Abstract
This work revealed peanut seed prolamins likely displaying a defensive role besides the known nitrogen storage. Drought stress and proteomic approaches were used in varieties of peanuts to explore the prolamin member in association with a test against Aspergillus flavus spore germination. The stress effect was showed by aerial biomass, leaf content of malondialdehyde, and seed contamination by A. flavus. Sodium dodecyl sulfate–polyacrylamide gel electrophoresis profiles were not informative for the antifungal polypeptides. From two-dimensional gel electrophoresis, the suspected polypeptides were those with pI 5.45–5.75 and sizes of 22.0–30.5 kDa specifically in Spanish-type peanuts. Regarding to the drought effect in most of these peanuts, the spot peak volume analysis deduced three novel prolamin-related antifungal polypeptides at pI 5.75–5.8 with 30.5, 27.5–28.5, and 22.0–22.5 kDa, which was confirmed after isoelectric purification at pH 5.60. The data could not yet conclude their correlation with resistance to drought and to seed infection by A. flavus.
Graphical Abstract
Antifungal peanut prolamins were related with polypeptides 1, 2, and 3 in pellet (Fig. A) not with spots 4, 5, and 6 in the supernatant (Fig. B) portion on precipitation at pH 5.60.
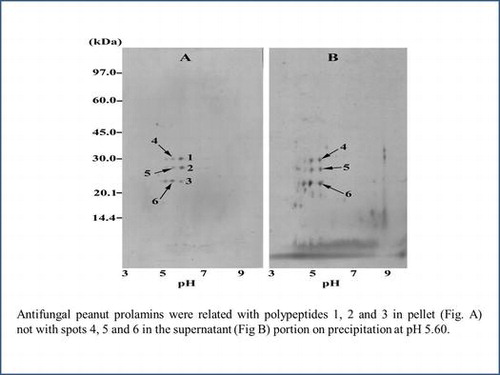
The market-type peanuts (Arachis hypogaea L.) including the runner, Virginia, Spanish, and Valencia are cultivated worldwide in tropical and warm temperate zones. Due to the poor water management in the globule peanut planting areas,Citation1) the peanut plant frequently exposes to water deficit (or drought) during crop season. As a result from drought during pod development, peanut pods are at high risk of infection by soil microorganisms, and the most importance is Aspergillus flavus infection which is causative for the contamination of aflatoxin.Citation2) The drop in seed kernel water activity during drought causes the reduction in phytoalexin synthesis, which was proposed to be the critical involvements.Citation3,4) Otherwise, drought causes plant weakness from oxidative impair of cell-to-organ functions which somehow promotes A. flavus invasion.Citation5)
Several workers have published electrophoretic evidence of seed proteins in peanut genotypes. The simple sodium dodecyl sulfate–polyacrylamide gel electrophoresis (SDS–PAGE) was successful in a few cases.Citation6,7) However, extensive successes were obtained using two-dimensional gel electrophoresis (2D-GE) in several aspects.Citation8–10) 2D-GE also demonstrated distinct changes in proteomic of total seed storage proteins in response to water deficit solely or in couple with A. flavus inoculation, comparing between drought susceptible and resistant genotypes.Citation11,12) Those findings were found mostly in association with peanut seed globulins and albumins, in the sense of allergens and antifungal proteins.Citation9,10,12,13) Peanut seed proteins contain three known classes of storage proteins including globulins (94–95%), albumins (2–3%), and prolamins (1–2%).Citation14) Information of prolamins has been restricted to that of cereal seeds with an importance as primary nitrogen reserves.Citation15) Native prolamins are hydrophobic and extractable in aqueous alcohol solutions like 50–55% propanol or 60–70% ethanol.Citation16) Our previous work described an extraction method for peanut seed prolamins which retarded A. flavus spore germination comparing to the globulin or albumin fraction.Citation17) No other works on prolamins have been published in peanut genotypes, but the Ara h 9 (a non-specific lipid transfer protein) in the peanut seed allergen prolamin superfamily displays an antifungal property.Citation13)
This study aimed to use the proteomic study, peanut genotypes with different susceptibility to drought and drought treatment as tools to investigate seed prolamin members in association with the anti-A. flavus activity. The experiments were conducted with five Spanish-type and one Virginia-type genotypes. A drought condition for peanut plantings was set up in parallel with the non-drought condition since the anti-A. flavus prolamin members might be drought-inductive especially in the resistant genotype. To accomplish our purposes, one part of the work prior to the proteomic study was dealt with demonstrations of the drought establishment on peanut plants.
Materials and methods
Planting design
Four drought resistant Spanish-type peanuts from the International Crops Research Institute for Semi-Arid Tropics (ICRISAT; India) included ICGV 98300, 98303, 98305, and 98308.Citation18) Two genotypes of drought-sensitive traits cropped in Thailand were the Virginia-type KK 60-3 and the Spanish-type Tainan-9.Citation18) Soil water holding at control field capacity or drought condition at 1/3 available water was instrumentally evaluated at 15.00 and 7.33% v/w, respectively. The water volume for monitoring control or drought condition was calculated.Citation19) The peanut plant stages were defined by following the classical description.Citation20)
Peanut plants were grown in plastic pots (38 cm width and 28 cm height) with plugholes during the dry season in November 2011 to March 2012 at the Field Crop Research Station, Khon Kaen University. At the date of 50% blooming (37 DAS; days after sowing), 100-mL spore suspension from fresh A. flavus cultures on potato dextrose agar (PDA) medium was applied entirely the soil surface at 1.75 × 107 spores/cm2 to normalize the infection. The drought stress was started at the beginning pod stage (57 DAS) by stopping water supply a few days in advance from the calculated daily water loss. The apparent soil water status was weekly confirmed by the conventional gravimetric method. The drought continued to the harvest time at the seed maturity stage (110–120 DAS dependent on genotypes).
Plant responses to water status
Samples were taken from the peanut plants at the beginning seed stage (76 DAS). The first fully expanding leaves from the main stem apex (n = 3) were detached at noon. One leaf was processed for determining relative water content from leaf fresh weight, turgid weight, and dry weight.Citation21) The other leaf was immediately dipped in cold absolute ethanol to block wounding stress and shortly kept at −30 °C. On analysis, the leaf was washed in water to remove ethanol, blotted dry, and weighed for the leaf wet weight. The malondialdehyde content in leaves (0.3 g) was quantified by the thiobarbituric acid reaction.Citation22) The aerial parts of peanut plants (n = 3) were dried at 80 °C to a constant weight as the biomass value.
Test for A. flavus seed contamination
Fresh sound mature peanut seeds (n = 10) were surface sterilized by rinse once for 30 s with 70% ethanol and subsequent 5-min shake in 1% sodium hypochlorite. After excessive washings with distilled water, seeds were placed on PDA medium at 30 °C for a week.
Preparation of prolamin samples
The kernel from the fresh sound mature seeds was got rid of seed coats to prevent the effect of phenolic compounds,Citation23) crushed in liquid N2 and defatted overnight at −20 °C with hexane (25 mL/g). The fat-free kernel meal was air-dried and stored at −70 °C.
The preparation of prolamins followed the two-step extraction,Citation17) which was performed by stirring the fat-free sample (10 g) in 5 volumes of a specified solvent for 1 h at room temperature. The first step was twice extraction with 2 M NaCl in 50 mM Tris–HCl (pH 6.8). Then, the meal was rinsed with acetone, air-dried, and subjected to second extraction with 70% ethanol. The ethanolic extract was carried out protein precipitation for 1 h on ice with 4 volumes of cold acetone. The precipitates were collected by centrifugation, flushed with N2 gas, and suspended in 20 mL of distilled water (pH 6.25) in order to obtain homogeneously dispersed powder by lyophilization. The packed dry sample was readily dissolved in water which was cited unusual for prolamins. The freeze-dried powder as crude prolamins was water soluble at the routine preparation of 3 μg protein/μL as determined by the modified Lowry protein assay kit (Pierce, USA).
In an experiment with ICGV 98305 as specified in “Results”, the crude prolamin powder solution was carefully adjusted with 1 M HCl to pH 5.60 and left for 18 h at 4 °C to partially purify particular members of prolamins by means of the isoelectric precipitation. After centrifugation, the purified precipitate was dissolved and the supernatant was collected. Both fractions were concentrated to a protein concentration of 10 μg/μL by centrifugal ultrafiltration using Vivaspin tube (10 kDa MWCO; GE Healthcare, UK) and processed for test on A. flavus and 2D-GE analysis.
Inhibitory assay on fungal spore germination
The aqueous solutions of prolamin powder or those from the isoelectric purification were adjusted for a protein concentration of 3 μg/μL and made sterile by membrane filtration. Spore suspensions were prepared from fresh A. flavus culture. In triplicates using a 96-well microplate, 80-μL suspensions (5 × 105 spores) were treated with 10 μL of the sterile prolamin sample (30 μg protein). After 16 h at 30 °C, glutaraldehyde was added at 5% for fixation and germinated spores were determined as a percentage of totally 300 spores/replicate by counting under a light microscopy.
SDS–PAGE analysis
Samples (10 μg protein) were separated in a 13% gel with a Mini-Protean III unit (Bio-Rad, USA) using the standard SDS–PAGE protocol.Citation24) Protein bands were visible by coomassie brilliant blue R-250.
2D-GE analysis
Isoelectric focusing was performed firstly with the Ettan IPGphore apparatus (GE Healthcare, Sweden). To eliminate the retarding effect by impurities, 1 mL of crude prolamin solution was washed three times in distilled water with fourfold each using the Vivaspin tube. The obtaining cloudy solution was finally adjusted to a total protein concentration of 10 μg/μL. The dry 13-cm immobilized pH gradient strip (pH 3–10 NL; GE Healthcare, Sweden) was incubated for 12 h at 20 °C with 100 μg of the protein sample solubilized in 250 μL of a solution containing 0.5% IPG buffer (GE Healthcare, Sweden), 4% CHAPS (3-[(3-cholamidopropyl)dimethylammonio]-1-propane sulfonate), 8 M urea, 1 M thiourea, 2 μM DTT (dithiothreitol), and trace of bromophenol blue. Electrophoresis was optimized at 0.5 kV for 1 h, 1 kV for 1 h, 3 kV for 2.5 h, and 8 kV for 5 h, continuously, with a constant current of 50 μA/strip. After that, the strip was treated for 20 min at room temperature in 50 mM Tris–HCl buffer (pH 8.8) containing 8 M urea, 1% DTT, 30% glycerol, 2% SDS, and trace of bromophenol blue, which was followed by 20-min incubation in the same buffer with 0.7% iodoacetamide instead of DTT. Then, the strip was rinsed once with distilled water and subjected to 15% gel SDS–PAGE using a Hoefer™ SE 600 Ruby apparatus (Amersham Biosciences, USA). The gel was processed for protein staining by colloidal coomassie brilliant blue G-250. Protein spots were recorded and analyzed by a LabScan ImageScanner-ImageMaster™ 2D Platinum 5.0 software system (GE Healthcare, Sweden).
Results
Plant responses to water status
All six peanut genotypes had the same trends of leaf relative water content (Table ). The lower content without leaf wilting was a general indicator for the drought effect on peanut plants. The vegetative parameters for drought response could be observed from growth in terms of biomass or the leaf content of malondialdehyde (MDA; a molecular index for the oxidative stress effect). The drought impact on Tainan-9 (Spanish-type peanut) and KK 60-3 (Virginia-type peanut) as the drought-sensitive models was demonstrated by both indices. Different results were found in the different resistant genotypes (all Spanish type). Except ICGV 98308, the other ICGV genotypes showed good growth adaptation under drought. Meanwhile, ICGV 98305 and ICGV 98308 exhibited better resistance to the drought-induced oxidative stress than the other four genotypes. Drought resistant characteristics in vegetative modes were therefore genotypic dependent.
Table 1. Peanut responses to the soil water content at control watering and drought. Mean ± S.D. values with the same superscripts are not significantly different by one-way ANOVA analysis at p ≤ 0.05.
With 1-week incubation on PDA medium, seeds of the drought-stressed plants differently exhibited outbreaks of fungal mycelium with A. flavus characteristic yellow–green spores. The lower outbreaks were found in ICGV 98300, 98303, and 98305. It demonstrated high resistance to A. flavus in these genotypes. The more fungal appearance was that of the ICGV 98308 and KK 60-3 while the highest was that of Tainan-9. There was no fungal growth in seeds (n = 10) of all genotypes from the control watering.
There was no conclusive evidence to rank the drought resistance of the peanut genotypes in the study. It was seen from indices of biomass, leaf MDA content, and seed resistance to A. flavus that ICGV 98300 resembled ICGV 98303 in response to drought, whereas it was different from other genotypes in at least one index. As it could not be expected for the correlation of peanut genotypes and the seed prolamin constituents, all including the two resembled genotypes were used for further investigations.
Characterization of crude prolamin fraction
The seed prolamins obtained by alcohol extraction and subsequent acetone precipitation were examined by SDS–PAGE. The profile contained polypeptides of small sizes to almost 66 kDa. Four major bands were unique at 22.4 kDa to below 14.4 kDa (Fig. ). Different from the Spanish-type Tainan-9, the profiles within the Spanish-type ICGV peanuts appeared more closely to those of the Virginia-type KK 60-3. The prolamin profiles from SDS–PAGE could not be distinguishable among the peanut genotypes. From band intensity, there were changes in the content of prolamin polypeptides at 17, 20.1, 22.4, 27.5, 31.6, and 66 kDa in some drought-stressed peanuts. However, these changes did not correlate with peanut characteristics to drought stress.
Fig. 1. SDS–PAGE profiles of crude prolamins from seeds of peanuts grown under the control watering (W) and drought (D) conditions.
Notes: The peanut genotypes from left to right are KK 60-3, Tainan-9, ICGV 98300, ICGV 98303, ICGV 98305, and ICGV 98308.
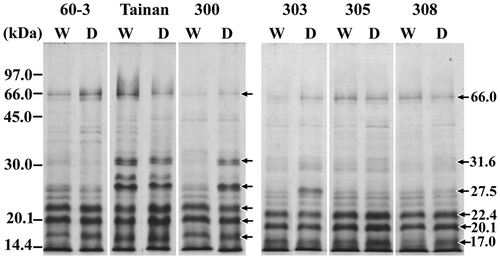
While the A. flavus spore germination in water was 84 ± 1.73%, the KK 60-3 prolamin fractions enhanced spore germination (Table ). Therefore, the KK 60-3 prolamins were used as negative references in the proteomic study for its components in relation to the lack of antifungal activity. The prolamin fractions of all Spanish-type peanuts including Tainan-9 and ICGV genotypes possessed inhibitory activity on A. flavus spore germination which was slightly higher in the samples from drought experiments of Tainan-9, ICGV 98305, and ICGV 98308. The inhibition was lower for the Tainan-9 prolamin samples while it was highest in those of ICGV 98308.
Table 2. Germination of Aspergillus flavus spores affected by peanut prolamins. Crude prolamin fractions were prepared from seeds of different peanut genotypes grown under the control watering and drought conditions. Mean ± S.D. values with the same superscripts are not significantly different by one-way ANOVA analysis at p ≤ 0.05.
Proteomic analysis by 2D-GE
From Fig. , the demonstrative profile by that of Tainan-9 revealed that the crude prolamins contained spots of basic (pI 9.8) to acidic polypeptides (pI 4.5). By software evaluation for the resolving distances along the X-axis (pH) vs. the Y-axis (MW), six dense spots at around pH 5.45–5.75 were detected namely the polypeptide spot 1 (pI 5.75, 30.5 kDa), spot 2 (pI 5.75, 28.5 kDa), spot 3 (pI 5.75, 22 kDa), spot 4 (pI 5.5, 30.5 kDa), spot 5 (pI 5.5, 28.5 kDa), and spot 6 (pI 5.45, 22 kDa). The results in Fig. showed that these six spots were also found commonly in other Spanish-type peanut models (ICGV 98300, ICGV 98303, ICGV 98305, and ICGV 98308). Except for the 22 kDa polypeptides, those around 28.5–30.5 kDa were not clearly detectable by the lower resolution on SDS–PAGE. These spots did not appear in the profiles of the Virginia-type KK 60-3 crude prolamins, but nine spots were found closely at pH 5.05, 5.35, and 5.90. Due to the restricted presence in the Spanish-type peanut models, the spot 1–6 prolamins were prospective for their correlative activity against A. flavus spore germination.
Fig. 2. Demonstrative 2D-GE profile of crude seed prolamins from the Spanish-type Tainan-9 peanut genotype.
Notes: The sample was from seeds of the control watering peanut plants. The arrows point to the six characteristic spots.
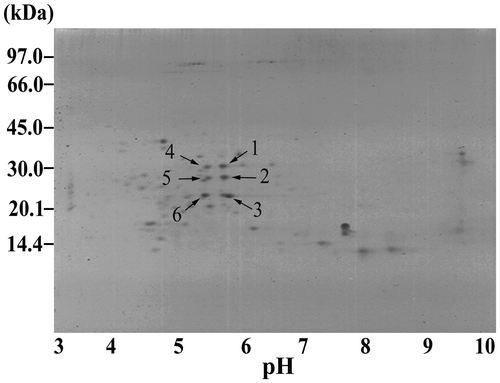
Fig. 3. Intensive 2D-GE profiles of crude seed prolamins zooming-in the common area around characteristic spots in individual genotype.
Notes: The second dimension gels were cropped to focus at the area containing polypeptide spots within the pI range of 4–7 and masses of 14.4–45.0 kDa. The samples were of Tainan-9 (A, B); ICGV 98300 (C, D); ICGV 98303 (E, F); ICGV 98305 (G, H); ICGV 98308 (I, J) and KK 60-3 (K, L). Figures in the left panel are of control watering samples, and those of drought-stressed samples are in the right panel. The spots 1–6 were numbered following the demonstrations in Fig. .
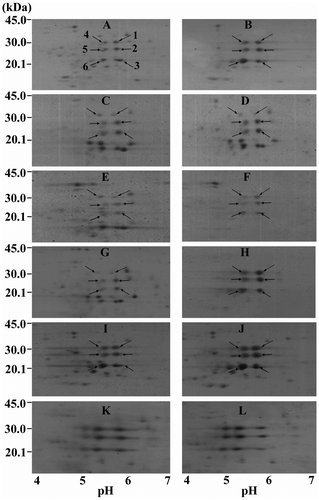
The spots 1–6 in the profile from Fig. were undertaken quantitative 3D view analysis for the peak volumes to represent their comparative contents (Fig. ). The peak volumes and inductive characteristic of spots 1–6 were genotypic dependent. The six spots showed high peak volumes in ICGV 98308, but all were relatively low in Tainan-9 and ICGV 98303. The ICGV 98300 had high volume of spot 5 while ICGV 98305 contained high volumes of spots 2 and 3. The increases in the peak volume were found in most samples from drought treatment except for the unchanged spot 4 in ICGV 98300 and ICGV 98303, as well as the declined level of spot 5 in ICGV 98300 and of spot 6 in ICGV 98303. These data would be discussed with the antifungal activity of the relevant prolamin samples of the Spanish-type peanuts in Table .
Fig. 4. Relative content of the spots 1–6 crude prolamin polypeptides from Tainan-9, ICGV 98300, ICGV 98303, ICGV 98305, and ICGV 98308.
Notes: The data were obtained from the 3D view analysis for peak volumes of the spots 1–6 in Fig. . For each spot, the dark chart stands for the drought-stressed sample which is superimposed on the chart for the control watering sample.
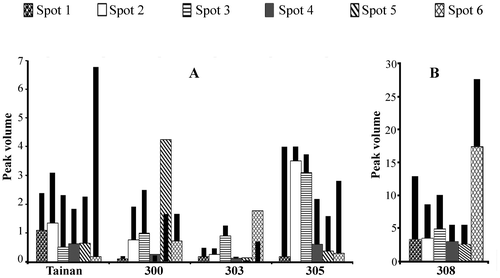
Isoelectric precipitation of the prospective antifungal prolamins
As considered from the results in Fig. , the solution of crude prolamin powder of ICGV 98305 from the drought experiment was chosen for precipitation at pH 5.60 to selectively purify prolamins containing the six prospective polypeptides (pI 5.45–5.75). The yield of the protein precipitate was approximately 6–7% of the original amount in prolamin solution. The 2D-GE profiles were demonstrated for the precipitate or the supernatant portion after precipitation. The precipitate contained immensely nine spots, and six spots among them could be identified as the prospective prolamins with slight shifts in pI and molecular weight (Fig. (A)) including the spot 1 (pI 5.8, 30.5 kDa), spot 2 (pI 5.8, 27.5 kDa), spot 3 (pI 5.8, 22.5 kDa), spot 4 (pI 5.5, 30.5 kDa), spot 5 (pI 5.5, 27.5 kDa), and spot 6 (pI 5.5, 23 kDa). The predominant presence of six spots related prolamins of the ICGV 98305 precipitate sample was concurrent with fivefold increase in inhibitory activity against A. flavus spore germination (10.34 ± 0.47% germination; 30 μg proteins) comparing to that before precipitation (50 ± 0.90%, Table ). More abundant spots were detected in the 2D-GE profile of the supernatant portion (Fig. (B)) including the three enriched spots considerably equivalent to the spot 4 (pI 5.6, 30 kDa), spot 5 (pI 5.6, 27 kDa), and spot 6 (pI 5.6, 22.5 kDa). Together with the change in the prolamin constituents with the loss in the spot 1–3, the supernatant strikingly enhanced A. flavus spore germination to 94.32 ± 0.62%. At this point, the spot 1–3 related peanut seed prolamins were regarded as significant members displaying antifungal activity.
Discussion
The peanut seeds are usually attacked by soil microbes, importantly by A. flavus. The seed has functional and structural defensive mechanisms including a number of the seed storage water-soluble proteins which are the three 2S albumins (Ara h 2, Ara h 6, Ara h 7) and a non-specific lipid transfer protein (Ara h 9).Citation13,16,25,26) Our preliminary work demonstrated the possibility that A. flavus spore germination could be significantly inhibited by the crude 70% ethanol-extractable peanut seed prolamins.Citation17) The investigation on the aqueous alcohol-soluble peanut antifungal prolamin members will provide another biological function of proteins in this group in addition to the known nitrogen storage for seedling growth.
The parameters in the study were water treatments and peanut genotypes to obtain comparative information within and between genotypes. The period of severe drought treatment covered the critical period of water-deficit stress in peanuts.Citation27) High leaf malondialdehyde (MDA) content is an index of drought-induced oxidative damage due to suppression of photosynthesis from stomatal closure to prevent water loss, while metabolic regulation of its production close to the basal level is promising for the drought resistance.Citation5) Although it was not our main point, it was found that the drought resistance in vegetative modes (aerial biomass and/or leaf MDA content; Table ) did not always promise the seed resistance to A. flavus as previously reported.Citation28)
The present data showed inhibitory activity on A. flavus spore germination of crude prolamin fractions from different peanut genotypes (Table ), which could be attributed to their antifungal components. The presence of significant antifungal proteins was suspected in the crude prolamins from the Spanish-type peanut genotypes. The distinct characteristic of Virginia KK 60-3 crude prolamins preliminarily predicted dissimilar components. The antifungal assessment of prolamins in vitro did not relate to the occurrence of fungal growth in seed tests, as in the cases of ICGV 98308. Other defensive mechanisms might be dealt with constitutive or drought-inductive seed components.Citation25,29,30)
In this work, SDS–PAGE was not successful in discriminating prolamin profiles between peanut genotypes, as well as between water statuses (Fig. ). The prolamin profiles were similar to the previous report.Citation17) They could be further cited to be free of the bands of globulins and albumins.Citation31) Therefore, the antifungal activity of the prolamin fractions should not be from 2S albumins. The profiles could not be compared to those of another legume,Citation32) because of species difference or the extraction method. This study provided the first 2D-GE maps for peanut prolamins which were resolved in the pH range of 4.5–9.8 (Fig. ). Although the native prolamins are hydrophobic and extractable by high alcohol concentration, polypeptides with acidic pIs to basic pIs were also published for the prolamins of Inca peanut and rice.Citation32,33) The results revealed six major constitutive polypeptides with sizes of 30.5, 28.5, and 22 kDa at pI 5.45–5.75 restrictedly in seed prolamins of the Spanish-type peanuts (Fig. (A–J)). Their presence was concurrent with the relatively high inhibitory activity on A. flavus spore germination (Table ), which was the first clue for their possible significance in that role. Nevertheless, the KK 60-3 prolamin extract might contain the six target prolamin members in low proportion so that the antifungal measurement and 2D-GE resolution were hindered by other prolamins.
There have been neither established 2D-GE maps nor sequence database of peanut seed prolamins. Several resources were searched for indirect information of any peanut seed proteins having related sizes and pIs. The six polypeptides have not been found in 2D-GE maps of total protein extracts from seeds of the established Spanish, Virginia and Valencia peanuts,Citation8–10) or maps of peanut seed crude globulins.Citation34) Despite water solubility of the seed albumins, a large amount of the peanut albumins could be extracted by NaCl buffer.Citation35) In a soybean 2D-GE model, little contamination of globulins and albumins was evident together with other hydrophilic proteins after one-step extraction by relatively high alcohol strength using 40% isopropanol.Citation36) The authors explained that it was resulted from protein aggregation as aqueous alcohol-soluble molecules. Therefore, we deduced from SDS–PAGE and 2D-GE that excessive extraction by buffered NaCl solution achieved in sufficient elimination of globulins and albumins as well as the antifungal proteins thereof. Thus, it resulted in significant enrichment of prolamins for alcohol extraction in the subsequent step. The spots of the antifungal Ara h 9 (9 kDa and pI around 9–10)Citation37) did not appear in our 2D-GE maps. The antifungal activity of the peanut prolamins was consequently not from the established 2S albumins and Ara h 9. Peanut seed proteins obtained from subsequent extraction with NaCl buffer and low ethanol concentration (20%) gave 2D-GE mapsCitation38) dissimilar to our results.
Prolamins are abundant storage proteins in cereal seeds which vast knowledge has been gathered from. All prolamins are recognized water insoluble in their native conformation in protein bodies within endoplasmic reticulum originated vacuoles, and they are extractable in water/alcohol mixtures.Citation39) Despite predominant content of hydrophobic amino acid residues, prolamins from different cereal seeds have different hydrophilic characteristic.Citation39,40) It was evident that a number of prolamins in wheat, barley, and rye could be directly extracted in water or 0.5 M NaCl at room temperature.Citation41) These prolamins probably exhibit their water solubility once vacuolar free. Peanut seed prolamin powder extracts in this work were ready to dissolve in water. It is likely that 80% acetone could precipitate prolamins having more hydrophilic nature out of the total prolamins soluble in 70% ethanol after extraction. However, their hydrophobic nature was apparent later since the crude proteins turned dramatically water insoluble under the repeatedly successive ultrafiltration in the sample preparation for 2D-GE. The rehydration buffer with a combination effect of urea and thiourea was consequently used as it has been recommended for 2D-GE analysis of hydrophobic proteins.Citation33)
In terms of Ara h, they are groups of peanut seed allergenic proteins of which biochemical properties (MW and pI) as well as biological roles have been reviewed.Citation13,42) The three main allergens are Ara h 1 (7S globulin or conarachin), Ara h 3 (11S globulin or arachin), and Ara h 2 (a 2S albumin with trypsin inhibitor activity against pathogen) comprising about 90% of total allergens.Citation13) These allergens together with another 2S albumin (Ara h 6; a trypsin inhibitor) have been already suggested as protein markers in identification, by SDS–PAGE or 2D-GE, of peanut genotypes at the viewpoint of genotypic markers and nutritional awareness.Citation8–10) Some peanut allergens are pathogenesis-related (PR) proteins including Ara h 8 (ribonuclease-like PR-10) and two defensins (Ara h 12 and Ara h 13). Ara h 9 is the group of non-specific lipid transfer proteins (nsLTPs) and is the last allergen that has activity against pathogens. The 2S albumins and nsLTPs are also alternatively characterized in the same prolamin superfamily due to conserved cysteine residues and intrachain disulfide bridges similar to the seed storage alcohol-soluble prolamin.Citation13,42) Most Ara h is soluble in water or saline solution except defensins which could be differently obtained by methanol/chloroform extraction of peanut seed meal.Citation13) Regarding to solubility property, these allergens are hardly present in the aqueous ethanolic prolamin fraction from the peanut seeds. The inhibitory action on A. flavus spore germination in Table consequently was neither caused by the relevant Ara h nor the novel water-soluble chitinase-like antifungal hypotin.Citation30)
The synthesis of a number of peanut seed functional proteins including defensive proteins could be up-regulated according to water stress, A. flavus infection, or aflatoxin contamination.Citation11,12,43–45) Basically, these proteins are less abundant and natively hydrophilic so that they should not be confound in the prolamin fractions after the two-step extraction used in the study.
Based on the analysis of spot peak volumes, the content of spots 1–6 could draw a primary attention for their possible relevance to the inhibitory activity of the prolamin fraction against A. flavus spore germination (Fig. and Table ). The interesting demonstrations were those from the control experiments of Tainan-9 (all low contents/low inhibition) and ICGV 98308 (all high contents/high inhibition). Meanwhile, the intermediate inhibition was observed in ICGV 98300, 98303, and 98305 which the mixed low or high presence of some prospective spots was remarked. However, the results from drought experiments and partial purification by isoelectric precipitation could be finally conclusive for the significance of the prolamin member spots 1–3, not spots 4–6, as antifungal prolamins inhibiting A. flavus spore germination. The present data did not inform the native conformation of the spot 1 (pI 5.75, 30.5 kDa), spot 2 (pI 5.75, 28.5 kDa), and spot 3 (pI 5.75, 22.0 kDa) whether they are monomeric or homo-/hetero-multimeric. It seemed that isoelectric precipitation at pH 5.60 with low protein yield obtained highly purified spot 1–3 prolamin polypeptides as considered by the comparative 2D-GE map (Fig. ) and the increased antifungal activity of the precipitate. Conclusively, the spot 1–3 related peanut seed prolamin storage proteins were closely associated with the antifungal property against spore germination in the A. flavus model. Their presence was specifically illustrated in the Spanish-type peanuts and was confirmed up on induction in the drought condition. The relative content of the final three spots herein did not always show positive correlation with the drought resistant genotypes. It was high in ICGV 98308 comparing to those in other ICGV genotypes and in the drought-sensitive Tainan-9 (Fig. ). Likewise, their content was not in agreement with resistance to A. flavus infection in seeds of the drought-stressed peanut plants. For example, the fungal infection was high in ICGV 98308 while it was very low in ICGV 98303. The use of drought-sensitive/resistant genotypes together with water-deficit treatment has been the conventional tool to investigate total protein extract for protein markers of the resistance traits to drought and seed aflatoxin contamination in peanuts.Citation12,44) That no correspondence was found between the prolamin content and the resistance traits might be resulted from the fractionated extraction procedure. The present data revealed the existence of prolamins with positive test in inhibiting A. flavus spore germination. If antibody is raised against these prolamins, it will be useful in further comparative detection for their presence in the total protein extract of peanut seeds in relation with the resistance traits for breeding purpose.
Author contribution
W. Senakoon carried out the whole experiments and made manuscript draft; S. Nuchadomrong was responsible for the main experimental assignments, data analysis and manuscript preparation; R.Y.-Y. Chiou advised in 2D-GE part; G. Senawong took part in antifungal tests; S. Jogloy, P. Songsri, and A. Patanothai guided works in peanut cultivation and water treatment.
Disclosure statement
No potential conflict of interest was reported by the authors.
Funding
This work was supported by the Higher Education Research Promotion and National Research University Project of Thailand, Office of the Higher Education Commission, through the Food and Functional Food Research Cluster of Khon Kaen University, Thailand [grant number NRU541007, FC1.1.7 PhD]; and the Thailand Research Fund, the Commission for High Education and Khon Kaen University through the Distinguish Research Professor Grant of Professor Dr. Aran Patanothai.
Acknowledgment
Miss Senakoon is grateful to a scholarship from the Graduate School Khon Kaen University for her short term work at the National Chiayi University, Taiwan.
References
- Pandey MK, Monyo E, Ozias-Akins P, et al. Advances in Arachis genomics for peanut improvement. Biotechnol. Adv. 2012;30:639–651.10.1016/j.biotechadv.2011.11.001
- Canavar Ö, Kaynak MA. Prevention of pre-harvest aflatoxin production and the effect of different harvest times on peanut (Arachis hypogaea L.) fatty acids. Food Addit. Contam. Part A 2013;30:1807–1818.10.1080/19440049.2013.818720
- Dorner JW. Management and prevention of mycotoxins in peanuts. Food Addit. Contam. 2008;25:203–208.10.1080/02652030701658357
- Surendranatha Reddy EC, Sudhakar C, Eswara Reddy NP. Aflatoxin contamination in groundnut induced by Aspergillus flavus type fungi: a critical review. IJABPT 2011;2:180–192.
- Sharma P, Jha AB, Dubey RS, et al. Reactive oxygen species, oxidative damage, and antioxidative defense mechanism in plants under stressful conditions. J. Bot. 2012;2012:1–26.10.1155/2012/217037
- Bianchi-Hall CM, Keys RD, Stalker HT. Use of seed protein profiles to characterize peanut cultivars. Peanut Sci. 1994;21:152–159.10.3146/i0095-3679-21-2-18
- Javaid A, Ghafoor A, Anwar R. Seed storage protein electrophoresis in groundnut for evaluating genetic diversity. Pak. J. Bot. 2004;36:25–29.
- Liang XQ, Luo M, Holbrook CC, et al. Storage protein profiles in Spanish and runner market type peanuts and potential markers. BMC Plant Biol. 2006;6:24–32.10.1186/1471-2229-6-24
- Kottapalli KR, Payton P, Rakwal R, et al. Proteomics analysis of mature seed of four peanut cultivars using two-dimensional gel electrophoresis reveals distinct differential expression of storage, anti-nutritional, and allergenic proteins. Plant Sci. 2008;175:321–329.10.1016/j.plantsci.2008.05.005
- Schmidt H, Gelhaus C, Latendorf T, et al. 2-D DIGE analysis of the proteome of extracts from peanut variants reveals striking differences in major allergen contents. Proteomics 2009;9:3507–3521.10.1002/pmic.v9:13
- Basha SM, Katam R, Naik KSS. Differential response of peanut genotypes to water stress. Peanut Sci. 2007;34:96–104.10.3146/0095-3679(2007)34[96:DROPGT]2.0.CO;2
- Wang T, Zhang E, Chen X, et al. Identification of seed proteins associated with resistance to pre-harvested aflatoxin contamination in peanut (Arachis hypogaea L). BMC Plant Biol. 2010;10:267–277.10.1186/1471-2229-10-267
- Bublin M, Breiteneder H. Cross-reactivity of peanut allergens. Curr. Allergy Asthma Rep. 2014;14:426–437.10.1007/s11882-014-0426-8
- Sebei K, Gnouma A, Herchi W, et al. Lipids, proteins, phenolic composition, antioxidant and antibacterial activities of seeds of peanuts (Arachis hypogaea L.) cultivated in Tunisia. Biol. Res. 2013;46:257–263.10.4067/S0716-97602013000300006
- Shewry PR, Tatham AS. The prolamin storage proteins of cereal seeds: Structure and evolution. Biochem. J. 1990;267:1–12.
- Shewry PR, Halford NG. Cereal seed storage proteins: Structures, properties and role in grain utilization. J. Exp. Bot. 2002;53:947–958.10.1093/jexbot/53.370.947
- Senakoon W, Nuchadomrong S, Senawong G, et al. An alternate fractionation of peanut seed proteins in association with inhibitory assay on Aspergillus flavus. APCBEE Procedia 2012;4:42–47.10.1016/j.apcbee.2012.11.008
- Girdthai T, Jogloy S, Vorasoot N, et al. Associations between physiological traits for drought tolerance and aflatoxin contamination in peanut genotypes under terminal drought. Plant Breed. 2010;129:693–699.10.1111/j.1439-0523.2009.01738.x
- Songsri P, Jogloy S, Holbrook CC, et al. Association of root, specific leaf area and SPAD chlorophyll meter reading to water use efficiency of peanut under different available soil water. Agric. Water Manage. 2009;96:790–798.10.1016/j.agwat.2008.10.009
- Boote KJ. Growth stages of peanut (Arachis hypogaea L.). Peanut Sci. 1982;9:35–40.10.3146/i0095-3679-9-1-11
- Sharp RE, Hsiao TC, Silk WK. Growth of the maize primary root at low water potentials: II. Role of growth and deposition of hexose and potassium in osmotic adjustment. Plant Physiol. 1990;93:1337–1346.10.1104/pp.93.4.1337
- Sheokand S, Bhankar V, Sawhney V. Ameliorative effect of exogenous nitric oxide on oxidative metabolism in NaCl treated chickpea plants. Braz. J. Plant Physiol. 2010;22:81–90.
- White BL, Gökce E, Nepomuceno AI, et al. Comparative proteomic analysis and IgE binding properties of peanut seed and testa (Skin). J. Agric. Food. Chem. 2013;61:3957–3968.10.1021/jf400184y
- Laemmli UK. Cleavage of structural proteins during the assembly of the head of bacteriophage T4. Nature 1970;227:680–685.10.1038/227680a0
- Liang XQ, Luo M, Guo BZ. Resistance mechanisms to Aspergillus flavus infection and aflatoxin contamination in peanut (Arachis hypogaea). Plant Pathol. J. 2006;5:115–124.
- Breiteneder H, Radauer C. A classification of plant food allergens. J. Allergy Clin. Immunol. 2004;113:821–830.10.1016/j.jaci.2004.01.779
- Suleiman AA, Soler CMT, Hoogenboom G. Determining FAO-56 crop coefficients for peanut under different water stress levels. Irrigation Sci. 2013;31:169–178.10.1007/s00271-011-0301-5
- Hamidou F, Rathore A, Waliyar F, et al. Although drought intensity increases aflatoxin contamination, drought tolerance does not lead to less aflatoxin contamination. Field Crops Res. 2014;156:103–110.10.1016/j.fcr.2013.10.019
- Ye XY, Ng TB. Hypogin, a novel antifungal peptide from peanuts with sequence similarity to peanut allergen. J. Pept. Res. 2001;57:330–336.10.1046/j.1397-002X.2001.00874.x
- Wang S, Shao B, Rao P, et al. Hypotin, a novel antipathogenic and antiproliferative protein from peanuts with a sequence similar to those of chitinase precursors. J. Agric. Food Chem. 2007;55:9792–9799.10.1021/jf071540j
- El-Beltagi HES. Effect of roasting treatments on protein fraction profiles, some enzyme activities of Egyptian peanuts. Int. J. Food Sci. Nutr. 2011;62:453–456.10.3109/09637486.2010.544642
- Sathe SK, Kshirsagar HH, Sharma GM. Solubilization, fractionation, and electrophoretic characterization of inca peanut (Plukenetia volubilis L.) proteins. Plant Food Hum. Nutr. 2012;67:247–255.10.1007/s11130-012-0301-5
- Shigemitsu T, Saito Y, Morita S, et al. Separation and identification of rice prolamins by two-dimensional gel electrophoresis and amino acid sequencing. Biosci. Biotechnol. Biochem. 2012;76:594–597.10.1271/bbb.110791
- Chassaigne H, Trégoat V, Nørgaard JV, et al. Resolution and identification of major peanut allergens using a combination of fluorescence two-dimensional differential gel electrophoresis, Western blotting and Q-TOF mass spectrometry. J. Proteomics 2009;72:511–526.10.1016/j.jprot.2009.02.002
- Porterfield HS, Murray KS, Schlichting DG, et al. Effector activity of peanut allergies: a critical role for Ara h 2, Ara h 6, and their variants. Clin. Exp. Allergy 2010;39:1099–1108.
- Natarajan SS, Krishnan HB, Lakshman S, et al. An efficient extraction method to enhance analysis of low abundant proteins from soybean seed. Anal. Biochem. 2009;394:259–268.10.1016/j.ab.2009.07.048
- Krause S, Reese G, Randow S, et al. Lipid transfer protein (Ara h 9) as a new peanut allergen relevant for a Mediterranean allergic population. J. Allergy Clin. Immunol. 2009;124:771–778.e5.10.1016/j.jaci.2009.06.008
- Chassaigne H, Brohée M, Nørgaard JV, et al. Investigation on sequential extraction of peanut allergens for subsequent analysis by ELISA and 2D gel electrophoresis. Food Chem. 2007;105:1671–1681.10.1016/j.foodchem.2007.04.055
- Taylor J, Anyango JO, Taylor JRN. Developments in the science of zein, kafirin, and gluten protein bioplastic materials. Cereal Chem. 2013;90:344–357.10.1094/CCHEM-12-12-0165-IA
- Belitz HD, Kieffer R, Seilmeier W, et al. Structure and function of gluten proteins. Cereal Chem. 1986;63:336–341.
- Kanerva P, Sontag-Strohm T, Brinck O, et al. Improved extraction of prolamins for gluten detection in processed foods. Agric. Food Sci. 2011;20:206–216.10.2137/145960611797471525
- Offermann LR, Perdue ML, He JZ, et al. Structural biology of peanut allergens. J. Contemp. Immunol. 2015;2:1–26.
- Mazhar H, Basha SM. Effects of desiccation on peanut (Arachis hypogaea L.) seed protein composition. Environ. Exp. Bot. 2002;47:67–75.10.1016/S0098-8472(01)00110-1
- Katam R, Vasanthaiah HKN, Basha SM. Proteomic approach to screen peanut genotypes with enhanced nutritional qualities. FBIT 2007;94:171–176.
- Wang Z, Yan S, Liu C, et al. Proteomic analysis reveals an aflatoxin-triggered immune response in cotyledons of Arachis hypogaea infected with Aspergillus flavus. J. Proteome Res. 2012;11:2739–2753.10.1021/pr201105d