Abstract
A feruloyl esterase catalyzes the hydrolysis of the 4-hydroxy-3-methoxycinnamoyl (feruloyl) group from esterified sugars in plant cell walls. Talaromyces cellulolyticus is a high cellulolytic-enzyme producing fungus. However, there is no report for feruloyl esterase activity of T. cellulolyticus. Analysis of the genome database of T. cellulolyticus identified a gene encoding a putative feruloyl esterase B. The recombinant enzyme was prepared using a T. cellulolyticus homologous expression system and characterized. The purified enzyme exhibited hydrolytic activity toward p-nitrophenyl acetate, p-nitrophenyl trans-ferulate, methyl ferulate, rice husk, and bagasse. HPLC assays showed that the enzyme released ferulic acid and p-coumaric acid from hydrothermal-treated rice husk and bagasse. Trichoderma sp. is well-known high cellulolytic-enzyme producing fungus useful for the lignocellulosic biomass saccharification. Interestingly, no feruloyl esterase has been reported from Trichoderma sp. The results show that this enzyme is expected to be industrially useful for biomass saccharification.
Graphical Abstract
HPLC assays showed that TcFaeB released ferulic acid from hydrothermal-treated rice husk, but less ferulic acid than p-coumaric acid from hydrothermal-treated bagasse.
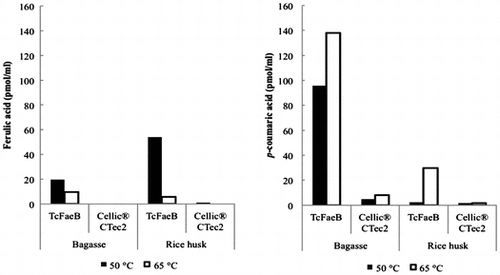
Lignin is well-known to bind tightly with hemicellulose and crystalline cellulose in lignocellulosic biomass.Citation1,2) Hemicellulose (mostly arabinoxylan) consists of ß-1,4-linked chains in which the xylose moieties are periodically replaced by arabinose, ferulic acid, p-coumaric, and acetyl ester groups.Citation3) Phenolic acids such as ferulic and p-coumaric acids are abundant in lignin and hemicellulose and are necessary to provide rigidity to the plant cell wall.Citation4–6) Recently, research into the production of biofuels, chemicals, and composite materials from lignocellulosic biomass has accelerated. Over the past few decades, many researchers have tried to remove these lignocellulosic materials by physical, chemical, and biological methods,Citation7,8) as the removal of lignin from lignocellulosic biomass would allow the facile degradation of biomass to fermentable sugars by the major cellulases such as cellobiohydrolases, endo-glucanases, and ß-glucosidases.Citation9) One of the accessory cellulases, ferulic acid esterase (FAEs, feruloyl esterases, cinnamic acid, or cinnamoyl esterases; EC 3.1.1.73 http://www.cazy.org/Carbohydrate-Esterases.html)Citation10) is known to cleave the ester bond between ferulic acid and polysaccharides in plant cell walls. Ferulates (as well as p-coumarates) are linked to cell wall polysaccharides through ester bonds (1→5 to arabinofuranose residues in heteroxylan-rich bagasse, rice husk, and wheat, and 1→2 to arabionofuranose residues in pectins, such as in sugar beet).Citation11) However, the esterolytic activity of FAE toward natural lignocellulosic biomass remains poorly studied.
The first fungal feruloyl esterase with a carbohydrate-binding module (CBM) from Penicillium funiculosum was reported by Kroon et al.Citation12) At first, this enzyme was exhibited the presence of types A and B FAEs; these two proteins are nearly identical by amino acid homology. Types A and B FAEs should show similar functional behavior since both catalytic domains are highly conserved.Citation12) The localization of fungal CBM-1 is different: Type A is on the N-terminal side and type B is on the C-terminal side of the protein, and their thermostabilities differ, with type B being slightly more stable than type A.Citation13) Then, FAE has been classified into four types (types A–D) based on differences in substrate specificity (preferential hydrolysis of methyl p-coumarate, methyl caffeate, methyl ferulate, or methyl sinapate) and effectiveness in releasing diferulic acid.Citation14,15) Talaromyces cellulolyticus (formerly known as Acremonium cellulolyticus) is a high cellulolytic-enzyme producing fungus first isolated by Yamanobe et al.Citation16) There is no report for FAE activity of T. cellulolyticus. We identified the feruloyl esterase B homologous gene (faeB) by searching the draft genome sequence of T. cellulolyticus.Citation17) Here, we report the cloning, expression, and purification of the recombinant FaeB from T. cellulolyticus (TcFaeB) and its characterization.
Materials and methods
Materials
Methyl ferulate (MFA) and p-nitrophenyl acetate (pNA) were purchased from Wako Pure Chemical Industries (Osaka, Japan). p-Nitrophenyl trans-ferulate (pNF) was purchased from Funakoshi Co., Ltd. (Tokyo, Japan). Cellic® CTec2 was purchased by Novozymes (Bagsvaerd, Denmark). All other chemicals were commercially available.
Strain and culture conditions
The T. cellulolyticus YP-4 uracil autotroph from Y-94 strain (CBS136886) was maintained on potato dextrose agar plates containing uracil and uridine at final concentrations of 1 g/L each.Citation18) Transformants of T. cellulolyticus YP-4 were maintained on minimum medium agar plates.Citation19)
Construction of the recombinant enzyme
A draft genome sequence of T. cellulolyticusCitation17) was searched for the feruloyl esterase gene (faeB) using Molecular Cloning gene analysis software (In Silico Biology, Inc., Yokohama, Japan) based on the internal amino acid sequence. Construction of the expression vector for T. cellulolyticus basically followed a procedure described previously.Citation18) The genomic region encoding the putative faeB was amplified by PCR from Y-94 chromosomal DNA using the following primers: 5′-ATGGCGATTCCCCTGGTCCTTGTTCT-3′ and 3′-AATCCTGCAGGTCACAGACACTGGGAGTAA-5′. The faeB expression plasmid pANC249 was constructed by introducing the amplified gene fragment containing the signal sequence digested with HpaI/SbfI into the EcoRV/SbfI site of a series of pANC249 expression vectors containing a glucoamylase (glaA) promoter and terminator.Citation18) The nucleotide sequences of the gene from YP-4 will appear in the GenBank/EMBL/DDBJ nucleotide database under accession number AB937136.
Enzyme preparation
Protoplasts of T. cellulolyticus YP-4 were transformed with pANC249 by nonhomologous integration into the host chromosomal DNA.Citation19) Gene integration into prototrophic transformants (strain Y249) was verified by genomic PCR. Expression of the recombinant enzyme was conducted as follows: T. cellulolyticus Y249 culture was grown in medium containing 20 g/L soluble starch and 5 g/L urea as described previously.Citation20) For a large-scale expression of the enzyme, the strain was cultivated in 100 ml of a starch-induced medium (24 g/L of KH2PO4, 1 g/L of Tween 80, 5 g/L of (NH4)2SO4, 1.2 g/L of MgSO4·7H2O, 0.01 g/L of ZnSO4·7H2O, 0.01 g/L of MnSO4·6H2O, and 0.01 g/L of CuSO4·7H2O; pH 4.0) supplemented with 20 g/L soluble starch (Wako Pure Chemical Industries) as a carbon source in 500-mL Erlenmeyer flasks at 30 °C for 96 h on a rotary shaker operated at 230 rpm. The cells were centrifuged for 15 min at 2,380×g, and then, the supernatants were centrifuged for 10 min at 20,180×g. The pooled enzyme solution was filtered through a 0.45 μM membrane and dialysis with 20 mM Tris–HCl pH 8.0 and 50 mM NaCl buffer using a Vivaspin 20 concentrator 10,000 MWCO (Sartorius AG, Goettingen, Germany) and stored at 4 °C until use.
The expressed recombinant enzyme was purified from the culture supernatant. Protein purification was carried out essentially as described previously.Citation20) Supernatant was applied to a HiTrap Q HP column (GE Healthcare, Little Chalfont, UK) equilibrated with 50 mM Tris–HCl pH 8.5, and eluted with an ascending salt gradient. The target protein eluted from the column at approximately 200 mM NaCl. Fractions containing the protein was pooled and dialysis into 20 mM Tris pH 8.0, 2 M NaCl and applied to a HiTrap Butyl HP column (GE Healthcare) equilibrated in the same buffer. Protein was eluted with a descending salt gradient. The protein was eluted at approximately 0 M NaCl. As a final step, the eluted protein was added directly to a HiLoad 26/60 Superdex 200 pg column (GE Healthcare) equilibrated with 20 mM Tris–HCl pH 8.0, 50 mM NaCl. The purity of the collected protein from this final step was confirmed by SDS-PAGE and also calculated from the specific activity toward pNF.
Protein assay
The concentration of enzyme was determined using the BCA methodCitation21) with bovine serum albumin as a standard. Protein solution (25 μL) was mixed with 200 μL of BCA reagent and then incubated at 37 °C for 30 min. Protein concentrations were determined by measuring the absorption at 570 nm.
Enzymatic activity assay
The activity of FAE was evaluated as reported previously,Citation22,23) with MFA, pNF, and pNA. As concerns the pNF and pNA assays, each experiment was performed under various buffer conditions from pH 3.0 to 11.0. Thermostability of the enzyme was also measured from 30 to 75 °C (at 5 °C intervals) for 10 min in MES buffer pH 6.0: appropriate dilutions of the protein solution (5 μM, 50 μL) were mixed with 0.45 mL of pNA and pNF (each 5 mM), followed by incubation for 10 min at 50 °C. The amount of the released p-nitrophenol was determined as the enzymatic activity by measuring the absorption at 405 nm. One unit of the activity was defined as the quantity of enzyme required to liberate 1 μmol of p-nitrophenol equivalent per minute at 50 °C.
On the other hand, ferulic acid released from MFA as the substrate was quantified by measuring its absorption at 340 nm. One unit of the activity was defined as the quantity of enzyme required to liberate 1 μmol of ferulic acid equivalent per minute at 50 °C.
The initial rates of reaction of the enzyme (5 μM) were measured with each substrate concentrations ranging from 0.1 to 5.0 mg/mL. The Michaelis constant (Km) and the maximum rate of the activity (Vmax) were determined by nonlinear regression analysis.Citation24)
HPLC assays for biomass hydrolysis products
The esterolytic activities of the enzyme toward pretreated bagasse and rice husk were determined from the concentration of free ferulic acid measured by HPLC using ZORBAX Eclipse PAH column (Agilent Technologies, CA, USA). Catalysis by TcFaeB was assayed with 5% (w/v) bagasse or rice husk (each pretreated at 180 °C, 10 min) in 0.1 M citric acid buffer (pH 4.75). Enzyme were mixed with 5% (w/v) bagasse or rice husk (wet biomass: 5 mg enzyme/g biomass) and incubated at 120 rpm for 24 h at 50 or 65 °C using a reciprocal shaking incubator. The glycated supernatants from the reaction mixes were collected by centrifugation filtration. The eluted free ferulic acid, p-coumaric acid, and acetic acid were measured at 280 nm by HPLC analysis. Cellic® CTec2 was used a control enzyme in a series of assays.
Differential scanning calorimetry
The thermostability of the recombinant enzyme was examined using Differential scanning calorimetry (DSC). Protein sample (1.0 mg/mL) in 50 mM sodium acetate pH 5.5 was used for DSC experiments. A nanoDSC (TA Instruments, New Castle, DE, USA) was used, and the scanning speed was 1.0 °C per min. Control runs of buffer (no protein) were run before and after each sample run. DSC scans on protein were performed on two or three fresh samples of each protein.
Results
Sequence alignments of the enzyme with other fungal feruloyl esterases
We identified one putative feaB gene in the draft genome sequence of T. cellulolyticusCitation17) that exhibits some homology with carbohydrate esterases (EC: 3.1.1.73) using In silico Molecular Cloning gene analysis software (In Silico Biology, Inc., Yokohama, Japan) based on the internal amino acid sequence. The gene contains an open reading frame of 1071 bp and encodes a protein with molecular mass of 37,689 Da. Sequence analysis of the gene identified a signal sequence, the catalytic domain of a serine esterase, linker domain, and CBM. The sequence alignment of the T. cellulolyticus feruloyl esterases B (TcFaeB) and other feruloyl esterases is shown in Fig. . The catalytic domain contains a typical serine esterase motif (the SGNH motif: G(134)-X-S(136)-X-G(138)) and the conserved catalytic triad (Ser136, Asp220, and His276) (shown as green stars in Fig. ). The serine esterases are widely distributed, for example, as microbial acetyl xylan esterases,Citation25) poly (3-hydroxybutyrate) depolymerases,Citation26) and lipases.Citation27) A FaeB from Aspergillus niger (AnFaeB) has been characterized functionally.Citation28,29) Sequence analysis of the enzyme and TcFaeB showed homology with 8% identity, including the conserved catalytic triad and a G-X-S-X-G motif typical of serine esterases (Fig. ). As regards AnFaeB and tannase from Aspergillus oryzae (AoTan), two catalytic and conserved residues of Asp and His are not identified from the sequence analysis (Fig. ).
Fig. 1. The sequence alignment between T. cellulolyticus FaeB and other fungal FAEs.
Notes: Strictly conserved residues are highlighted with a red background and similarly conserved residues are also highlighted by red characters with blue boxes. The putative signal sequence of TcFaeB is shown as a black underline. The catalytic triad (Ser, Asp, and His) is conserved (marked green stars) (excluding AnFaeB and AoTan). The putative CBM-1 of TcFaeB and TfFaeB is highlighted as yellow. The species origin of each enzyme is shown by an abbreviation as follows: TcFaeB: T. cellulolyticus FaeB (GenBank Accession Number AB937136); TfFaeB: Talaromyces (Penicillium) funiculosus (GenBank Accession Number Q9HE18); AoFaeB: Aspergillus oryzae FaeB (GenBank Accession Number 3WMT_A); AoFaeC: Aspergillus oryzae FaeC (GenBank Accession Number XP_001819091); AnFaeB: Aspergillus niger FaeB (GenBank Accession Number CAC83933); NcFaeB: Neurospora crassa OR74A FaeB (GenBank Accession Number XP_963215); MoFaeB: Magnaporthe oryzae Y34 FaeB (GenBank Accession Number ELQ34391); AoTan: Aspergillus oryzae tannase (GenBank Accession Number BAA09656). The figure was made using ClustalWCitation36) and ESPriptCitation37).
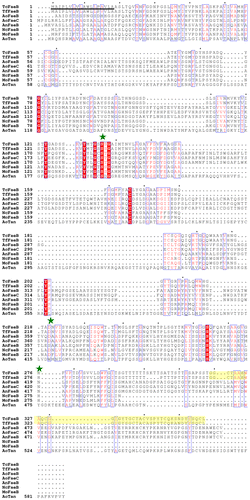
Expression and purification of the recombinant enzyme
The recombinant enzyme was prepared and purified as described in Materials and methods. High amount of the recombinant enzyme prepared (Table ) suggests that the expression and secretion by the speculative signal sequence using the homologous expression system are well done.Citation18) The protein was purified about 7-fold, as judged by its specific activity (Table ). By SDS-PAGE analysis, a single smeared band was observed and the estimated molecular mass of the recombinant enzyme is slightly larger than that calculated from the sequence (Fig. S1). The result of the gel filtration chromatography using HiLoad 26/60 Superdex 200 pg column indicates that the enzyme exhibits the monomer structure.
Table 1. Purification of TcFaeB from T. cellulolyticus Y249.
Characterization of the enzyme
Hydrolytic activities of the purified enzyme toward pNA, Pnf, and MFA were detected. The activities of the enzyme toward pNA, pNF, and MFA were examined in 50 mM Mes-NaOH buffer (pH 6.0) for 10 min at 50 °C. Table shows the specific activities and kinetic parameters of the enzyme toward pNA, pNF, and MFA. The optimum pH and temperature of the enzyme were estimated to be pH 4.5–6.5 and 65 °C, respectively. From these results, this enzyme was identified as one of the carbohydrate esterase (CE).
Table 2. Kinetic parameters of TcFaeB.
The activity of the enzyme toward pretreated biomass
The activity of the enzyme toward biomass was examined using pretreated bagasse and rice husk. Biomass was incubated with the enzyme at 50 °C, pH 4.75, and the released ferulic and p-coumaric acids were detected by HPLC analysis (Fig. (A) and (B)). The enzyme released more p-coumaric acid than ferulic acid from bagasse, but released more ferulic acid than p-coumaric acid from rice husk (Fig. (A) and (B)). Cellic® CTec2 containing no activity of feruloyl esterase is a commercially available enzyme from Novozymes, used as a control. From the above results, the enzyme was identified as feruloyl esterase. We also examined the effects of the temperature. TcFaeB showed significantly reduced ferulic acid production from both biomasses at 65 °C (Fig. (A)) whereas p-coumaric acid production was increased at least 1.5-fold from bagasse and 12-fold from rice husk compared to 50 °C (Fig. (B)). The difference of the activity by the temperature (Fig. ) seems to be derived from the different solubility of the products and/or the local environment in the biomass substrate. Free acetic acid was not detected in any of the reaction mixes.
Fig. 2. The temperature-dependent activity of TcFaeB toward pretreated bagasse and rice husk.
Notes: (A) shows the free ferulic acid produced from bagasse (left) and rice husk (right), and (B) shows the free p-coumaric acid produced from bagasse (left) and rice husk (right). The black bars show data collected at 50 °C and the white bars show data collected at 65 °C. All free concentrations are shown as pmol/mL. These experiments are carried out only once (n = 1). The uncertainty limit for the HPLC analysis was ±10%.
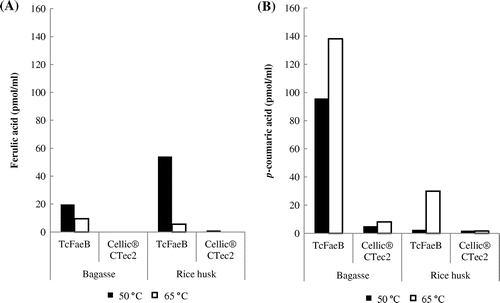
Thermostability assay of the enzyme
The thermostability of TcFaeB (1.0 mg/mL) was examined using a nano DSC in 50 mM sodium acetate pH 5.5 and showed the melting temperature of TcFae at around 70 °C (Fig. ). Precipitate of the enzyme was observed after the heating process (25–125 °C) by DSC, and the reversibility for the unfolding process was not observed. The result that the optimum temperature of the enzyme was estimated to be 65 °C at pH 4.5–6.5 and the above melting temperature indicate that this enzyme can be applicable for biomass saccharification process.
Discussion
We described the cloning of the putative faeB gene from T. cellulolyticus and the expression and purification of its recombinant enzyme. Characterization of the recombinant enzyme showed it to be FaeB from T. cellulolyticus (TcFaeB). TcFaeB comprises 357 amino acids and has a molecular mass of 36 kDa. The enzyme is a member of CE family 1, which contains a Ser–Asp–His catalytic triad, and a fungal CBM-1 at the C-terminus. This report characterized the enzymatic activity of one such enzyme, TcFaeB, using synthetic and natural substrates. Amino acid sequence homology showed that TcFaeB is very similar to other fungal type B feruloyl esterases, including the conserved active residues (Ser, Asp, His) in the catalytic domain involved in substrate recognition and catalysis and in the CBM. The CBM mediates binding to complex polymeric substrates; for example, ferulates and p-coumarates, and arabionofuranose residues linked through 1–5 esters bonds in heteroxylans in bagasse.Citation11) CBM-containing enzymes specifically hydrolyze the esters of hydroxycinnamic acids such as ferulates and p-coumarates. Only T. cellulolyticus and T. funiculosus have the fungal CBM-1 (Fig. ). Fungal CBMs have been classified into a number of familiesCitation10); of these, the fungal CBM-1 possessing proteins have highly conserved sequences 33–40 residues in length. In addition, four aromatic residues are completely conserved and play an important role in binding the targeted celluloseCitation30) and four strictly conserved cysteines form two essential disulfide bonds, enabling the CBM-1 to function normally (Fig. ).Citation31) The CBM-1 and the catalytic domain are separated by an unstructured linker comprising a 20-residue sequence rich in Ser, Thr, and Pro. This linker is believed necessary for correctly binding the CBM to cellulose.
TcFaeB has a tightly conserved catalytic triad consisting of a Ser, Asp, and His residue in the active site (Fig. ). TcFaeB exhibits about 50% sequence identity with other fungal FAE type B proteins, but no crystal structure of a type B FAE is available. Furthermore, the relationship between structure and function remains unclear. Recently, a feruloyl esterase B from Aspergillus oryzae (AoFaeB) was purified and characterized functionally and structurally.Citation32) TcFaeB exhibits low amino acid sequence identity to AoFaeB (around 10%; Fig. ). The ESTHER databaseCitation33) ranks AoFaeB as a member of the fungal tannase family; both are type B enzymes, with tannase, paraben esterase and lipolytic esterase as active forms, whereas TfFae (FAE type B) and TcFaeB are ranked in Esterase_phb. The family of Esterase_phb contains two species of depolymerases for poly(3-hydroxybutyrate) (PHB) and related polyhydroxyalkanoates, as well as acetyl xylan esterases, and feruloyl esterases from fungi.Citation32) PHB is produced from the hydrolysis of [(R)-3-hydroxybutanoic acid]n by PHB depolymerase (EC 3.1.1.75). The catalytic mechanism of the PHB depolymerase has been clarified from the crystal structure of PHB depolymerase from Penicillium (Talaromyces) funiculosum (TfPhb).Citation26) This enzyme reaction also occurs with esters of other short-chain-length (C(1)-C(5)) hydroxyalkanoic acids (HA). TcFaeB and TfPhb share quite low amino acid sequence homology, although the Ser–Asp–His catalytic triad is conserved.
The homologous expression and purification of TcFaeB involved overexpression using pANC expression vectors containing a glaA promoter and terminator.Citation18) The results that the purified TcFaeB exhibits a smeared single band by SDS-PAGE (Fig. S1) and its molecular mass is slightly larger than that calculated from the sequence suggest the glycosylation modification of the Ser-/Thr-rich linker region in the enzyme. Analysis of the linker region by CBS Prediction Servers (http://www.cbs.dtu.dk/services/) suggests it is modified by O-linked glycosylation. It has been reported that the glycosylated Ser-/Thr-rich linker region enhances the binding affinity toward biomass substrate in the cellulase-related enzymes.Citation34)
There are no reports regarding the optimum pH and temperature, and substrate specificity for FaeB derived from Penicillium (Talaromyces) funiculosum, which has 95% identity with TcFaeB. A comparison of the specific activity of TcFaeB for pNF and pNA revealed that pNF is hydrolyzed about 1.8 times faster than pNA. This shows that substrates with aromatic ring side chains support higher activity than acetyl bases due to the substrate specificity of TcFaeB, although TcFaeB exhibited high activity toward pNA.
Thermal stability measurement using DSC showed that TcFaeB has a Tm value of 70 °C. From the analysis of the observed peak of heat capacity, calorimetric enthalpy (dHcal) and van’t Hoff enthalpy (dHvH) were obtained to be 850 and 540 kJ/mol, respectively. The value of dHvH was calculated by the equation for assuming the two-state model, dHvH = 4RTdCitation2Cp(Td)/ dHcal where R is the gas constant, Td is the denaturation temperature (70.3 °C), and Cp(Td) is the peak height (120 kJ/(K mol) at Td. If the transition is two-state, then dHcal = dHvH.Citation35) The value of the ratio dHvH/dHcal (=0.64) lower that unity indicates that the denaturation process is not simple two-state transition model N ↔ D, where N and D are native and unfold state of protein. The above result supports that TcFaeB consists of two domains (catalytic and CBM-1 domain) and the two domains are unfolded individually by heating.Citation35)
The activity of TcFaeB toward pretreated biomass was assayed. Interestingly, TcFaeB released more p-coumaric acid than ferulic acid using bagasse as the substrate (Fig. (A) and (B)). p-Coumaric acid is produced by removing an o-methoxy group from ferulic acid.
We had thought that the similar chemical structures of p-coumaric acid and ferulic acid would result in recognized and comparable catalysis by TcFaeB. TcFaeB seems to exhibit a wide substrate specificity toward similar compounds such as ferulic acid in natural biomass. The structural information of FaeB complexed with p-coumaric acid (or ferulic acid) will clarify the detailed substrate specificity of the enzyme. The activity for tannase of the enzyme was not examined.
Finally, TcFaeB releases both ferulic and p-coumaric acids from natural lignocellulosic biomass. We conclude that TcFaeB can cleave the ester bond of ferulic and p-coumaric acids and thus possesses great potential for efficient lignocellulosic biomass saccharification.
Author contribution
M.W. and K.I. designed research; M.W., E.Y., and H.F. performed research; M.W., E.Y., H.F., H.I., M.T., and K.I. analyzed data; M.W. and K.I. wrote the manuscript.
Supplemental material
The supplementary material for this paper is available online at http://dx.doi.org/10.1080/09168451.2015.1058700.
Disclosure statement
No potential conflict of interest was reported by the authors.
Supplemental_Figure.doc
Download MS Word (1.5 MB)Acknowledgments
We would like to thank Dr Tatsuya Fujii of our member at National Institute of Advanced Industrial Science and Technology for his kind suggestions. The differential scanning calorimetry experiments were conducted by Dr Harumi Fukada of the Graduate School of Life and Environmental Sciences, Osaka Prefecture University.
Notes
Abbreviations: TcFaeB, feruloyl esterase B from Talaromyces cellulolyticus; pNA, p-nitrophenyl acetate; pNF, p-nitrophenyl trans-ferulate; MFA, methyl ferulate.
References
- van den Brink J, de Vries RP. Fungal enzyme sets for plant polysaccharide degradation. Appl. Microbiol. Biotechnol. 2011; 91:1477–1492.10.1007/s00253-011-3473-2
- Zeng Y, Zhao S, Yang S, Ding SY. Lignin plays a negative role in the biochemical process for producing lignocellulosic biofuels. Curr. Opin. Biotechnol. 2014;27:38–45.10.1016/j.copbio.2013.09.008
- Christov LP, Prior BA. Esterases of xylan-degrading microorganisms: production, properties, and significance. Enzyme Microb. Technol. 1993;15:460–475.10.1016/0141-0229(93)90078-G
- Anderson WF, Akin DE. Structural and chemical properties of grass lignocelluloses related to conversion for biofuels. J. Ind. Microbiol. Biotechnol. 2008;35:355–366.10.1007/s10295-007-0291-8
- Deutschmann R, Dekker RF. From plant biomass to bio-based chemicals: latest developments in xylan research. Biotechnol. Adv. 2012;30:1627–1640.10.1016/j.biotechadv.2012.07.001
- Kumar R, Singh S, Singh OV. Bioconversion of lignocellulosic biomass: biochemical and molecular perspectives. J. Ind. Microbiol. Biotechnol. 2008;35:377–391.10.1007/s10295-008-0327-8
- Damásio AR, Braga CM, Brenelli LB, et al. Biomass-to-bio-products application of feruloyl esterase from Aspergillus clavatus. Appl. Microbiol. Biotechnol. 2013;97:6759–6767.10.1007/s00253-012-4548-4
- Wong DW. Feruloyl esterase: a key enzyme in biomass degradation. Appl. Biochem. Biotechnol. 2006;133:87–112.10.1385/ABAB:133:2
- Abokitse K, Wu M, Bergeron H, Grosse S, Lau PC. Thermostable feruloyl esterase for the bioproduction of ferulic acid from triticale bran. Appl. Microbiol. Biotechnol. 2010;87:195–203.10.1007/s00253-010-2441-6
- Cantarel BL, Coutinho PM, Rancurel C, Bernard T, Lombard V, Henrissat B. The Carbohydrate-Active EnZymes database (CAZy): an expert resource for Glycogenomics. Nucleic Acids Res. 2009;37:D233–D238.10.1093/nar/gkn663
- Benoit I, Danchin EG, Bleichrodt RJ, de Vries RP. Biotechnological applications and potential of fungal feruloyl esterases based on prevalence, classification and biochemical diversity. Biotechnol. Lett. 2008;30:387–396.10.1007/s10529-007-9564-6
- Kroon PA, Williamson G, Fish NM, Archer DB, Belshaw NJ. A modular esterase from Penicillium funiculosum which releases ferulic acid from plant cell walls and binds crystalline cellulose contains a carbohydrate binding module. Eur. J. Biochem. 2000;267:6740–6752.10.1046/j.1432-1033.2000.01742.x
- Knoshaug EP, Selig MJ, Baker JO, Decker SR, Himmel ME, Adney WS. Heterologous expression of two ferulic acid esterases from penicillium funiculosum. Appl. Biochem. Biotechnol. 2008;146:79–87.10.1007/s12010-007-8074-2
- Crepin VF, Faulds CB, Connerton IF. Functional classification of the microbial feruloyl esterases. Appl. Microbiol. Biotechnol. 2004;63:647–652.10.1007/s00253-003-1476-3
- Koseki T, Fushinobu S, Ardiansyah, Shirakawa H, Komai M. Occurrence, properties, and applications of feruloyl esterases. Appl. Microbiol. Biotechnol. 2009;84:803–810.10.1007/s00253-009-2148-8
- Yamanobe T, Mitsuishi Y, Takasaki Y. Isolation of a cellulolytic enzyme producing microorganism, culture conditions and some properties of the enzymes. Agric. Biol. Chem. 1987;51:65–74.10.1271/bbb1961.51.65
- Fujii T, Koike H, Sawayama S, Yano S, Inoue H. Draft Genome Sequence of Talaromyces cellulolyticus Strain Y-94, a Source of Lignocellulosic Biomass-Degrading Enzymes. Genome Announc. 2015;3:e00014–15.
- Inoue H, Fujii T, Yoshimi M, et al. Construction of a starch-inducible homologous expression system to produce cellulolytic enzymes from Acremonium cellulolyticus. J. Ind. Microbiol. Biotechnol. 2013;40:823–830.10.1007/s10295-013-1286-2
- Fujii T, Iwata K, Murakami K, Yano S, Sawayama S. Isolation of uracil auxotrophs of the fungus acremonium cellulolyticus and the development of a transformation system with the pyrF Gene. Biosci. Biotechnol. Biochem. 2012;76:245–249.10.1271/bbb.110498
- Watanabe M, Ishikawa K. Crystallization and preliminary X-ray crystallographic analysis of a putative feruloyl esterase from Talaromyces cellulolyticus. Acta Cryst. 2014;F70:1664–1667.
- Shibuya T, Watanabe Y, Nalley KA, Fusco A, Salafsky B. The BCA protein determination system. An analysis of several buffers, incubation temperature and protein standards. Tokyo Ika Daigaku Zasshi. 1989;47:677–682.
- Esteban-Torres MReverón I, Mancheno JM, de Las Rivas B, Muñoz R. Characterization of a feruloyl esterase from lactobacillus plantarum. Appl. Environ. Microbiol. 2013;79:5130–5136.10.1128/AEM.01523-13
- Skjold-Jørgensen J, Vind J, Svendsen A, Bjerrum MJ. Altering the activation mechanism in thermomyces lanuginosus lipase. Biochemistry. 2014;53:4152–4160.10.1021/bi500233h
- Sakoda M, Hiromi K. A method for the deductive and unique determination of the values of three parameters involved in fractional functions applicable to relaxation kinetics. J. Biochem. 1976;80:1335–1341.
- Biely P, Côté GL, Kremnický L, Greene RV, Tenkanen M. Action of acetylxylan esterase from Trichoderma reesei on acetylated methyl glycosides. FEBS Lett. 1997;420:121–124.10.1016/S0014-5793(97)01500-7
- Hisano T, Kasuya K, Tezuka Y, et al. The Crystal Structure of Polyhydroxybutyrate Depolymerase from Penicillium funiculosum Provides Insights into the Recognition and Degradation of Biopolyesters. J. Mol. Biol. 2006;356:993-1004.10.1016/j.jmb.2005.12.028
- Sánchez-González M, Blanco-Gámez A, Parra-Saldívar R, Mateos-Díaz JC, Estrada-Alvarado MI. Study of new feruloyl esterases to understand lipase evolution: the case of Bacillus flexus. Methods Mol. Biol. 2012;861:53–61.
- de Vries RP, vanKuyk PA, Kester HC, Visser J. The Aspergillus niger faeB gene encodes a second feruloyl esterase involved in pectin and xylan degradation and is specifically induced in the presence of aromatic compounds. Biochem. J. 2002;363:377–386.10.1042/0264-6021:3630377
- Levasseur A, Benoit I, Asther M, Asther M, Record E. Homologous expression of the feruloyl esterase B gene from Aspergillus niger and characterization of the recombinant enzyme. Protein Expr. Purif. 2004;37:126–133.10.1016/j.pep.2004.05.019
- Reinikainen T, Ruohonen L, Nevanen T, et al. Investigation of the function of mutated cellulose-binding domains of Trichoderma reesei cellobiohydrolase I. Proteins. 1992;14:475–482.10.1002/(ISSN)1097-0134
- Kraulis Per J., Clore GM, Nilges M, et al. Determination of the three-dimensional solution structure of the C-terminal domain of cellobiohydrolase I from Trichoderma reesei. A study using nuclear magnetic resonance and hybrid distance geometry-dynamical simulated annealing. Biochemistry. 1989;28:7241–7257.10.1021/bi00444a016
- Suzuki K, Hori A, Kawamoto K, et al. Crystal structure of a feruloyl esterase belonging to the tannase family: A disulfide bond near a catalytic triad. Proteins. 2014;82:2857–2867.10.1002/prot.24649
- Hotelier T, Renault L, Cousin X, Negre V, Marchot P, Chatonnet A. ESTHER, the database of the alpha/beta-hydrolase fold superfamily of proteins. Nucleic Acids Res. 2004;32:D145–D147.10.1093/nar/gkh141
- Payne CM, Resch MG, Chen L, et al. Glycosylated linkers in multimodular lignocellulose-degrading enzymes dynamically bind to cellulose. Proc. Nat. Acad. Sci. USA. 2013;110:14646–14651.10.1073/pnas.1309106110
- Sturtevant JM. Biochemical applications of differential scanning calorimetry. Ann. Rev. Phys. Chem. 1987;38:463–488.10.1146/annurev.pc.38.100187.002335
- Thompson JD, Higgins DG, Gibson TJ. CLUSTAL W: improving the sensitivity of progressive multiple sequence alignment through sequence weighting, position-specific gap penalties and weight matrix choice. Nucleic Acids Res. 1994;22:4673–4680.10.1093/nar/22.22.4673
- Gouet P, Robert X, Courcelle E. ESPript/ENDscript: extracting and rendering sequence and 3D information from atomic structures of proteins. Nucleic Acids Res. 2003;31:3320–3323.10.1093/nar/gkg556