Abstract
Enzymatic esterification is an efficient approach for modifying starch to functionalized biomaterials. In this study, conversion of cassava starch to fatty acid acyl esters using immobilized Thermomyces lanuginosus lipase on Fe3O4 microparticles modified with 3-aminopropyltriethoxysilane and covalently linked by 1-ethyl-3-(3-dimethyl aminopropyl) carbodiimide and N-hydroxysuccinimide (Fe3O4-AP-ED-lipase) in a solvent-free system was studied. An optimized reaction containing 5% w/v gelatinized starch, 1% v/v Triton X-100, and 1% w/v biocatalyst with 2.5% w/v of fatty acids (palmitic, oleic, or linoleic acid) resulted in esterified products with a degree of substitution (DS) of 0.12–0.14, while a slightly lower DS was observed using crude palm fatty acid distillate as the acyl donor with 42.9–59.6% recovery yield. Increasing DS led to lower glass transition temperature and higher viscosity of the esterified products. The enzyme showed high operational stability with 85% retaining in activity after recycling in three consecutive batches with simple separation by magnetization, leading to improved process economics.
Graphical abstract
Effects of acyl donors on esterification of starch. The reaction contained 5% gelatinized starch, 2.5% acyl donor, 1% Triton X-100 with 1% enzyme dosage and incubated at 50 °C, pH 7.0 for 24 h with stirring speed at 200 rpm.
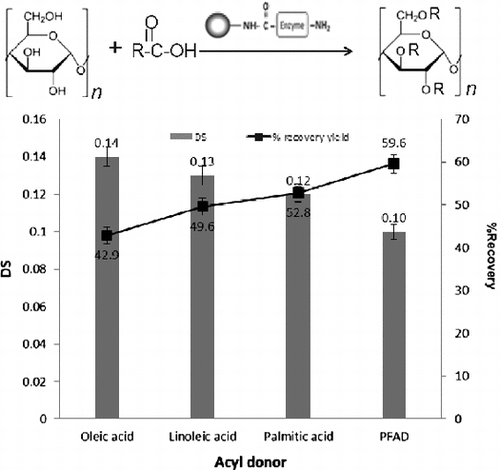
Starch is an abundant, inexpensive, renewable, and fully biodegradable material. However, native starch has many unfavorable properties related to utilization as biomaterials including its high solubility in water and limited solubility in organic solvents, low tolerance to acidity, and poor processing properties. Starch ester is a form of widely used modified biopolymers used in plastic, food, and pharmaceutical industries or biomedical applications. Esterification resulted in changes in the highly hydrophilic hydroxyl groups to the hydrophobic ester groups, which leads to an alteration in physicochemical characteristics of starches.Citation1–3) Esterified starches from various origins have been prepared with variation in their thermal and physicochemical properties related to their further processing and application.Citation4)
Esterification of starch can be achieved by catalytic reactions using acids, alkalis, or enzymes. The acid-catalyzed process using sulfuric acid and acetic acidCitation5–7) has several limitations in catalyst recovery, equipment corrosion, and waste treatment, which affect the cost of the process, while the alkali-catalyzed process suffers from a drawback in recovery of the catalyst. The lipase-catalyzed process is environmentally friendly as it is performed under mild condition and generates no chemical wastes. Lipases from different microbial sources, e.g. Thermomyces lanuginosus,Citation8,9) Candida rugosa,Citation10) and Staphylococcus aureusCitation11) have been studied for esterification of starch from different origins, either in solvent-free or organic media. Acyl donors ranging from short-chain organic acids and anhydrides to long-chain fatty acids were used as the co-substrate for synthesis of starch esters with variation in physicochemical characteristics.Citation2,4,12) However, the use of free soluble lipases in industrial process is still limited by high cost of the enzymes, making the process not economically attractive.
Enzyme immobilization on solid supports by physical adsorption, covalent bonding, or entrapment is used to prepare heterogeneous biocatalysts with advantages on reusability and improved enzyme’s operational stability with less downstream process for product recovery, which thus results in cost reduction of the production process.Citation12) However, a limited design of immobilized lipases has been studied for the synthesis of starch esters, almost with the commercial immobilized C. antarctica lipase on macroporous acrylic resin (Novozymes® 435), which still has a major drawback due to high cost of the biocatalystCitation13–16), while only one example on non-commercial CaCO3-immobilized lipase from S. aureus has been reported.Citation11) Immobilization of lipases on magnetic micro/nanoparticles has been introduced as an interesting approach for fabrication of high-performance biocatalysts with an advantage on simple enzyme separation by magnetization for recovery and recycling. Magnetic micro/nanoparticle lipases in different designs using various modifications of the core iron matrix and covalent linkages have been studied for catalyzing reactions in aqueous and non-aqueous media. Immobilization of lipase on Fe3O4 treated with (3-aminopropyl) triethoxysilane (APTES) using glutaraldehyde as a coupling reagent was first reported for transesterification of soybean oilCitation17) and then with the use of immobilized lipase linked to the magnetic support by 1-ethyl-3-(3-dimethylaminopropyl) carbodiimide (EDAC).Citation18) Composite inorganic or biological polymers can improve magnetic micro/nanoparticle-immobilized lipase stability by strengthening the core magnetic structures or modifying physical properties of the magnetic carriers, e.g. chitosan-coated Fe3O4 nanoparticle,Citation19) magnetic composite poly(styrene-methacrylic acid) microsphere,Citation20) and Fe3O4/SBA-15 composite.Citation21) These magnetic immobilized lipases were applied for catalyzing various reactions, for example, biodiesel productionCitation17–21) and synthesis of glycerol carbonate.Citation22)
Recently, preparation of an immobilized lipase on Fe3O4 modified by 3-aminopropyltriethoxysilane and covalently linked by 1-ethyl-3-(3-dimethylaminopropyl) carbodiimide and N-hydroxysuccinimide (NHS) (Fe3O4-AP-ED-lipase) was reported as a high-performance biocatalyst design with high operational stability in transesterification of palm oil.Citation23) In this study, the biocatalytic esterification of cassava starch with long-chain acyl donors, i.e. palm-derived fatty acids and crude palm fatty acid distillate (PFAD) from palm oil processing industry using Fe3O4-AP-ED-lipase, was studied. Physicochemical analysis of the esterified starch showed modification of starch properties with potential for further processing of materials for plastic and biomedical industries. The work demonstrates a promising approach for improving cost-efficiency in eco-friendly biocatalytic synthesis of starch esters.
Materials and methods
Materials
Commercial-grade cassava starch was purified by washing with distilled water repeatedly and dried in an oven at 50 °C for 24 h. Analytical-grade free fatty acids (palmitic acid, oleic acid, and linoleic acid) were purchased from Sigma-Aldrich. PFAD was obtained from Pathum Vegetable Oil, Co. Ltd. (Pathum Thani, Thailand) containing 93% (w/w) free fatty acid (45.6% palmitic, 33.3% oleic, and 7.7% linoleic as the major components) and the rest comprising triglycerides, diglycerides, monoglycerides, and trace impurities. Lipolase, a liquid-lipase from Thermomyces lanuginosus, was purchased from Novozymes AS (Bagsvaerd, Denmark).
Preparation of immobilized lipase on magnetic microparticles
The magnetic microparticle support (Fe3O4 microparticle) and immolized lipase were prepared by the method of Xie and MaCitation18) as modified by Raita et al. 2014.Citation23) Fe2SO4·7H2O (2.78 g) and FeCl3·6H2O (5.4 g) equivalent to a molar ratio of 1:2 were dissolved in 100 mL distilled water at a final concentration of 0.3 mol/L Fe2+/Fe3+ combined ions. The iron microparticles were co-precipitated by drop-wise addition of 75 mL of 25% ammonium hydroxide solution at 25 °C in a stirring vial. The suspension was incubated at 80 °C for 30 min and then cooled down to room temperature. The precipitate was separated by centrifugation at 5000 × g and washed thrice with distilled water. The Fe3O4 microparticle (0.25 g) was mixed with 0.15 m APTES in 4.85 mL ethanol. The mixture was sonicated at a frequency of 35 kHz with shaking overnight at room temperature. The product was separated by magnetization, washed thrice with 5 % ethanol, and then dried in a dry oven at 50 °C.
The lipase solution (2.5 mL) was mixed with 0.5 mL of 2.5 mg/mL 1-ethyl-3-(3-dimethylaminopropyl) carbodiimide solution (EDC) and incubated at room temperature for 2 h with shaking at 200 rpm. The mixture was added to 3 mg of NHS and incubated under the same condition for an additional 2 h. The solution was then added to 0.25 g of Fe3O4-APTES microparticles and further incubated for 2 h. The immobilized lipase was separated by magnetization and then washed with distilled water for several times until no free lipase was detected in the supernatant as determined by Bio-Rad Bradford’s assay reagent (Bio-Rad, Hercules, CA) using bovine serum albumin as a standard. The biocatalyst was air-dried and stored at 4 °C for subsequent study.
Lipase activity assay
Lipase hydrolysis activity was assayed based on hydrolysis of p-nitrophenylpalmitate.Citation24) The standard reaction (200 μL) contained 20 mM sodium phosphate buffer, pH 8, 1 mM of p-nitrophenylpalmitate, and 10 mg of the immobilized enzyme. The reaction was incubated at 45 °C for 30 min and then terminated by addition of 100 μL of 0.2 M Na2CO3. The formation of p-nitrophenolate was determined by measuring the absorbance at 405 nm. One unit of the enzyme activity was defined as the amount of enzyme catalyzing the release of 1 μmole p-nitrophenolate/min under the standard experimental conditions.
Synthesis of starch ester
An optimized starch esterification reaction (100 mL) contained 5% (w/v) of cassava starch gelatinized in distilled water, 2.5% (w/v) fatty acid, 1% (v/v) Triton X-100, and 1% (w/v) of immobilized lipase. The reaction was incubated at 50 °C, pH 7.0 as adjusted by NaOH for 24 h. After the reaction completed, the starch ester product was precipitated by adding 20 mL ethanol. The immobilized lipase was separated using magnetization. The precipitated starch ester was separated by centrifugation at 5000 × g, washed with ethanol, and dried at 50 °C for 24 h. The product recovery yield was defined as the percentage of starch ester obtained based on the starting substrate on a dry weight basis.
The %substitution (%Sub) and degree of substitution (DS) of starch ester were determined according to Jerachaimongkol et al.Citation2) The starch ester sample (5 g) was resuspended in 50 mL of distilled water. The sample was saponified with 25 mL of 0.1 M NaOH and incubated at 25 °C for 24 h. The reaction was titrated with 0.1 M H2SO4 to the original pH using phenolphthalein as an indicator. The reactions were performed in triplicate, and the averages were reported with standard deviation. The %substitution and DS were calculated as follows:
where MW = molecular weight of ester group, WS = weight of sample (g), V = amount of sulfuric acid for equivalence point in titration (mL), and N = concentration of sodium hydroxide (M).
where 162 = molecular weight of anhydroglucose unit, MW = molecular weight of ester group.
BET surface area measurement
The method of Brunauer, Emmett, and Teller (BET) was used to determine the average of particles size and total surface area of materials. The immobilized enzyme was analyzed for the BET surface area using a Belsorp-max TPDpro (BEL Japan, Tokyo, Japan) with a thermal conductivity detector (Semi-diffusion-type, 4-element W-Re filament) at the National Metal and Material Technology Center, Thailand.
X-ray diffraction analysis (XRD)
X-ray diffraction (XRD) data of the magnetic microparticle support and the immobilized lipase were collected at room temperature on a Rigaku TTRAX III X-ray diffractometer using Cu Kα radiation (λ = 1.5418Å). The sample was scanned in the 2θ value of 10–45° at a rate of 2°/min.
Fourier transfer infrared (FT-IR) spectroscopyKindly suggest whether the term “Fourier transfer infrared (FT-IR) spectroscopy” can be changed as “Fourier transform infrared (FT-IR) spectroscopy”
The functional groups on immobilized lipase and starch ester products were analyzed by FT-IR (Perkin-Elmer System 2000, Waltham, MA) with infrared spectra collected in the 400–4,000 cm−1 wave number range. The starch ester sample was ground to fine powder and mixed with dry potassium bromide before casting into a film for FT-IR analysis.
Thermal analysis
The glass transition temperatures (Tg) of the control gelatinized starch and starch ester products (moisture content ≈10%) were analyzed by differential scanning calorimetry (DSC) on a DSC822 differential scanning calorimeter (Mettler Toledo, Columbus, OH, USA). Temperature scanning was from 20 to 250 °C at a rate of 10 °C/ min under nitrogen atmosphere.
Viscosity measurement
Viscosity of control starch and starch ester solution (1% w/v in distilled water) was measured using a rheometer (Bohlin Gemini HR nano, Malvern Instruments, UK). The parameters used for viscosity analysis by the frequency sweep test are as follows: frequency range 0.05–200 rad/s with 20 Pa stress at 50 °C for 15 min.
Results and discussion
Characterization of immobilized lipase on magnetic microparticles
Immobilization of lipase on Fe3O4 magnetic support was performed, and the heterogeneous biocatalyst was characterized for its biochemical activities and physical characteristics. The pre-concentrated lipase solution had a specific activity of 2.9 × 10−3 IU/mg protein based on hydrolysis of p-nitrophenylpalmitate. The free enzyme was bound to the Fe3O4 matrix modified by APTES via EDC and NHS with a high immobilization efficiency of 81.6% based on total protein recovery. The immobilized lipase (Fe3O4-AP-EN-lipase) showed a catalytic activity of 2.9 × 10−2 IU/g of the biocatalyst, equivalent to a specific activity of 5.12 × 10−4 IU/mg protein immobilized on the solid support. The decrease in specific activity of enzymes upon immobilization could be due to several factors, for examples, partial enzyme inactivation by chemical reactions used for immobilization and alteration in enzyme’s molecular structure and flexibility in the solid biocatalyst.Citation25)
The average size of the protein-free magnetic particles matrix was in micron-scale (50–100 μm) according to scanning electron microscopy analysis (data not shown) with a specific surface area of 19.3 m2/g based on BET analysis. The formation of bound enzyme on the carrier was demonstrated by FT-IR (Fig. (A)). The protein-free Fe3O4 showed the signature adsorption peaks at 579 and 3428 cm−1, which were assigned to the Fe–O stretching vibrations and the hydroxyl group, respectively. The free lipase showed the signature peaks at 1650 cm−1 for the carbonyl amide group (O=C stretching vibration) and 1548 cm−1 for the amide group from N–H bending vibration. The FT-IR spectra of the biocatalyst showed a combination of the signature peaks of both the Fe3O4 matrix and the free lipase at 564, 1541, 1639, and 3404 cm−1, indicating the formation of bound enzyme molecules on the carrier. The spectral pattern corresponded well with the spectra of magnetic micro/nanoparticle support previously reported by Xie and Ma.Citation18) The immobilized lipase showed the same XRD pattern of the protein-free Fe3O4 matrix with six characteristic peaks at 2θ of 30.3°, 35.6°, 43.4°, 53.9°, 57.3°, and 62.9° (Fig. (B)). The results did not show any structural changes of the iron particle upon binding to lipase.Citation18)
Esterification of cassava starch
The efficiency of the immobilized lipase on starch esterification was measured based on the DS and %recovery yield. DS indicates the moles of substituents of hydroxyl groups per anhydroglucose unit in starch which have the maximal value of 3 according to available free OH groups available per an anhydroglucose unit. The effects of reaction parameters (enzyme loading, time, and acyl donor) on esterification of cassava starch with oleic acid by the immobilized lipase were studied. No detectable DS was found for the product from the control reaction with no enzyme. Increasing enzyme loading from 0.25 to 4% (w/v) led to an increase of DS from 0.06 to 0.16, however with a decreasing %recovery of the starch ester product from 43.8 to 24.2% (Fig. (A)). The highest DS was obtained at 2.5% of oleic acid as an acyl donor in the reaction (Fig. (B)). Further increase in the co-substrate led to a marked decrease in DS. This could be due to the incomplete solubility and dispersion of the fatty acid in the reaction which led to the decrease in enzyme efficiency in the heterogeneous phase reaction. A marked increase in DS was observed within the first 24 h of the reaction, which led the DS of 0.14 (Fig. (C)). No substantial increase in DS was found with longer incubation time yet with increasing yield. The highest %recovery of 66.2 was achieved at 48 h under the experimental conditions. The optimal conditions were defined at 1% enzyme dosage with 2.5% acyl donor which led to the final DS of ≥0.14, which was at least 85% of the maximal DS obtained.
Fig. 2. Effects of reaction parameters on esterification of starch.
Notes: (A) enzyme loading: at fixed oleic acid (2.5%) and time (24 h); (B) %Oleic acid: at fixed enzyme dosage (1%) and time (24 h); (C) reaction time: at fixed enzyme dosage (1%) and %oleic acid (2.5%). The reaction contained 5% gelatinized starch, 1% Triton X-100 and was incubated at 50 °C, pH 7.0 with stirring speed at 200 rpm.
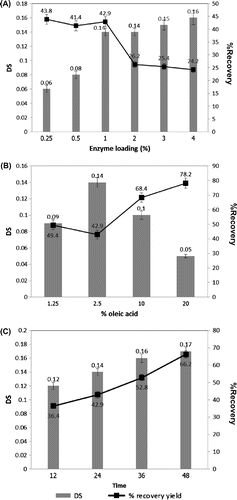
Variation of acyl groups on the esterification of starch was studied at the fixed condition for other reaction variables under the optimal conditions (Fig. ). After incubating at 50 °C, pH 7 for 24 h, the obtained starch ester yield was in the range of 42.9–59.6% using different free fatty acids as the acyl donors. Variation in DS (0.12–0.14) was observed using different free fatty acids. The use of oleic acid (C18:1) led to the starch ester product with the highest DS (0.14), but the lowest recovery yield (42.9%) compared with linoleic (C18:2) and palmitic acid (C16:0). A higher recovery yield was obtained using PFAD as the acyl donor, but with the lowest DS (0.10).
Fig. 3. Effects of acyl donors on esterification of starch.
Notes: The reaction contained 5% gelatinized starch, 2.5% acyl donor, 1% Triton X-100 with 1% enzyme dosage and was incubated at 50 °C, pH 7.0 for 24 h with stirring speed at 200 rpm.
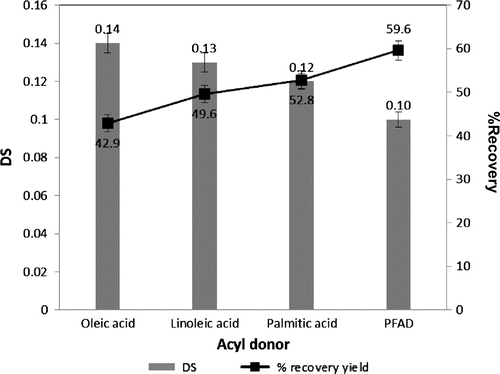
Esterification of acyl group on starch was demonstrated by FT-IR. The FT-IR spectra of the native and esterified starch using different acyl donor are shown in Fig. . The adsorption peaks at 1159, 1082, 1018, and 929 cm−1 associated with C–O bond stretching vibrations were identified for the native starch. The peaks at 1159 and 1018 cm−1 are attributed to the anhydroglucose ring O–C stretch. A strong absorption band at 1018 cm−1 was correlated to the C–OH band stretching vibrations. A broad band appeared at 3399.11 cm−1 which represented the hydrogen bonded hydroxyl group (O–H) which is characteristic for the complicated vibrational stretches related to the free inter- and intra- molecular bound hydroxyl groups. The band at 2930.97 cm−1 was assigned for the aliphatic C–H stretching vibrations. The signature peak of C=O absorption frequency appeared at 1734–1738 cm−1 for the starch esters linked with the different acyl donors. The presence of the signature peak in the esterified starches was in accordance with those reported for other starch esters.Citation8,11,14,26) The peak of FT-IR of starch sample from the control reaction without enzyme showed the same peak pattern to the native starch (data not shown).
Reusability of Fe3O4-AP-EN lipase
The reusability of Fe3O4-AP-EN microparticle lipase on esterification was studied by analyzing the conversion efficiency after the consecutive batch cycles (Fig. ). The biocatalyst was separated from the reaction using magnetization and washed with ethanol to remove the bound fatty acid before reusing in the next batch without further re-activation. The biocatalyst retained high operational stability after repeated use with >85% of the original activity remaining as reflected by the DS obtained after three consecutive batch cycles under the experimental conditions. Further reuse of the enzyme led to a marked decrease in DS of the product. This result thus demonstrates the potential of Fe3O4-AP-EN lipase in the consecutive batch process.
Fig. 5. Reusability of the magnetic microparticle lipase in consecutive batch process.
Notes: The reaction contained 5% w/v gelatinized starch, 2.5% w/v oleic acid, and 1% v/v Triron X-100 with 1% w/v Fe3O4-AP-EN lipase. The mixture was incubated at 50 °C, pH 7 for 24 h with stirring speed at 200 rpm. The biocatalyst was separated by magnetization and washed with ethanol before reused in the subsequent batch. The remaining activity is calculated based on the obtained DS divided by the DS obtained in batch 1.
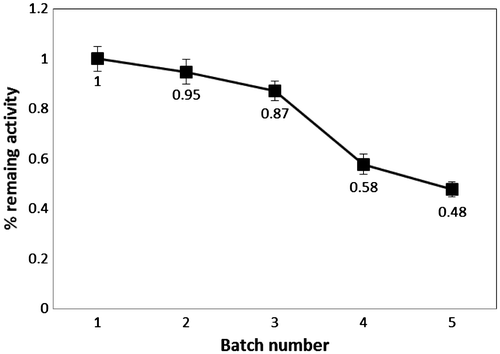
Physicochemical analysis of starch esters
The effects of esterification on thermal properties of the starch esters compared to the control gelatinized cassava starch were studied by DSC (Table ). The control starch had a high Tg midpoint calculated by DIN methodCitation27) of 190.24 °C, while decreasing Tg was observed for the esterified starches in the order of PFAD > palmitic acid > linoleic acid > oleic acid. The decreasing tendency of Tg for the starch esters prepared with different acyl donors was opposite to the increasing trend of DS. Reduction in Tg was due to an interruption of inter-molecular hydrogen bonds by substitution of the hydroxyl group in the unmodified starch with the acyl group which induced loss of crystallinity of the native starch.Citation11) Decrease in Tg with increasing length of fatty acid chain in starch esters has been previously reported for oleate esterified maize starch.Citation11) The Tg for the control and esterified starches reported in this study was in the same range compared to those previously reported for starch esterified by recovered coconut oil.Citation8)
Table 1. Thermal and physicochemical properties of starch esters.
The solubility and viscosity properties of starch esters were related to their further processing and application. An increasing viscosity of the starch ester (1.25–6.52 Pa.s) was observed for the esterified starches compared with the control starch (1.04 Pa.s) (Table ). Esterification was reported to lead to a decrease in hydrophilicity and an increase in hydrophobicity of starch.Citation28) The highest viscosity was observed with oleic acid, followed by linoleic acid and palmitic acid, respectively, while the esterified starch with PFAD showed the lowest increase in viscosity. This suggests that the increase in viscosity was correlated to DS of the starch ester. Substitution of the hydroxyl groups with the hydrophobic acyl groups resulted in increasing viscosity of the esterified starch. The viscosity was found to increase with higher hydrophobicity of the acyl chains.Citation8) This also led to lower water solubility of esterified starch with long-chain acyl groups compared to those with short-chain acyl groups.Citation29)
Enzymatic acylation of starch has been recently reviewed.Citation4) However, most of the previous works on biocatalytic esterification of starch were based on the use of free lipases, while there are only few reports on application of immobilized lipases. A commercial immobilized C. antarctica lipase (Novozyme® 435) was studied for esterification of cassava starch with rosin acid, which led to a production of esterified starch with improved thermal properties and hydrophobicity for application in waterproof coating and plasticCitation16) and also on esterification of starch microparticle in microemulsions with different acyl donors, e.g. vinyl stearate, ε-caprolactone, and maleic anhydride.Citation13) An S. aureus lipase immobilized on CaCO3 was reported for esterification of maize starch with oleic acid in a microwave heating process followed by liquid-state esterification.Citation11) This resulted in a preparation of starch esters with various acyl groups at varying DS for different industrial applications. According to their physicochemical properties, the thermoplastic starch esters prepared in our study would find potential application in pharmaceutical and plastic industries.
In conclusion, a biocatalytic process using immobilized lipase on magnetic microcarriers for efficient conversion of starch to esterified starch was firstly demonstrated with the recently reported high-performance Fe3O4-AP-ED-lipase. The starch esters coupled by long-chain acyl groups showed the distinct thermal and viscosity properties compared with starch esters linked to short-chain acyl groups, showing their potential on different applications. The magnetic microparticle lipase showed high operational stability and allowed reusability by simple separation by magnetization, which could thus lead to cost reduction in production of starch esters in a solvent-free system. Further study will be focused on reaction kinetic study in order to provide important basis for up-scaling of the developed biocatalytic process with competitive advantages to the current chemical and enzymatic starch ester synthesis reactions.
Author contribution
NP and MR conducted the experimental works and prepared the first draft. VC and NL designed the experiments, analyzed the results, and prepared the final manuscript for submission.
Supplemental material
The supplemental material for this study is available at http://dx.doi.org/10.1080/09168451.2015.1056507.
Disclosure statement
No potential conflict of interest was reported by the authors.
Funding
The work was supported by the Discovery Based Development Grant from the National Science and Technology Development Agency and the Thailand Research Fund. [grant number D142]
Acknowledgements
The authors would like to thank Dr. Sithichoke Tangphatsornruang for proofreading the manuscript.
References
- Kiatkamjornwong S, Thakeow P, Sonsuk M. Chemical modification of cassava starch for degradable polyethylene sheets. Polym. Degrad. Stab. 2001;73:363–375.10.1016/S0141-3910(01)00100-8
- Jerachaimongkol S., Chonhenchob V., Naivikul O., et al. Modification of cassava starch by esterification and properties of cassava starch ester films. Kasertsart J. Nat. Sci., 40, 148–151 (2006).
- Dastidar TG, Netravali AN. ‘Green’ crosslinking of native starches with malonic acid and their properties. Carbohydr. Polym. 2012;90:1620–1628.10.1016/j.carbpol.2012.07.041
- Alissandratos A, Halling PJ. Enzymatic acylation of starch. Bioresour. Technol. 2014;115:41–47.
- Lepeniotis S, Feuer B. Synthesis of starch acetate: Statistical designed experiments to optimize the reaction conditions. Chemom. Intell. Lab. Syst. 1997;36:229–243.10.1016/S0169-7439(96)00071-8
- Shogren RL. Rapid preparation of starch esters by high temperature/pressure reaction. Carbohydr. Polym. 2003;52:319–326.10.1016/S0144-8617(02)00305-3
- Guan J, Eskridge KM, Hanna MA. Acetylated starch-polylactic acid loose-fill packaging materials. Ind. Crops Prod. 2005;22:109–123.10.1016/j.indcrop.2004.06.004
- Rajan A, Prasad V.S.. Enzymatic esterification of starch using recovered coconut oil. Int. J. Biol. Macromol. 2006;39:265–272.10.1016/j.ijbiomac.2006.04.006
- Alissandratos A, Baudendistel N, Flitsch SL, et al. Lipase-catalysed acylation of starch and determination of the degree of substitution by methanolysis and GC. BMC Biotechnol. 2010;10:82.10.1186/1472-6750-10-82
- Rajan A, Sudha JD, Abraham TE. Enzymatic modification of cassava starch by fungal lipase. Ind. Crops. Prod. 2008;27:50–59.10.1016/j.indcrop.2007.07.003
- Horchani H, Chaâbouni M, Gargouri Y, et al. Solvent-free lipase-catalyzed synthesis of long-chain starch esters using microwave heating: Optimization by response surface methodology. Carbohydr. Polym. 2010;79:466–474.10.1016/j.carbpol.2009.09.003
- Tan T, Lu J, Nie K, et al. Biodiesel production with immobilized lipase: A review. Biotechnol. Adv. 2010;28:628–634.10.1016/j.biotechadv.2010.05.012
- Chakraborty S, Sahoo B, Teraoka I, et al. Enzyme-catalyzed regioselective modification of starch nanoparticles. Macromolecules. 2005;38:61–68.10.1021/ma048842w
- Xin J-Y, Wang Y, Liu T, et al. Biosysthesis of corn starch palmitate by lipase Novozym 435. Int. J. Mol. Sci. 2012;13:7226–7236.10.3390/ijms13067226
- Wang Y, Xin J, Shi J, et al. A kinetic study of starch palmitate synthesis by immobilized lipase-catalyzed esterification in solvent free system. J. Mol. Catal. B: Enzym. 2014;101:73–79.10.1016/j.molcatb.2014.01.003
- Lin R, Li H, Long H, et al. Optimization of lipase-catalyzed rosin acid starch synthesis by response surface methodology. J. Mol. Catal. B: Enzym. 2014;105:104–110.10.1016/j.molcatb.2014.04.010
- Xie W, Ma N. Immobilized lipase on Fe3O4 nanoparticles as biocatalyst for biodiesel production. Energy Fuels. 2009;23:1347–1353.10.1021/ef800648y
- Xie W, Ma N. Enzymatic transesterification of soybean oil by using immobilized lipase on magnetic nano-particles. Biomass Bioenergy. 2010;34:890–896.10.1016/j.biombioe.2010.01.034
- Xie W, Wang J. Immobilized lipase on magnetic chitosan microspheres for transesterification of soybean oil. Biomass Bioenergy. 2012;36:373–380.10.1016/j.biombioe.2011.11.006
- Xie W, Wang J. Enzymatic production of biodiesel from soybean oil by using immobilized lipase on Fe3O4/poly(styrene-methacrylic acid) magnetic microsphere as a biocatalyst. Energy Fuel. 2014;28:2624–2631.10.1021/ef500131s
- Zang X, Xie W. Enzymatic interesterification of soybean oil and methyl stearate blends using lipase immobilized on magnetic Fe3O4/SBA-15 composites as a biocatalyst. J. Oleo. Sci. 2014;63:1027–1034.10.5650/jos.ess14089
- Tudorache M, Negoi A, Protesescu L, et al. Biocatalytic alternative for bio-glycerol conversion with alkyl carbonates via a lipase-linked magnetic nano-particles assisted process. Appl. Catal., B. 2014;145:120–125.10.1016/j.apcatb.2012.12.033
- Raita. M., Arnthong, J., Champreda, V., et al. Modification of magnetic nanoparticle lipase designs for biodiesel production from palm oil. Fuel Proc. Technol. 2015;134:189–219.
- Gilham D, Lehner R. Techniques to measure lipase and esterase activity in vitro. Methods. 2005;36:139–147.10.1016/j.ymeth.2004.11.003
- Zhu J, Sun G. Lipase immobilization on glutaraldehyde-activated nanofibrous membranes for improved enzyme stabilities and activities. React. Funct. Polym. 2012;72:839–845.10.1016/j.reactfunctpolym.2012.08.001
- Boruczowska B, Boruczkowski T, Leszcynski W, et al. The obtaining of starch-and oleic acid-based ester and its properties. Nauka Technologia. 2012;4:98–107.
- Shawe JEK, Collected applications themal analysis. Elastomers, Vol. l, Mettler Toledo Collected Application (2002).
- Fathi F, Namazi H. Characterization and solvent free-synthesis of modified potato starch. J. Mater. Chem. Eng. 2014;2:11–15.
- Namazi H, Fathi F, Dadkhan A. Hydrophobically modified starch using long-chain fatty acids for preparation of nanosized starch paticles. Scientia Iranica C. 2011;18:439–445.