve methods,Citation9) bio-electro spraying,Citation10) and numerous spray drying techniques.Citation11) All of these methods have potential advantages such as better particle size controllability, better particle morphology and better penetration at target areas.
Microparticle technology has potential applications in the controlled delivery of bioactives such as vitamins, probiotics, small bioactive peptides, and antioxidants.Citation12) For instance, Ellis and JacquierCitation13) formulated food-grade κ-carrageenan microspheres with a broad range of functionalities such as nutraceutical delivery systems or fat replacers. Prasertmanakit et al.Citation14) prepared ethyl cellulose microcapsules for protection and controlled release of folic acid in foods and supplements. Duclairoir et al.Citation15) formulated gliadin nanoparticles as efficient drug carriers for α-tocopherol or vitamin E.
l-Ascorbic acid (l-AA) is a water soluble vitamin and regarded as dietary intake of vitamin C.Citation16) l-AA is present in many leafy vegetables and fruits, noticeably in guava, orange, apple, strawberry, kiwi, capsicum, pawpaw, and cauliflower.Citation17) The importance of vitamin C is seen clearly in scurvy, a life-threatening vitamin C deficiency disease that results in loss of energy, depression, mood disorders, poor wound healing, and connective tissue disorders, culminating in death.Citation18) Vitamin C plays an important role in formation of collagen (in bones, teeth and cartilage) and activation of various enzymes related to nervous system, and detoxification of drugs in liver. With a structure similar to carbohydrates, vitamin C can uniquely undergo reversible oxidation reactions.Citation19) Additionally, the chemical properties of vitamin C allow it to react with a wide variety of potentially harmful reactive oxygen species. Vitamin C is stable in powder form, while its stability decreases in aqueous formulations. Environmental factors that affect the stability include temperature, pH, oxygen, metal ion, UV, and X-ray.Citation20,21)
Microchannel emulsification (MCE) is a progressive method that enables preparing monodisperse droplets by spontaneous transformation of oil-water interface specifically driven by interfacial tension on a micron scaleCitation22) and comprehensively reviewed by Vladisavljevic et al.Citation23) Similarly, this emulsification technique allows integration of hundreds of thousands of droplet generation units on a single silicon and on the other hand stainless steel chips currently contain fewer number of droplet generating units.Citation24,25) Kawakatsu et al.Citation26) introduced MCE and this technology enables the production of different emulsions with diameter ranged from 1 to 500 μm. The fabricated microchannel (MC) arrays are classified as grooved MC arrays consisting of uniform microgrooves with slit-like terraces outside them and straight-through MC arrays having two-dimensionally positioned, symmetric or asymmetric micro-through-holes.Citation23) These straight-through MC arrays enable the mass production of monodisperse droplets, with precise control on processing parameters.
MCE has been used to produce monodisperse microdispersions including solid lipid microspheres,Citation27) gel microbeadsCitation28) and giant vesicles.Citation29) Many dispersions encapsulating β-carotene,Citation30) oleuropein,Citation31) γ-oryzanol,Citation32) l-AACitation33) and ascorbic acid derivativesCitation34) have been also prepared using MCE.
Several research groups have encapsulated l-AA at relatively low concentrations (up to 5%) in aqueous droplets using different homogenization techniques.Citation35–38) The main objective of this study is to formulate different pharmaceutical and food-based products such as energy drinks and fortified nutritional supplements containing high concentrations of bioactives. This model study was conducted with n-Decane as the continuous phase that can be replaced with appropriate viscosity oil such as, ethyl oleate, oleic acid or different types of medium chain triglyceride oils. Moreover, this study was conducted to optimize production method for obtaining monodisperse aqueous microspheres containing high concentration of l-AA using straight-through MCE. In this study l-AA was encapsulated at a concentration of 30% (w/w) in aqueous microspheres. We also evaluate the stability of the prepared microspheres and the retention profile of the l-AA encapsulated in them.
Materials and methods
Materials
l-AA (99.9% purity), anhydrous magnesium sulfate (MgSO4) and sodium alginate (Na-ALG) were purchased from Wako Pure Chemical Ind. (Osaka, Japan). All of these chemicals were used for preparing a dispersed phase. Milli-Q water with a resistivity of 18 MΩ cm was used to dissolve all the above-mentioned chemicals. n-Decane was procured from Wako Pure Chemical Ind. and tetraglycerol condensed ricinoleate (TGCR, CR-310) was supplied by Sakamoto Yakuhin Kogyo Co. Ltd (Osaka, Japan). They were used for preparing a continuous phase. Hexamethyldisilazane (LS-7150), purchased from Shin-Etsu Chemical Co. Ltd (Tokyo, Japan), was used for surface hydrophobization of a silicon MC array chip and a glass plate. HPLC grade methanol was used to extract l-AA from aqueous microspheres. All these chemicals were of analytical grade and used without further purification.
Asymmetric MC array chip
In this study we used 24 × 24 mm MC array chip (Model WMS1–2) consisting of 10,313 micrchannels (MCs) (Fig. ) in a 10 × 10 mm in the center of the plate and four 1.5 mm diameter holes at the corners of the chip. This MC array was fabricated by repeated processes of photolithography and deep-reactive-ion etching on 5-inch silicon wafer.Citation39) Each asymmetric MC consisted of a circular microhole (10 μm diameter and 70 μm depth) located on the inlet side and a microslot (11 × 104 μm cross section and 21 μm depth) located on the outlet side. The slot aspect ratio of 9.5 was above the threshold value of 3 for stably generating monodisperse emulsion droplets.Citation40) The fabricated microholes and microslots were highly uniform, meeting the criterion for obtaining monodisperse microparticles. Oxygen plasma treatment was then performed to grow a hydrophilic silicon dioxide layer on the fabricated plate before carrying out hydrophobic treatment. Prior to the first use, the WMS1–2 chips as well as the glass plates were hydrophobilized by using LS-7150 (100% concentration for MC array chip, 20% (w/w) in hexane for glass plate) and left for two nights at room temperature. Afterwards, the unreacted material was washed away by using an ultrasonic bath (VS-100III, As One Co., Osaka, Japan) at a frequency of 100 kHz.
Fig. 1. Schematic representation of asymmetric straight-through MC array chip used in MCE.
Notes: (a) WMS1–2 silicon chip. (b) Optical micrograph of the bottom-illuminated chip surface. The circular MCs are highlighted in dark black color in the center of light grey color microslot. (c) Arrangement of asymmetric MCs on the top side of a WMS1–2 chip. Each horizontal row contains alternatively 82 or 83 MCs and vertical rows contain alternatively 62 or 63 MCs making the total number of MCs equal to 10,313 = 62 × 82 + 63 × 83.
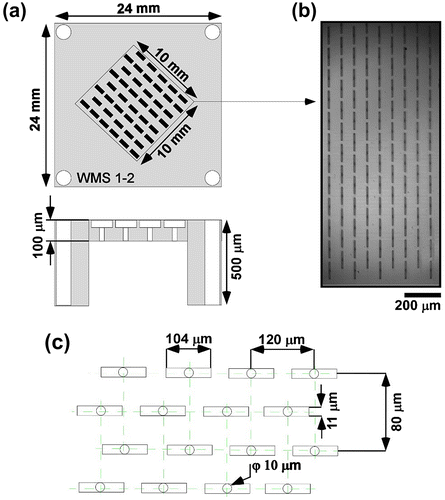
Preparation of aqueous dispersed phase
2.0% (w/w) Na-ALG aqueous solution was prepared by dissolving Na-ALG powder in Milli-Q water for at least 2 h under constant stirring at room temperature. The solution was stored at 4 ± 1°C overnight to ensure complete hydration. The dispersed phase used for straight-through MCE contains 2.0% (w/w) Na-ALG, 1% (w/w) MgSO4 and 5–30% (w/w) l-AA. Na-ALG was chosen on the basis of its functionality in food systems, since it is a plant-based polysaccharide and easily to handle even at high concentration. Moreover, it is widely used for formulation of different types of microspheres. 2.0% (w/w) Na-ALG in the dispersed phase was used after optimization, since an optimized viscosity range was needed to conduct MCE. At higher viscosity it was difficult to pressurize the dispersed phase from the MCs. Moreover, MgSO4 was added to increase the stability of l-AA in the dispersed phase. The continuous phase contains water-saturated decane containing 5% (w/w) TGCR. Water saturation was carried out to prevent water diffusion from the surface of Na-ALG microspheres. Decane was water-saturated by mixing a volume ratio of 9:1 (decane:water) for 30 min, after which they were separated by centrifugation at 1,500 g for 15 min using a table centrifuge (KN-70, Kubota Co., Tokyo, Japan). The decane supernatant part was used as the continuous phase.Citation29) All of the phases were maintained at 45 ± 1°C prior to MCE.
Emulsification procedure
Each emulsification experiment was started with degassing of a WMS1–2 chip soaked in the continuous phase under ultrasonication at a 100 kHz for 20 min. During module assembly, the degassed hydrophobic WMS1–2 chip was mounted in a module compartment previously filled with continuous phase (water saturated decane together with TGCR). Fig. (a) shows a simplified schematic diagram of the experimental setup used for MCE. A hydrophobized silicon MC array plate is tightly attached to hydrophobized glass plate in the emulsification module initially filled with the continuous phase. A syringe pump (Model 11, Harvard Apparatus Inc., Holliston, USA) was used to supply both the continuous and dispersed phases. The heating system provides temperature controlled water circulation inside the module and outside the reservoir. The emulsification process was carried out for approximately 1 h and monitored through FASTCAM-1024 PCI high speed video system at 250–500 fps (Photron Ltd, Tokyo, Japan) attached to an inverted metallographic microscope (MS-511B, Seiwa Optical Co., Ltd., Tokyo, Japan).
Fig. 2. Simplified representation of MCE.
Notes: (a) experimental set-up used in this work for straight-through MCE. (b) Generation process of aqueous microspheres in MCE.
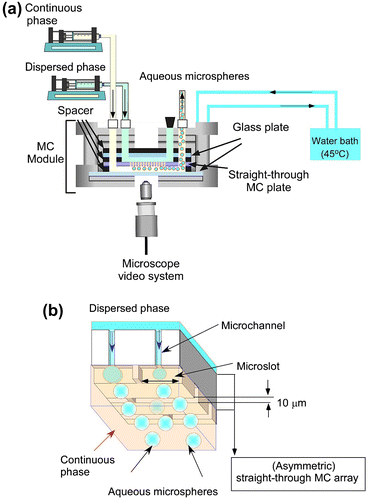
The module temperature was kept at 45 ± 1°C during MCE. Aqueous microsphere generation occurred when the dispersed phase occupies the active surface area of the WMS1–2 chip. The resulting microspheres were then swept away by the cross-flow of the continuous phase. The microsphere generation process is schematically illustrated in Fig. (b). The dispersed phase flux (Jd) (L m−2 h−1) was calculated using the following equation:(1)
where Qd is the dispersed phase flow rate (L h−1) and AMCA is the effective area of a straight-through MC array (1.0 × 10−4 m2). The average flow velocity of the continuous phase over the plate surface (Uc) was calculated with the following equation:(2)
where Qc is the continuous phase flow rate (m h−1), and Aa,s (active surface area) is the flow area over the chip surface (m2) and is calculated by measuring the dimensions of the spacer placed on the WMS1–2 chip:(3)
where W is the spacer internal width (10−2 m), and H is the spacer thickness (10−3 m).
Measurement and analysis
The size and size distribution of the W/O aqueous microspheres obtained from straight-through MCE were determined as follows. The average droplet diameter (dav) was defined by:(4)
where di is the diameter of the ith microsphere measured using WinRoof software (Mitani Co., Ltd, Fukui, Japan) and n is the number of the microspheres measured (n = 250). The microsphere dispersity was expressed as coefficients of variation (CV), and is defined as:(5)
where σ is the standard deviation and dav is the average microsphere diameter (μm).
Measurement of fluid properties
The densities of dispersed and continuous phases were measured using a density meter (DA-130 N, Kyoto Electronics Manufacturing Co., Ltd., Kyoto, Japan) at 25 ± 2 °C. The viscosities of dispersed and continuous phases were measured with a vibro viscometer (SV-10, A&D Co. Ltd, Tokyo, Japan) at 25 ± 2°C by taking either 10 or 35 mL of samples in a measuring vessel followed by immersion of sensor plates in that vessel. Viscosity was measured by detecting the electric current needed to resonate the sensor plates.
The static interfacial tension between the oil and aqueous phases was measured with a fully automatic interfacial tensiometer (PD-W, Kyowa Interface Sciences Co. Ltd, Saitama, Japan) using a pendant drop method. The key physical properties of dispersed and continuous phases are presented in Table .
Table 1. Fluid properties of the two-phase systems containing high concentrations of l-AA, 2% (w/w) sodium alginate (Na-ALG), and 1% (w/w) magnesium sulfate used for preparing aqueous microspheres.
Physical and chemical stability
The liquid microspheres encapsulating l-AA formulated through straight-through MCE were observed with optical micrographs to evaluate dav, CV, consistency, homogeneity, and coalescence during 10 days of storage at 40°C.
The amount of l-AA encapsulated in the microspheres was determined spectrophotometrically. All spectral measurements of their methanolic extracts were carried out using a UV/VIS/NIR spectrophotometer (UV-1700, Shimadzu, Co., Kyoto, Japan). First, 1 mL of the sample containing aqueous microspheres was extracted with 9 mL of methanol, followed by ultrasonication for 20 min. The methanolic extracts were then centrifuged (Avanti, Beckman Coulter, Miami, USA) at 20,000 g for 30 min at 40°C. A 1 mL aliquot of the supernatant was diluted 50 times with methanol and then injected into a quartz cell with a 10 mm pass length. The absorbance of l-AA in extract was measured at 245 nm using an appropriate blank. A representative standard curve of absorbance vs. concentration gave linear least-squares regression with a coefficient of determination (r2) of 0.9996. All experiments were repeated in triplicate and mean values were calculated. The encapsulation efficiency (EE) of l-AA in samples was calculated with the equation:(6)
where Cl-AA is the concentration of l-AA at a given storage time and Cl-AA,0 is the concentration of l-AA initially encapsulated in the microspheres.
Results and discussion
Effect of different operating conditions in MCE for encapsulation of l-AA in microspheres
The experiments were conducted using the dispersed phase containing 5% (w/w) l-AA together with 2% (w/w) Na-ALG and 1% (w/w) MgSO4 in water. The continuous phase contains 5% (w/w) TGCR in water-saturated decane. Uniformly sized W/O microspheres were generated from the asymmetric through holes with a dav of 18.5 μm and a CV of 3.5% (Fig. ). The operating conditions and various physical properties of this two-phase system are summarized in Table and only in this case the generation of aqueous microspheres from asymmetric through-holes was clearly driven by spontaneous transformation of the oil-water interface in and over the microslot, following successful MCE process.Citation41) The generated microspheres detached smoothly without showing any coalescence. Neves et al.Citation32) successfully encapsulated β-carotene in monodisperse oil-in-water (O/W) emulsions with a dav of 28.5 μm and a CV of 3.3% by straight-through MCE.
Fig. 3. Optical micrograph of the model experiment for evaluating preparation conditions of aqueous microspheres.
Note: The generated microspheres contain 5% (w/w) l-AA.
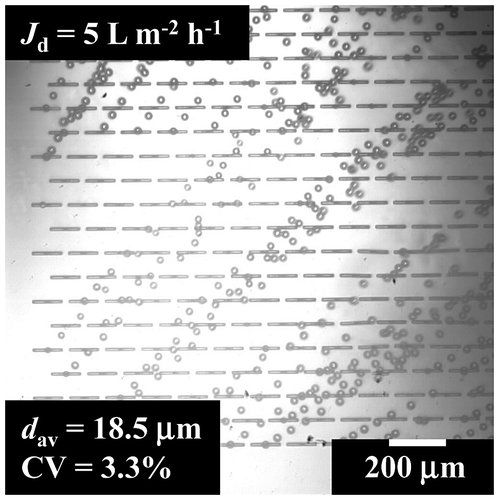
Table 2. Operating conditions and fluid properties of the continuous and dispersed phases.
Dispersed phase flux is an important parameter in MCE that correlates with droplet productivity in the stable microsphere generation zone. Fig. (a) depicts the effect of Jd on the dav and CV of the generated aqueous microspheres. The dispersed phase constitute 5% (w/w) l-AA, 2% (w/w) Na-ALG and 1% (w/w) MgSO4 in water, while the continuous phase includes 5% (w/w) TGCR in water-saturated decane. The straight-through MCE was conducted by maintaining Jd between 5 and 20 L m−2 h−1, while the continuous phase flow velocity was maintained constantly at 2.8 mm s−1.
Fig. 4. Effect of dispersed phase flux on production characteristics of aqueous microspheres.
Notes: (a) The average diameter (dav) and CV of the microspheres with increasing dispersed phase flux (Jd). (●) denotes the dav of the microspheres and (○) indicates their CV. (b) Optical micrographs of microsphere generation at Jd of 10 and 20 L m−2 h−1.
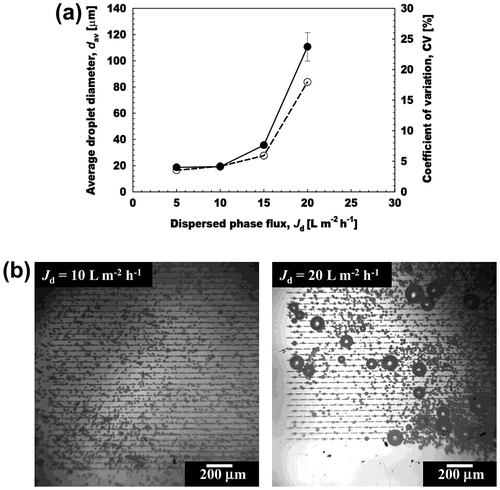
The dav and CV quite slowly increased with increasing Jd until its critical value of 20 L m−2 h−1. Outflow of the dispersed phase and unstable microsphere generation were observed after crossing the critical Jd of 15 L m−2 h−1 (Fig. (b)). The resultant microspheres had dav of 18.7 to 20.7 μm and CV of 3.6 to 6.0%. The average generation frequency per active asymmetric MC (fav,MC) was calculated from number of frames taken to generate 20 microspheres. As the number of frames per second for each video was already known from the high speed video system and could be used to calculate the frequency of microsphere generation:(7)
where f is the frequency of microsphere generation (Hz), nf is the number of microspheres counted, z is the number of frames taken to generate nf droplets and y is the frame rate of the image sequence (Hz). The fav,MC increased with increasing Jd only in a narrow range. At Jd above 10 L m−2 h−1, there was no further increase in fav,MC (Fig. ). The influence of Jd on the microsphere generation frequency per MC array chip (fMCA) is also presented in Fig. . This microsphere generation frequency can be estimated by:(8)
Fig. 5. Effect of dispersed phase flux on microsphere generation frequency and mean productivity containing l-AA.
Notes: (●) Denotes microsphere generation frequency per each active asymmetric microchannel (fMC) and (■) denote microsphere generation frequency per MC array chip (fMCA).
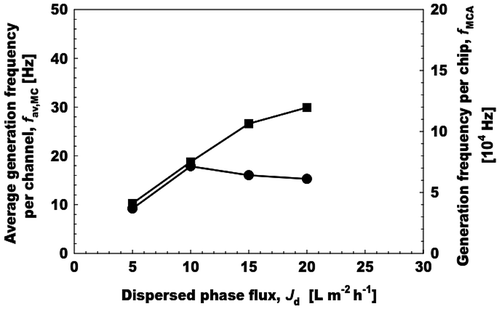
where Vav is the average droplet volume. fMCA increased linearly with increasing Qd at Jd of 5–15 L m−2 h−1. The monodisperse microspheres prepared at Jd of 15 L m−2 h−1 had a maximum productivity of 10.6 × 104 s−1. In this case, we observed that 50% of asymmetric MCs generated uniformly sized microspheres at fav,MC of 16.2 s−1. The maximum dispersed phase flux for preparing monodisperse emulsions usually increases with decreasing the dispersed phase viscosity.Citation42)
Fig. (a) illustrates the effect of l-AA concentration on the dav and CV of the aqueous microspheres prepared by straight-through MCE. The l-AA concentration in the dispersed phase varied from 5 to 30% (w/w) in the presence of 2% (w/w) Na-ALG solution and 1% (w/w) MgSO4. Successful MCE was conducted with different concentrations of l-AA by keeping Jd at 5 L m−2 h−1 and Uc at 2.8 mm s−1 (Fig. (b)). The dav and CV of the resultant microspheres hardly changed up to 20% (w/w) l-AA. Their CV was below 6%, demonstrating monodispersity of the aqueous microspheres obtained here. The dav and CV of microspheres increased to 20.5 μm and 10.7% at the l-AA concentration of 30% (w/w) (Fig. (b)). The variation in monodispersity with increasing the l-AA concentration can be attributed to the interactions between the MC and terrace surfaces with the dispersed phase containing l-AA in the presence of a very thin layer of the continuous phase. Increase of the l-AA concentration in the dispersed phase is assumed to cause stronger interactions with MC and terrace surfaces, hence leading towards less monodispersity.
Fig. 6. Effect of l-AA concentration on aqueous microsphere generation in MCE.
Notes: (a) Effect of l-AA concentration on the average diameter (dav) and CV of the microspheres. (b) Optical micrographs showing generation of aqueous microspheres with increasing l-AA concentration (5–30% (w/w)).
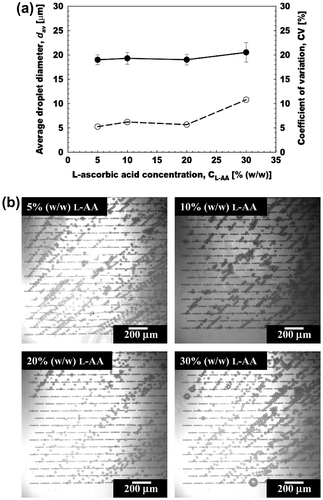
The results obtained here were comparable with our recent study using a grooved MC array. In that study the dav of the aqueous microspheres increased with increasing the l-AA concentration. Our previous studies also encapsulated l-AA of high concentration up to 30% (w/w) in W/O and W/O/W emulsions with similar compositions in the absence of Na-ALG.Citation33,43,44) Viveros-Contreras et al.Citation45) encapsulated 1% (w/w) l-AA in calcium alginate matrix (2% (w/w)). The encapsulation in calcium alginate matrix improved the retention, microstructure and micropore volume of the resultant product. Devi and KakatiCitation46) also encapsulated l-AA in smart porous microparticles based on gelatin/Na-ALG polyelectrolyte complex. These smart microparticles have the potential to improve the stability and delivery of l-AA.
Stability evaluation of aqueous microspheres encapsulating l-AA
MCE using grooved MC arrays provides useful information regarding the basic behaviors of microsphere generation, whereas the drawback lies in low droplet productivity due to the low-density arrangement of microgrooves on a chip.Citation47) In contrast, straight-through MCE has much higher through-put capacity of monodisperse microspheres,Citation42) making it possible to collect microspheres sufficient for stability evaluation.
The monodisperse aqueous microspheres encapsulating 20% (w/w) l-AA were stored at 40 ± 1°C for 10 days. These microspheres were obtained by keeping Jd at 5 L m−2 h−1 and Uc at 2.80 mm s−1. Immediately after collection, the microsphere-containing dispersion had a light yellowish turbid appearance with good flowability. Its appearance did not change with storage time. Fig. depicts time changes in the dav and CV of the resultant microspheres encapsulating l-AA. There was slight increase in their dav and CV during evaluated storage period of 10 days. Their dav and CV after 10 days of storage were 22.3 μm and 10%, respectively.
Fig. 7. Storage stability of the prepared aqueous microspheres containing 20% (w/w) l-AA at 40°C.
Notes: (a) Storage stability of the microspheres during 10 days of storage at 40°C. (●) Denote their average diameter (dav) and (○) denote their CV. (b) Optical micrographs at d 1 and 10.
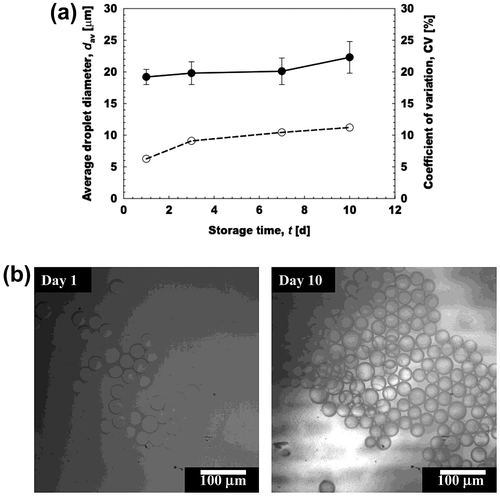
There was slight increase in the dav of the monodisperse W/O/W emulsions encapsulating 30% (w/w) l-AA by straight-through MCE after 10 days of storage.Citation33) These authors pointed out the increased osmotic pressure as a key factor for increased in dav of W/O/W emulsions. 2–20% (w/w) l-AA was encapsulated in solid lipid microcapsules using microfluidic approach.Citation48) The average diameter of these particles varied from 170 to 342 μm during 30 days of storage period.
Encapsulation efficiency of l-AA in aqueous microspheres
To evaluate the chemical stability, the monodisperse aqueous microspheres encapsulating 20% (w/w) l-AA were stored at 40 ± 1°C for 10 days. These microspheres were obtained by keeping Jd at 5 L m−2 h−1 and Uc at 2.80 mm s−1. The freshly prepared aqueous microspheres containing l-AA had an initial concentration of 2.7 mg mL−1 (total emulsion volume) and regarded as 100% EE, since in MCE it was difficult to maintain the volume fraction of the microspheres (φMS) with passage of time in comparison to conventional emulsification techniques. The φMS during MCE corresponds to 0.62%. Fig. indicates the retention and EE of aqueous microspheres. The EE gradually decreased with storage time and exhibited 71% EE of l-AA after 10 days of storage at 40°C. This high EE at 40°C can be ascribed to very mild generation process of microspheres in straight-through MCE. Moreover, in MCE the generation was based upon difference in interfacial tension rather than high energy shear forces. In comparison the conventional emulsification methods produce emulsions with larger droplet sizes and have low energy and encapsulation efficiencies.Citation49)
Fig. 8. Encapsulation efficiency and retention of 20% (w/w) l-AA during 10 days of storage.
Notes: (●) Denotes the l-AA retention in mg mL−1, while (○) indicates the encapsulation efficiency of l-AA in the aqueous microspheres. The retention values were presented as total emulsion volume.
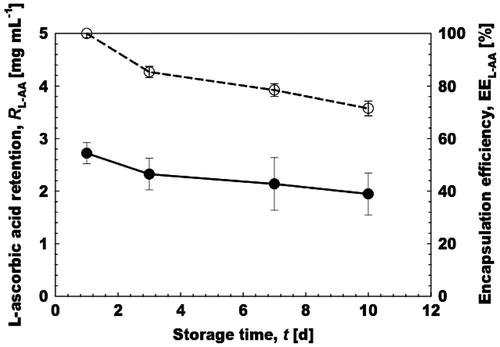
Our results can be considered well when compared to other l-AA encapsulation studies. Farhang et al.Citation50) encapsulated l-AA in liposomes and showed that liposomes have EE of 30% after 7 weeks at 25°C. Rozman and GašperlinCitation51) encapsulated l-AA in W/O microemulsions and reported EE of 60% in the samples stored at room temperature for 28 days. Khalid et al.Citation44) encapsulated high concentration of l-AA in food grade W/O emulsions prepared with either soybean or moringa oil and reported EE of 30% after 30 days of storage at 25°C. Comunian et al.Citation52) encapsulated l-AA by the double emulsion followed by complex coacervation and obtained EEs in the range of 15–40% for samples stored at 37°C for 60 days. Comunian et al.Citation48) recently encapsulated l-AA in solid lipid microcapsules containing different coating agents by glass microfluidic device and obtained EE in the range of 46–96% when stored for 30 days at 20°C.
Conclusion
Monodisperse aqueous microspheres encapsulating a high concentration of l-AA were successfully prepared through straight-through MCE. In this study, we demonstrated that uniformly sized microspheres were stably generated via an asymmetric straight-through MC array, without any significant wetting or outflow of the dispersed phase at optimum processing conditions. The optimum emulsification conditions involve 20% (w/w) l-AA, 2% (w/w) Na-ALG and 1% (w/w) Mg2SO4 in the dispersed phase under Jd of 5 L m−2 h−1, while 5% (w/w) TGCR in water-saturated decane as the continuous phase. The resultant microspheres have good physical and chemical stability for more than 10 days at 40°C. Moreover, they exhibit high EE of more than 70% during 10 days of evaluated period at 40°C. Our results indicate that MCE is a promising technique for encapsulating bioactive compounds, with enhanced control over processing parameters. The monodisperse aqueous microspheres obtained using MCE have potential applications in designing new food and pharmaceutical products with enriched concentration of bioactive substances.
Author contribution
N.K. and I.K. designed the experiments and equally contributed in manuscript writing. N.K. conducted the experiments. M.A.N. and K.U. provided valuable interpretation of results. M.N. and H.N. supervised the research work and provided valuable interpretation of results.
Disclosure statement
No potential conflict of interest was reported by the authors.
Acknowledgments
The first author gratefully acknowledges a scholarship from the Ministry of Education, Culture, Sports, Science, and Technology (MEXT) of Japan and University of Tokyo Fellowship for study at the University of Tokyo and National Food Research Institute, NARO, of Japan.
References
- Shin J, Anisur RM, Ko MK, et al. Hollow manganese oxide nanoparticles as multifunctional agents for magnetic resonance imaging and drug delivery. Angew. Chem. Int. Ed. 2009;48:321–324.10.1002/anie.v48:2
- Yang S, Wang C, Chen L, et al. Facile dicyandiamide-mediated fabrication of well-defined CuO hollow microspheres and their catalytic application. Mat. Chem. Phys. 2010;120:296–301.10.1016/j.matchemphys.2009.11.005
- McQuade DT, Pullen AE, Swager TM. Conjugated polymer-based chemical sensors. Chem. Rev. 2000;100:2537–2574.10.1021/cr9801014
- Freiberg S, Zhu X. Polymer microspheres for controlled drug release. Int. J. Pharm. 2004;282:1–18.10.1016/j.ijpharm.2004.04.013
- Pekarek kJ, Jacob JS, Mathiowitz E. Double-walled polymer microspheres for controlled drug release. Nature. 1994;367:258–260.10.1038/367258a0
- Sinha V, Bansal K, Kaushik R, et al. Poly-ϵ-caprolactone microspheres and nanospheres: an overview. Int. J. Pharm. 2004;278:1–23.
- Dudhani AR, Kosaraju SL. Bioadhesive chitosan nanoparticles: preparation and characterization. Carbohydr. Polym. 2010;81:243–251.10.1016/j.carbpol.2010.02.026
- Qin R, Li F, Chen M, et al. Preparation of chitosan–ethylenediaminetetraacetate-enwrapped magnetic CoFe2O4 nanoparticles via zero-length emulsion crosslinking method. Appl. Surf. Sci. 2009;256:27–32.10.1016/j.apsusc.2009.07.032
- Agnihotri SA, Aminabhavi TM. Controlled release of clozapine through chitosan microparticles prepared by a novel method. J. Controlled Release. 2004;96:245–259.10.1016/j.jconrel.2004.01.025
- Workman VL, Tezera LB, Elkington PT, et al. Controlled generation of microspheres incorporating extracellular matrix fibrils for three-dimensional cell culture. Adv. Funct. Mater. 2014;24:2648–2657.10.1002/adfm.v24.18
- Ochiuz L, Peris J-E. Preparation and characterisation of alendronate-loaded chitosan microparticles obtained through the spray drying technique. Med. Chem. 2009;5:191–196.10.2174/157340609787582963
- Li B-Z, Wang L-J, Li D, et al. Fabrication of starch-based microparticles by an emulsification-crosslinking method. J. Food Eng. 2009;92:250–254.10.1016/j.jfoodeng.2008.08.011
- Ellis A, Jacquier J. Manufacture of food grade κ-carrageenan microspheres. J. Food Eng. 2009;94:316–320.10.1016/j.jfoodeng.2009.03.030
- Prasertmanakit S, Praphairaksit N, Chiangthong W, et al. Ethyl cellulose microcapsules for protecting and controlled release of folic acid. AAPS PharmSciTech. 2009;10:1104–1112.10.1208/s12249-009-9305-3
- Duclairoir C, Orecchioni A, Depraetere P, et al. α-Tocopherol encapsulation and in vitro release from wheat gliadin nanoparticles. J. Microencapsulation. 2002;19:53–60.10.1080/02652040110055207
- Abbas S, Da Wei C, Hayat K, et al. Ascorbic acid: microencapsulation techniques and trends–a review. Food Rev. Int. 2012;28:343–374.10.1080/87559129.2011.635390
- Carr AC, Vissers M. Synthetic or food-derived vitamin C—are they equally bioavailable? Nutritional. 2013;5:4284–4304.
- Weinstein M, Babyn P, Zlotkin S. An orange a day keeps the doctor away: scurvy in the year 2000. Pediatrics. 2001;108:e55–e55.10.1542/peds.108.3.e55
- Yuan J-P, Chen F. Degradation of ascorbic acid in aqueous solution. J. Agric. Food Chem. 1998;46:5078–5082.10.1021/jf9805404
- Munyaka AW, Makule EE, Oey I, et al. Thermal stability of l-ascorbic acid and ascorbic acid oxidase in broccoli (Brassica oleracea var. italica). J. Food Sci. 2010;75:C336–C340.10.1111/(ISSN)1750-3841
- Van den Broeck I, Ludikhuyze L, Weemaes C, et al. Kinetics for isobaric−isothermal degradation of l -ascorbic acid. J. Agric. Food Chem. 1998;46:2001–2006.10.1021/jf9708251
- Sugiura S, Nakajima M, Kumazawa N, et al. Characterization of spontaneous transformation-based droplet formation during microchannel emulsification. J. Phys. Chem. B. 2002;106:9405–9409.10.1021/jp0259871
- Vladisavljevic GT, Kobayashi I, Nakajima M. Production of uniform droplets using membrane, microchannel and microfluidic emulsification devices. Microfluid. Nanofluid. 2012;13:151–178.10.1007/s10404-012-0948-0
- Kobayashi I, Mukataka S, Nakajima M. Production of monodisperse oil-in-water emulsions using a large silicon straight-through microchannel plate. Ind. Eng. Chem. Res. 2005;44:5852–5856.10.1021/ie050013r
- Kobayashi I, Wada Y, Hori Y, et al. Microchannel emulsification using stainless-steel chips: oil droplet generation characteristics. Chem. Eng. Technol. 2012;35:1865–1871.10.1002/ceat.v35.10
- Kawakatsu T, Kikuchi Y, Nakajima M. Regular-sized cell creation in microchannel emulsification by visual microprocessing method. J. Am. Oil Chem. Soc. 1997;74:317–321.10.1007/s11746-997-0143-8
- Sugiura S, Nakajima M, Tong JH, et al. Preparation of monodispersed solid lipid microspheres using a microchannel emulsification technique. J. Colloid Interface Sci. 2000;227:95–103.10.1006/jcis.2000.6843
- Ikkai F, Iwamoto S, Adachi E, et al. New method of producing mono-sized polymer gel particles using microchannel emulsification and UV irradiation. Colloid Polym. Sci. 2005;283:1149–1153.10.1007/s00396-005-1271-z
- Sugiura S, Kuroiwa T, Kagota T, et al. Novel method for obtaining homogeneous giant vesicles from a monodisperse water-in-oil emulsion prepared with a microfluidic device. Langmuir. 2008;24:4581–4588.10.1021/la703509r
- Neves MA, Ribeiro HS, Kobayashi I, et al. Encapsulation of lipophilic bioactive molecules by microchannel emulsification. Food Biophys. 2008;3:126–131.10.1007/s11483-008-9056-9
- Souilem S, Kobayashi I, Neves M, et al. Preparation of monodisperse food-grade oleuropein-loaded w/o/w emulsions using microchannel emulsification and evaluation of their storage stability. Food Bioprocess Technol. 2014;7:2014–2027.10.1007/s11947-013-1182-9
- Neves MA, Ribeiro HS, Fujiu KB, et al. Formulation of controlled size PUFA-loaded oil-in-water emulsions by microchannel emulsification using beta-carotene-rich palm oil. Ind. Eng. Chem. Res. 2008;47:6405–6411.10.1021/ie071552u
- Khalid N, Kobayashi I, Neves MA, et al. Monodisperse W/O/W emulsions encapsulating l-ascorbic acid: Insights on their formulation using microchannel emulsification and stability studies. Colloid Surf. A. 2014;458:69–77.10.1016/j.colsurfa.2014.04.019
- Khalid N, Kobayashi I, Neves MA, et al. Formulation of monodisperse water-in-oil emulsions encapsulating calcium ascorbate and ascorbic acid 2-glucoside by microchannel emulsification. Colloid Surf. A. 2014;459:247–253.10.1016/j.colsurfa.2014.07.014
- Desai KG, Park HJ. Encapsulation of vitamin C in tripolyphosphate cross-linked chitosan microspheres by spray drying. J. Microencapsulation. 2005;22:179–192.10.1080/02652040400026533
- Esposito E, Cervellati F, Menegatti E, et al. Spray dried Eudragit microparticles as encapsulation devices for vitamin C. Int. J. Pharm. 2002;242:329–334.10.1016/S0378-5173(02)00176-X
- Alishahi A, Mirvaghefi A, Tehrani MR, et al. Shelf life and delivery enhancement of vitamin C using chitosan nanoparticles. Food Chem. 2011;126:935–940.10.1016/j.foodchem.2010.11.086
- Goncalves G, Compos P. Shelf life and rheology of emulsions containing vitamin C and its derivatives. Revista de Ciências Farmacêuticas Básica e Aplicada. 2009;30:217–224.
- Kobayashi I, Mukataka S, Nakajima M. Novel asymmetric through-hole array microfabricated on a silicon plate for formulating monodisperse emulsions. Langmuir. 2005;21:7629–7632.10.1021/la050915x
- Kobayashi I, Mukataka S, Nakajima M. Effect of slot aspect ratio on droplet formation from silicon straight-through microchannels. J. Colloid Interface Sci. 2004;279:277–280.10.1016/j.jcis.2004.06.028
- Kobayashi I, Vladisavljevic GT, Uemura K, et al. CFD analysis of microchannel emulsification: droplet generation process and size effect of asymmetric straight flow-through microchannels. Chem. Eng. Sci. 2011;66:5556–5565.10.1016/j.ces.2011.07.061
- Vladisavljevic GT, Kobayashi I, Nakajima M. Effect of dispersed phase viscosity on maximum droplet generation frequency in microchannel emulsification using asymmetric straight-through channels. Microfluid. Nanofluid. 2011;10:1199–1209.10.1007/s10404-010-0750-9
- Khalid N, Kobayashi I, Neves MA, et al. Preparation and characterization of water-in-oil-in-water emulsions containing a high concentration of L-ascorbic acid. Biosci. Biotechnol. Biochem. 2013;77:1171–1178.10.1271/bbb.120870
- Khalid N, Kobayashi I, Neves MA, et al. Preparation and characterization of water-in-oil emulsions loaded with high concentration of l-ascorbic acid. LWT—Food Sci. Technol. 2013;51:448–454.10.1016/j.lwt.2012.11.020
- Viveros-Contreras R, Téllez-Medina D, Perea-Flores M, et al. Encapsulation of ascorbic acid into calcium alginate matrices through coacervation coupled to freeze-drying. Revista Mexicana de Ingeniería Química. 2013;12:29–39.
- Devi N, Kakati DK. Smart porous microparticles based on gelatin/sodium alginate polyelectrolyte complex. J. Food Eng. 2013;117:193–204.10.1016/j.jfoodeng.2013.02.018
- Kobayashi I, Wada Y, Uemura K, et al. Microchannel emulsification for mass production of uniform fine droplets: integration of microchannel arrays on a chip. Microfluid Nanofluid. 2010;8:255–262.10.1007/s10404-009-0501-y
- Comunian TA, Abbaspourrad A, Favaro-Trindade CS, et al. Fabrication of solid lipid microcapsules containing ascorbic acid using a microfluidic technique. Food Chem. 2014;152:271–275.10.1016/j.foodchem.2013.11.149
- Santana RC, Perrechil FA, Cunha RL. High- and low-energy emulsifications for food applications: a focus on process parameters. Food Eng. Rev. 2013;5:107–122.10.1007/s12393-013-9065-4
- Farhang B, Kakuda Y, Corredig M. Encapsulation of ascorbic acid in liposomes prepared with milk fat globule membrane-derived phospholipids. Dairy Sci. Technol. 2012;92:353–366.
- Rozman B, Gašperlin M. Stability of vitamins C and E in topical microemulsions for combined antioxidant therapy. Drug Delivery. 2007;14:235–245.10.1080/10717540601067786
- Comunian TA, Thomazini M, Alves AJG, et al. Microencapsulation of ascorbic acid by complex coacervation: protection and controlled release. Food Res. Int. 2013;52:373–379.10.1016/j.foodres.2013.03.028