Abstract
Protein–protein interactions (PPI) play key roles in various biological processes. The bimolecular fluorescence complementation (BiFC) assay is an excellent tool for routine PPI analyses in living cells. We developed new Gateway vectors for a high-throughput BiFC analysis of plants, adopting a monomeric Venus split just after the tenth β-strand, and analyzed the interaction between Arabidopsis thaliana coated vesicle coatmers, the clathrin heavy chain (CHC), and the clathrin light chain (CLC). In competitive BiFC tests, CLC interacted with CHC through a coiled-coil motif in the middle section of CLC. R1340, R1448, and K1512 in CHC and W94 in CLC are potentially key amino acids underlying the inter-chain interaction, consistent with analyses based on homology modeling. Our Gateway BiFC system, the V10-BiFC system, provides a useful tool for a PPI analysis in living plant cells. The CLC–CHC interaction identified may facilitate clathrin triskelion assembly needed for cage formation.
Graphical Abstract
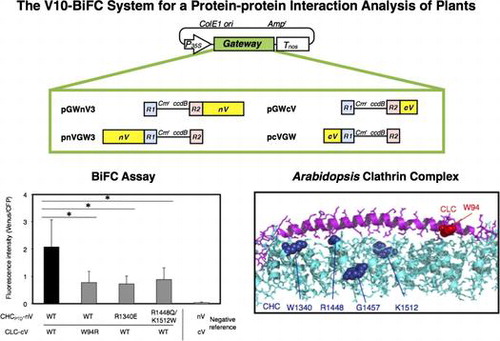
Various biological processes ranging from enzymatic actions, nutrition, energy and protein transport, cellular signal transduction, and cell-to-cell communication are mediated by protein–protein interactions (PPIs). Therefore, investigations of PPI are important for elucidating the molecular mechanisms responsible for these processes. Various methods such as bimolecular fluorescence complementation (BiFC),Citation1) bioluminescence resonance energy transfer (BRET),Citation2) and fluorescence resonance energy transfer (FRET) have been developed to analyze PPI in living cells.Citation3) The BiFC assay is an important tool for PPI analyses in living cells because it enables PPI to be visualized and quantified in these cells. The BiFC assay has been modified in order to increase sensitivity and specificity for the detection of PPI.Citation4) For example, brighter fluorescence reporters such as Venus have been shown to enable weak PPI to be detected with higher sensitivity than enhanced yellow fluorescent protein YFP (EYFP).Citation5) A series of fluorescence reporter tags split at various positions have been investigated in order to increase the specificity of the BiFC assay.Citation6) The non-specific interactions of the split fluorescence tags themselves have been detected in the BiFC assay, thereby leading to debate regarding the specificity of this assay.Citation7) We recently developed a series of plant Gateway destination vectors for the high-throughput cloning of genes of interest in order to evaluate the combination of BiFC-tagged proteins by a transient expression assay in plants, and successfully characterized the assembly of SEC13 and SEC31 proteins from Arabidopsis thaliana, which are coat subunits of the COPII vesicle machinery in plants.Citation8) However, difficulties have been associated with obtaining sufficient sensitivity and specificity for PPI analyses in the BiFC assay using these vectors, presumably due to the usage of EYFP as a split fluorescence tag.
Ohashi et al. recently developed a highly specific Venus-based BiFC system by examining vast combinations of a pair of Venus reporters split at various positions.Citation9) The BiFC reporter split between 210 and 211 just after the 10th β-strand of Venus did not interact with itself and enabled the detection of specific interactions between a pair of proteins of interest by evaluating differences between BiFC levels in the cofilin–actin interaction with and without functional point mutations. A similar type of Venus-based BiFC binary system to that described above has also been reported and was applied to the vast screening of PPI in planta by agroinfiltration-mediated transient expression.Citation10)
In this study, we developed new plant Gateway-compatible vectors for a high-throughput PPI analysis using the BiFC assay, which adopted a monomeric Venus split between residues 210 and 211, named the V10-BiFC system. We demonstrated the benefit of the high-throughput type of vector system along with a competitive BiFC assay by applying a PPI analysis of the A. thaliana clathrin heavy chain (CHC) and clathrin light chain (CLC), the 3D structures of which have not yet been clarified. The interaction between CLC and CHC of A. thaliana clathrin coats was analyzed in detail by our V10-BiFC system, and the results obtained were consistent with a computational molecular biological analysis.
Materials and methods
Materials
KOD-Plus- DNA polymerase was purchased from Toyobo (Osaka, Japan). BP clonase II and LR clonase II were from Invitrogen (Carlsbad, CA, USA). Phusion hot start II High-Fidelity DNA polymerase and fetal bovine serum (FBS) were supplied by Thermo Fisher Scientific (Waltham, MA, USA). Dulbecco’s modified Eagle’s medium (DMEM) was obtained from PAA Laboratories (Dartmouth, MA, USA). The following antibodies were purchased: anti-GFP from Nacalai Tesque (Kyoto, Japan) and anti-FLAG from Sigma-Aldrich (St. Louis, MO, USA). All other chemicals were of reagent or tissue culture grade.
Cell line culture and plasmid transfection
293T cells were cultured in DMEM containing 10% heat-inactivated FBS, 50 U/ml penicillin, and 50 mg/ml streptomycin. Cells were transfected using the CalPhos transfection kit (Takara Bio, Kyoto, Japan), according to the manufacturer’s instructions.
Plasmid construction
All plasmids used for BiFC and immunoprecipitation analyses were constructed by the Gateway cloning method. The probes of A. thaliana CLC and CHC (At3g11130, and At2g40060, respectively) used for these analyses are shown in Fig. . In PCR, KOD-plus DNA polymerase or Phusion hot start II High-Fidelity DNA polymerase was used to make amplified products with blunt ends. All primers and linkers used to construct plasmids are listed in Table S1. New plant Gateway-compatible BiFC vectors for fusion with a split Venus were constructed as follows: A pair of split Venus reporters were composed of the amino-terminal part, named nV, from residues 1 to 210 (the A of the translational initiation codon was designated +1) of Venus, and the carboxyl terminal part, named cV, from 211 to 239, both of which were created by PCR with Venus CDS as a template and the corresponding nV211-F, nV211-A207K-R, cV211-F, and cV211-R primers (Table S1). nV harbored the point mutation of A to K at residue 207 to reduce the formation of dimers.Citation11) Each fragment was ligated with the AfeI ends of a linearized pUGW0 or pUGW2Citation12) to make a series of new Gateway-compatible BiFC vectors such as pnVGW3, pcVGW, pGWnV3, and pGWcV, as shown in Fig. . These BiFC vectors were available for the expression of a protein (named X) fused with a split monomeric Venus fragment driven by a cauliflower mosaic virus (CaMV) 35S promoter, pnVGW3 for nV-X, pcVGW for cV-X, pGWnV3 for X-nV, and pGWcV for X-cV. These BiFC vectors were used to construct plasmids for a plant PPI analysis by BiFC in the transient expression system through biolistic bombardment.Citation8) Regarding the competitive BiFC analysis, a pUGW2C vector was created by replacing the 3′-terminal T of attR2 sequences with C in pUGW2 by PCR in order to remove the well-known binding motif for PPI.Citation13) The complete nucleotide sequences of the BiFC vectors such as pnVGW3, pcVGW, pGWnV3, pGWcV, and pUGW2C were registered in GenBank/EMBL/DDBJ as LC030196, LC030197, LC030198, LC030199, and LC030202, respectively. The corresponding CDSs to the CDS entry clones of CHC, CHCTD, CHCLADD, CHCHUB, CHCPTD, CLC, CLCF, CLCM, and CLCB were amplified from the cDNAs of CHC and CLC provided by RIKEN BRC and ABRC (resource numbers: RAFL16-77-M12 and U17121, respectively) using adaptor PCR with a pair of attB1-F and attB2-R primers after PCR with primers as follows: CHC-F and CHC-R (for CHC), CHC-F and CHCTD-R (for CHCTD), CHCLADD-F and CHCLADD-R (for CHCLADD), CHCHUB-F and CHC-R (for CHCHUB), and CHCPTD-F and CHC-R (for CHCPTD), CLC-F and CLC-R (for CLC), CLCF-R and CLC-F (for CLCF), CLCM-F and CLCM-R (for CLCM), and CLCB-F and CLC-R (for CLCB) (Table S1). The amplified DNA fragments were cloned into pDONR201 (Invitrogen) by a BP reaction according to the manufacturer’s instructions (Invitrogen) to construct the corresponding pDONR201/CHC, pDONR201/CHCTD, pDONR201/CHCLADD, pDONR201/CHCHUB, pDONR201/CHCPTD, pDONR201/CLC, pDONR201/CLCF, pDONR201/CLCM, and pDONR201/CLCB entry clones. The entry clones for CHCPTD-R1340E and CHCPTD-R1448W/K1512Q were constructed by a BP reaction following the amplification of the corresponding mutated CDSs from pDONR201/CHCPTD by overlapping PCR using CHCPTD-F, CHC-R1340E-R, CHC-R1448W-R, and CHC-K1512Q-R primers (Table S1). In order to construct the entry clones of CLC-W94R, pDONR201/CLC was used as a template for PCR with CLC-F, CLC-W94R-R, and CLC-R primers (Table S1). The resultant plasmids were subjected to an LR reaction with the BiFC vectors to construct the corresponding expression vectors. A pUGW2C vector was used as a Gateway destination vector in competitive BiFC in order to create expression vectors for competitors including CHCTD, CHCLADD, CHCHUB, CHCPTD, CLC, CLCF, CLCM, or CLCB. Mammalian Gateway-compatible vectors such as pEGFPGW and p3xFLAGGW for fusion with a green fluorescent protein (GFP) and 3× FLAG were constructed for immunoprecipitation and immunoblotting experiments, respectively, as follows: An EGFP-C1-XbaI-SacI linker was introduced into the BsrGI-BglII sites of pEGFP (Takara Bio) while a 3×FLAG-XbaI-SacI linker was introduced into p3×FLAG (Sigma-Aldrich) to make intermediate plasmids such as pEGFPn and p3×FLAGn, followed by the introduction of the XbaI-SacI fragment of pUGW0 containing the Gateway cassette into the intermediate plasmids to make the Gateway-compatible pEGFPGW and p3FLAGGW vectors, respectively (Table S1).Citation14) These plasmids were used to construct mammalian expression vectors such as pEGFPGW/CHC, pEGFPGW/CHCPTD, p3×FLAGGW/CLC, and p3×FLAGGW/CLCM for immunoprecipitation and immunoblotting analyses.
Fig. 1. Mapping probes used in a BiFC analysis, and immunoprecipitation analyses on the Arabidopsis CHC and CLC interaction.
Notes: Motifs predicted by Pfam are shown in the box. The amino acids used in the point mutation analysis are indicated by arrows. (A) A diagram of full-length CHC (At3g11130), including the terminal, linker, ankle, distal leg, knee, proximal leg, and trimerization domains as previously classified, is delineated.Citation17) Probes for full-length CHC and parts such as TD, LADD, HUB, and PTD were used in this study. (B) A diagram of full-length CLC (At2g40060) is delineated. The acidic residues EDD (amino acids at 5–7) are indicated by an arrowhead.Citation18,25) The predicted CHC-binding domain is shown in gray.Citation18) Probes for full-length CLC and parts such as F, M, and B were tested in this study.
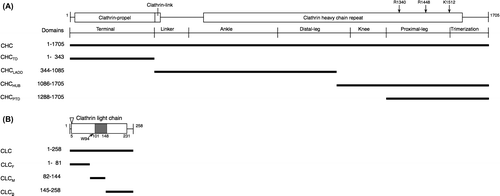
Fig. 2. Schematic illustration of four plant Gateway-compatible BiFC vectors for transient expression.
Notes: Ampr, ampicillin resistance marker used for selection in bacteria; ccdB, negative selection marker used in bacteria; Cmr, chloramphenicol resistance marker used for selection in bacteria; ColE1 ori, ColE1 replication origin; cV, C-terminal region (211–239) of Venus; nV, N-terminal region (1–210) of Venus; P35S, 35S promoter; R1, attR1; R2, attR2; Tnos, nopaline synthase terminator.
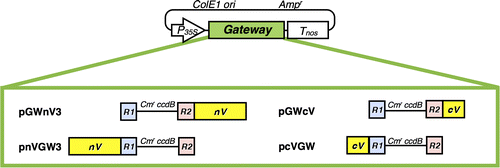
BiFC analysis
The BiFC vectors described above were used for an interaction analysis of plant proteins transiently transformed into Japanese leek epidermal cells by biolistic bombardment as previously reported.Citation8) As an internal reference, 1 μg of pUGW45Citation12) encoding ECFP driven by the CaMV 35S promoter was mixed with 0.5 μg of each BiFC plasmid, and was absorbed onto tungusten particles (1.0 μm in diameter) according to the manufacturer’s instructions (TANAKA, Sapporo, Japan). In the competitive BiFC analysis, 0.3 μg of each BiFC plasmid, 1 μg of pUGW45, and 3 μg of a suitable competitor plasmid were coated on bombarded tungsten particles. The inner epidermal peels of leek (1.5 square cm), the inner sides of which were oriented downwards, were placed on a 2% (w/v) agar plate. In each bombardment, leek epidermal cells were bombarded with 5 μl of DNA-coated tungsten particles in a plastic holder using a GIE-III IDERA particle gun (TANAKA) at a helium pressure of 5 kgf/cm2 under a vacuum of 600 mm Hg. Following bombardment, the cells were incubated on the same plate overnight at 22 °C in darkness and the fluorescence proteins that were transformed were viewed with a TCS SP5 laser scanning confocal microscope (Leica Microsystems, Wetzler, Germany) using a HCX PL APO CS 20.0 × 0.70 IMM UV objective lens. The BiFC of the split Venus fused with the proteins of interest was excited with an argon laser line (514 nm) to detect fluorescence at 520–555 nm while ECFP was excited with the same argon laser line (458 nm) to view fluorescence at 465–500 nm using the sequential scanning mode, respectively. In order to determine cellular BiFC levels, images of each BiFC and ECFP fluorescence in a leek epidermal cell expressing a pair of split Venus fusions together with ECFP as an internal reference were captured by the Z-stacking mode and total fluorescence levels of BiFC per ECFP in the individual cell were quantified using Leica LAS AF software according to the manufacturer’s instruction (Leica Microsystems).
Immunoprecipitation and immunoblotting
Cells were lysed in cell lysis buffer (50 mM HEPES-NaOH pH 7.5, 20 mM MgCl2, 150 mM NaCl, 1 mM dithiothreitol, 1 mM phenylmethane sulfonylfluoride, 1 mg/ml leupeptin, 1 mM EDTA, 1 mM Na3VO4, 10 mM NaF, and 0.5% Nonidet P-40). The proteins in the centrifugally cleaned supernatants were mixed with the ExactaCruz resin (Santa Cruz Biotechnology, Santa Cruz, CA, USA) that was preabsorbed with antibodies. The washed immunoprecipitates or the proteins in the cell lysates were denatured and then subjected to SDS-PAGE. Electrophoretically separated proteins were transferred to PVDF membranes, blocked, and immunoblotted first with primary antibodies and then with peroxidase-conjugated secondary antibodies. The bound antibodies were detected by chemiluminescence.
3D structure prediction
Models of the 3D structures of CHCPTD and CLC were generated based on the Bos taurus 3D structures of the orthologous proteins (Protein Data Bank IDs 3lvg and 3iyv). The amino acid residues of CHCPTD and CLC were aligned to the Bos taurus sequences by MAFFTash, and based on the alignments, the 3D structures were modeled by Spanner.Citation15) The resultant structures were visualized by the PyMOL Molecular Graphics System, Version 1.99, Schrödinger, LLC.
Statistical analysis
Each experiment was performed 2 to 4 times under the conditions in which the bombardments tested showed no detectable cytotoxicity. The significance of differences between two groups was determined by the Student’s t-test.
Results
Construction of new Gateway-compatible BiFC vectors for the high-throughput BiFC assay
In order to analyze PPI in plant living cells, we previously created a series of plant Gateway-compatible BiFC vectors.Citation8) However, difficulties have been associated with evaluating the existence of a weak protein interaction or with differences between a protein interaction with a wild-type protein and that with its functional mutant, presumably due to the lower sensitivity of enhanced YFP (EFYP). In addition, the weak dimerization characteristics of GFP variants are more likely to lead to difficulties in detecting a specific PPI.Citation11) These issues prompted us to replace the EYFP-based BiFC system with a Venus-based one, which adopted a Venus split between residues 210 and 211, as previously reported by Ohashi et al.Citation9) Furthermore, alanine at 207 was replaced with lysine in order to diminish the dimerization of the fluorescence proteins.Citation11) The resulting Gateway-compatible BiFC vectors, named the V10-BiFC system, are shown in Fig. . We then examined the availability of these vectors for a PPI analysis by transient expression in plant living cells through biolistic bombardment.
Availability of the V10-BiFC system for a PPI analysis of CHC and CLC from A. thaliana
The interaction between CLC and CHC from A. thaliana, coated proteins of clathrin-coated vesicles (CCVs), was investigated by the BiFC system in order to evaluate the accuracy and specificity of the newly developed V10-BiFC system. Plant CCV can function to sort proteins de novo synthesized in the endoplasmic reticulum from the trans-Golgi network into late endosomes as well as internalize integral proteins located on the plasma membrane into early endosomes in the mode of endocytosis.Citation16) The clathrin coat in animals was previously shown to be mainly composed of a heterotetrameric adaptor protein (AP) complex together with a microscopically visible structure with a three-legged shape, called a triskelion.Citation17) A triskelion comprises three CHCs, each harboring a single CLC. The tertiary structure of CHC was divided into seven characteristic segments, including the terminal, linker, ankle, distal leg, knee, proximal leg, and trimerization domains, from the amino to carboxyl terminal in that order (Fig. (A)). The 3D structure of A. thaliana clathrin currently remains unknown; however, it is likely to be very similar to those of other eukaryotes because most of the clathrin-coated proteins including CLC, CHC, and APs are conserved in this kind of organism. A recombinant CLC from A. thaliana was previously reported to interact with a recombinant HUB region including a set of knee, proximal leg, and trimerization domains of bovine CHC by a pull-down analysis in vitro,Citation18) and the in vivo interaction of CHC and CLC from A. thaliana in tobacco epidermal cells was demonstrated by the BiFC assay.Citation19) However, the region responsible for the interaction between both chains has yet to be elucidated in detail. In animals, CLC has been reported to directly interact with the proximal leg and trimerization domains (PTD) of CHC (CHCPTD) in a clathrin triskelion through the coiled-coil motif in the middle region of CLC (CLCM).Citation20) Therefore, we carried out a PPI analysis to explore the interactive site for the interaction between CHCPTD (amino acids at 1288–1705) and CLC from A. thaliana in more detail using the V10-BiFC system. We initially investigated the involvement of CLCM (amino acids at 82–144) in the CHCPTD–CLC interaction. We performed the BiFC assay using all combinations of the split Venus fusion of CHCPTD and CLCM. The results obtained indicated that the strongest interaction between both chains was detected with the combination of CHCPTD-nV and CLCM-cV as BiFC probes, as shown in Fig. S1. In order to evaluate the specificity of the interaction between CHCPTD and CLCM, we performed a competitive BiFC assayCitation21) by the co-bombardment of a plasmid harboring a clathrin chain without a BiFC tag at 10-fold amounts of the split Venus fusion vectors as a competitor. As shown in Fig. , the competitor led to significant decreases in BiFC levels caused by the presence of both CHCPTD-nV and CLCM-cV. The competitive BiFC clearly demonstrated the specific interaction between CHCPTD and CLCM, which could not be caused by the non-specific interaction between split Venus tags. Alternatively, we expressed both clathrin chains in mammalian 293T cells to investigate the molecular interaction between A. thaliana CHC and CLC using a co-immunoprecipitation assay. The results obtained demonstrated that CHC interacted with CLC (Fig. S2). CHCPTD formed an immunocomplex with CLCM, revealing the CHCPTD interaction with CLCM, which supported the data obtained from the BiFC assay and confirmed that our V10-BiFC system had sufficient specificity and quantitativity to recognize PPI and was capable of serving as a useful tool for plant PPI analyses.
Fig. 3. Interaction between CHCPTD and CLCM by a BiFC analysis.
Notes: (A) Visualization of the interaction between CHCPTD and CLCM by BiFC. Venus fluorescence images of leek epidermal cells expressing a pair of CHCPTD-nV and CLCM-cV with or without a competitor, or a pair of nV and cV as a negative reference were shown. Equal amounts of pUGW45 encoding ECFP were co-transformed in each cell as an internal reference. Bars show 20 μm. (B) Quantification of BiFC and evaluation of its specificity using a competitor. Non-fluorescence tagged CHCPTD by a 10-fold amount of BiFC-tagged CHCPTD used as a competitor. The resulting fluorescence intensity was calculated by dividing the fluorescence intensity from Venus by that from CFP (n = 15, error bars represent SD, **p < 0.001, t-test).
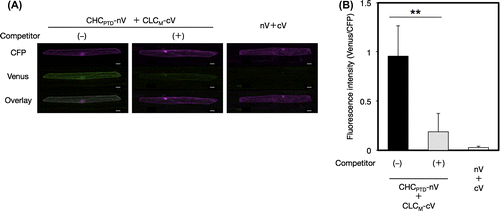
Interaction between CHCPTD and CLC through the middle region of CLC (CLCM)
The contribution of other regions besides CLCM for the interaction between CHCPTD and CLC was investigated by the BiFC assay. The front region (amino acids at residue 1–81, as shown in Fig. ) of CLC (CLCF) markedly interacted with CHCPTD, whereas no interaction was observed between the tail region (amino acids at residues 145–258) of CLC (CLCB) and CHCPTD, as shown in Fig. . However, the strength of the interaction between CHCPTD and CLCF was smaller than that between CHCPTD and CLCM or that between CHCPTD and CLC, suggesting that the dominant contribution to the interaction between CHCPTD and CLC is from CLCM.
Fig. 4. Interaction between CHCPTD and CLC by a BiFC analysis.
Notes: (A) Visualization of the interaction of CHCPTD with full-length or a part of CLC by a BiFC analysis. Venus fluorescence images of leek epidermal cells expressing CHCPTD-nV together with CLC-cV, CLCF-cV, or CLCB-cV as well as CFP as an internal reference were shown. Bars show 20 μm. (B and C) Evaluation of the specificity of interaction of CHCPTD with CLC-cV or CLCF-cV using a competitive BiFC assay, respectively. (D) Evaluation of the interaction between CHCPTD and CLCB by a BiFC analysis. Non-fluorescence tagged CLC (B) or CLCF (C) was used as a competitor. The resulting fluorescence intensity was calculated as described in Fig. (n = 10–14, error bars represent SD, ***p < 0.001, **p < 0.01, t-test).
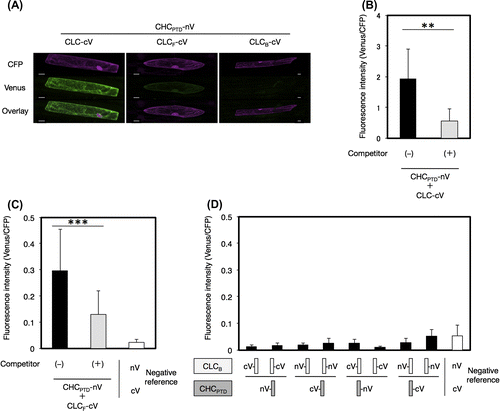
Interaction between CHC and CLC, presumably through not only CHCPTD, but also the other regions of CHC
CHC is composed of several structural regions including terminal, linker, ankle, distal leg, knee, proximal leg, and trimerization domains. We examined the contribution of the other regions of CHC besides CHCPTD, which was composed of proximal leg and trimerization domains, to the interaction between CHC and CLC by the BiFC analysis. The results obtained showed that each region including the terminal domain (CHCTD), a set of linker, ankle, and distal leg domains (CHCLADD), and an array of knee, proximal leg, and trimerization domains (CHCHUB) interacted with the CLC chain, with CHCHUB having the strongest interaction (Fig. ). These results suggested that the whole regions of CHC exhibited considerably potential to bind CLC and stabilize the structure of the clathrin lattice.
Fig. 5. Interaction of CLC with a part of CHC by a BiFC analysis.
Notes: The interaction of CLC with CHCTD (A), CHCLADD (B), or CHCHUB (C) was evaluated by a competitive BiFC assay. Non-fluorescence tagged CHCTD (A), CHCLADD (B), or CHCHUB (C) was used as a competitor. Plasmids encoding a pair of CLC-cV and a fragment of CHC-nV with or without a competitor, or a pair of nV and cV as a negative reference were bombarded on leek epidermal tissues. Equal amounts of a plasmid for CFP were also coated on bombarded particles as an internal reference. Quantification of the interaction between CHC and CLC and an evaluation of the specificity of this interaction was performed as described in Fig. (n = 10–15, error bars represent SD, **p < 0.0001, ***p < 0.005, t-test).
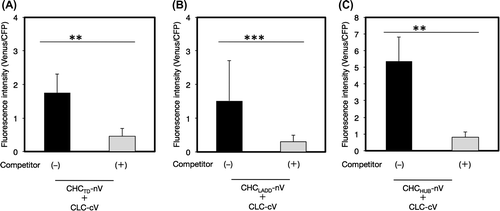
Candidates of key amino acid residues responsible for the interaction between CHCPTD and CLC
A point mutation analysis revealed that the substitution of tryptophans at 105, 127, or 138 in bovine CLC (BosLCb) to glutamine impaired the interaction with CHC, while the replacement of lysine at 1326 to glutamic acid or lysine at 1415 to glutamic acid in bovine CHC rescued these effects of CLC mutations, suggesting the involvement of these amino acids in the CHC–CLC interaction.Citation20) The alignment of amino acid sequences between CLC from A. thaliana and Bos taurus showed that tryptophan at 94 of A. thaliana CLC corresponded to that at 105 of bovine CLC, which is one of the interactive sites for CHC (Fig. ).Citation20) Based on the above findings,Citation20) a point mutation analysis was performed to determine whether W94 in CLC was involved in the interaction with CHCPTD. The replacement of W at 94 in CLC with R (CLC-W94R) caused lower BiFC levels with the CHCPTD–CLC interaction than those with non-substitution (Fig. (A)), indicating the significant contribution of W94 to the interaction between CHCPTD and CLC. As for bovine CHC, the substitution of lysine at 1326 with glutamic acid has been reported to suppress the mutational effects of W105R of CLC on its interaction with CHC.Citation20) Therefore, the effects of the corresponding mutation at 1340 of A. thaliana CHC from arginine to glutamic acid on the interaction with CLC were investigated. The results obtained revealed the interaction between both chains was decreased by this mutation. In addition, BiFC levels with the CHCPTD–CLC interaction were attenuated and not rescued in the case of a pair of CHCPTD-R1340E and CLC-W94R (Fig. (B)). Furthermore, a set of natural substitutions at 1434, from arginine to tryptophan, at 1443, from lysine to glycine, and at 1498, from arginine to glutamine of human CHC, were previously reported to impair the binding of CHC to CLC.Citation22) As shown in Fig. , arginine at 1448 and lysine at 1512 of A. thaliana CHC corresponded to that at 1434 and 1498 of human CHC, respectively. The point mutation analysis indicated that R1448 W together with K1512Q attenuated the interaction between CHCPTD and CLC (Fig. (A)). These results suggest significance of R1340, R1448, and K1512 in CHC for the interaction between CLC and CHCPTD.
Fig. 6. Alignment of amino acid sequences of Arabidopsis CHCs and CLCs including point mutations in BiFC analyses.
Notes: (A) Alignment of the amino acid sequences of AtCHC-I (Arabidopsis thaliana, At3g08530), AtCHC-II (Arabidopsis thaliana, At3g11130), GmCHC (Glycine Max, AAC49294), CHC22-1 (Homo sapiens, NP_009029), BosCHC (Bos taurus, BTU31757), and mCtlc (Mus musculus, NM_001003908) using ClustalW2. Alignment of the mutated sequences of AtCHC-II such as R1340E, R1448 W, and K1512Q used in the BiFC analysis is indicated in white and boxed as I, II, and IV.Citation20,22) Glycine at 1557 of Arabidopsis CHC, indicated as III, was not used in the analysis because of the same amino acid as the substitution of K at 1443 to G of Bos taurus CHC.Citation22) (B) Alignment of the amino acid sequences of AtCLC-I (Arabidopsis thaliana, At2g20760), AtCLC-II (Arabidopsis thaliana, At2g40060), AtCLC-III (Arabidopsis thaliana, At3g51890), BosLCa (Bos taurus, X04851), and BosLCb (Bos taurus, X04853) using ClustalW2. The CHC-binding domain is shown by a line. Alignment of the mutated sequence of W94R for the BiFC analysis is in white and boxed. Positions corresponding to a pair of residues from bovine clathrin chains, shown by compensating mutations to interact with both chains, are boxed as I and V.Citation20) Positions corresponding to residues from CHC22-1, reported to not bind CLC, are boxed as II–IV.Citation22)
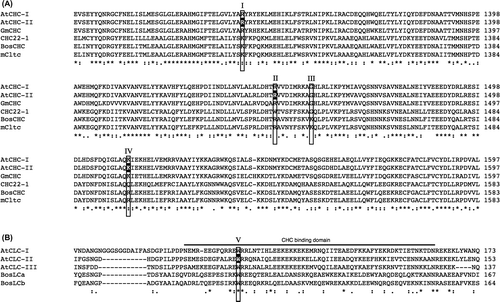
Fig. 7. Effects of the mutation of conserved amino acids on the interaction of CHCPTD and CLC by a BiFC analysis.
Notes: Effects of the mutation of conserved amino acids on the interaction of CHCPTD and CLC by a BiFC analysis. (A) A pair of plasmids encoding CLC-cV or CHC-nV with or without mutated amino acids excluding a pair of R1340E in CHCPTD and W94R in CLC, or a pair of plasmids for nV and cV as a negative reference were bombarded on leek epidermal tissues. (B) A pair of plasmids encoding CLC-cV or CHC-nV or of those encoding CHCPTD-R1340E-nV and CLC-W94R-cV were co-bombarded. Equal amounts of a plasmid for CFP were also coated on bombarded particles as an internal reference. The resulting fluorescence intensity was calculated as described in Fig. (n = 10–14, error bars represent SD, *p < 0.003, t-test).
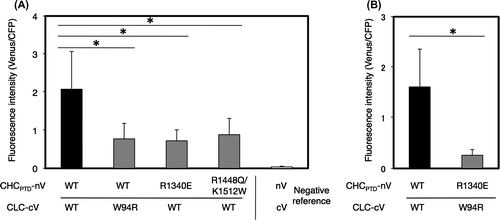
Homology modeling of 3D structures of A. thaliana CHCPTD and CLC
We modeled 3D structures in order to examine the structural plausibility of interactions between CHCPTD and CLC indicated by the BiFC data. Homology modeling of A. thaliana CHCPTD showed that the 3D structure was similar to that of the bovine ortholog, consistent with previous findings (Fig. S3).Citation23) Furthermore, homology modeling of the interface between CHCPTD and CLC demonstrated that the structure of the CHCPTD and CLC complex from A. thaliana was very similar to that of the bovine clathrin complex (Fig. (A)). A close examination of the Arabidopsis model revealed that the mutated amino acids R1340, R1448, and K1512 in CHCPTD and W94 in CLC were predicted to be located in the interface between the chains (Fig. (B)); thus, these amino acids were good candidates for those involved in binding the clathrin complex.
Fig. 8. The 3D structure of the CHC–CLC complex of Bos taurus and that of the predicted orthologous complex of Arabidopsis thaliana.
Notes: (A) Structures of the interface between CLC and the carboxyl terminal of CHC. (B) Detailed structures of the complex with the amino acids mutated in this study. The mutated amino acids in CHC and CLC in PPI analyses are shown in purple and red, respectively.
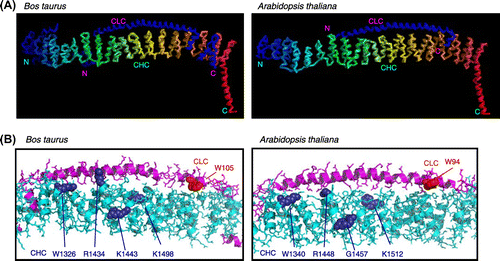
Discussion
Many techniques have been developed in order to analyze PPI in living cells because PPI play significant roles in various biological processes. Of these, the BiFC assay is one of the routine tools for PPI as well as protein dynamics and localization analyses in living cells because it enables PPI to be visualized in living cells. The BiFC assay has several significant points that make it ideal for PPI analyses. High sensitivity is required for the detection of a weak PPI by the BiFC assay. Furthermore, high specificity is advantageous for distinguishing differences between a protein interaction with a non-mutated protein and that with its mutant. The plasmids available for the high-throughput cloning of genes of interest are also convenient for the BiFC assay because eight plasmids encoding each BiFC-tagged protein are required to determine the pair of BiFC plasmids suitable for the detection of a specific PPI. We previously created plant Gateway BiFC vectors for transient expression in plant living cells.Citation8) However, difficulties were associated with obtaining sufficient sensitivity and specificity for PPI analyses in the BiFC assay using these vectors, presumably due to the split-EYFP tag. In addition, a previous study reported that a non-specific interaction caused by the split fluorescence reporter tags themselves occasionally occurred in the BiFC assay, leading to debate regarding the specificity of the BiFC assay for PPI analyses.Citation7) Therefore, it was necessary to confirm that BiFC obtained in experiments was due to specific PPI. Ohashi et al. demonstrated the specificity of the Venus-based BiFC system by testing vast combinations of a pair of Venus reporters split at various positions.Citation9) The BiFC reporter tags split between 210 and 211 just after the 10th β-strand of Venus did not interact with themselves and also enabled the detection of specific interactions between a pair of proteins fused to each BiFC tag by evaluating differences between the BiFC levels from protein interactions with a non-mutated protein and those with its functional mutant. Difficulties are associated with appreciating the specific interaction of a pair of proteins, the functional mutations in which remain unknown. In this case, one of the most appropriate ways to evaluate the specificity of the BiFC assay for PPI is to perform a competitive BiFC assay, a PPI analysis using no BiFC-tagged protein of interest as a competitor, which is expected to specifically interfere with the interaction between the two proteins tested.Citation21) We herein developed new plant Gateway-compatible vectors for a PPI analysis by a BiFC assay adopting a monomeric Venus split between residues 210 and 211, named the V10-BiFC system. We demonstrated the benefits of this high-throughput type of vector system in combination with a competitive BiFC assay for application to a PPI analysis of A. thaliana CHC and CLC, the 3D structures of which have not yet been elucidated.
Regarding plant clathrin, three CLC and two CHC isoforms have been identified in A. thaliana.Citation24) CHCs are well conserved between plants and animals, whereas plant CLCs have low similarities to other organisms, suggesting that CLC may have a plant-specific function. In this study, our plant Gateway V10-BiFC system, which was developed in combination with a competitive BiFC assay, demonstrated that A. thaliana CHCPTD interacted with its CLC, the middle region (CLCM) of which preferentially contributed to the CHCPTD–CLC interaction (Figs. and ), and this was supported by data obtained from co-immunoprecipitation experiments (Fig. S2). CLCM harbors a conservative coiled-coil motif, suggesting the involvement of the motif in the CHCPTD–CLC interaction, as previously reported.Citation18,20) In addition, a homology modeling analysis suggested that the 3D structure of the complex of CLC and CHCPTD from A. thaliana was very similar to that of the bovine clathrin complex (Fig. ). CLCF also strongly interacted with CHCPTD (Fig. ). Mammalian CLCs have acidic amino acid sequences at their amino-terminal ends, which reportedly bind CHCHUB and inhibit CHC assembly by controlling high-affinity salt bridges.Citation25) These acidic residues have also been detected in three CLCs from A. thaliana, suggesting the potential contribution of the acidic residues EDD (amino acids at 5–7) in CLCF to the CHCPTD–CLC interaction (Fig. (B)).Citation18)
Regarding A. thaliana CHC, each section such as TD, LADD, and HUB (including PTD) significantly interacted with CLC, with the strongest interaction being detected between CHCHUB and CLC (Fig. ). A bovine CHC ortholog was previously reported to assemble together at its carboxyl terminal to form a triskelion structure harboring three legs, each of which was associated with CLC in its PTD region, as demonstrated by an immunoelectron microscopic analysis of bovine clathrin coat proteins.Citation22,26) CHC has seven repeated coiled–coiled structures, called CHC repeats, which contribute to the interaction with polypeptide chains to form a triskelion. Electron micrography of a bovine clathrin cage cryostat demonstrated that CLC on the CHCPTD in a triskelion may also be located close to the other regions of CHC belonging to a separate nascent triskelion.Citation27) Therefore, the interaction of CLC with whole sections of CHC suggested that CLC bridged the interaction between each CHC in two separate neighboring triskelia in order to stabilize the clathrin cage structure in A. thaliana.
Arginines at residues 1340 and 1448, and lysine at 1512 in CHC as well as tryptophan at 94 in CLC from A. thaliana may be involved in the interaction between these chains (Fig. ). However, the substitution of arginine at 1340 with glutamine in CHC did not rescue the defective interaction between CHCPTD and CLC caused by the W94R mutation of CLC in A. thaliana, in contrast with the structural analysis of the Bos taurus clathrin previously reported,Citation20) thereby implying that the 3D structure of A. thaliana clathrin may be similar to, but not the same as that of bovine CLC. Homology modeling of the 3D structure of the complex of CLC and CHCPTD suggested that the amino acids analyzed in the point mutation tests may all be located along the interface between both chains (Fig. ). The amino acids in mammalian clathrin that corresponded to the amino acids of A. thaliana CLC were previously shown to be involved in the interaction between animal CHC and CLC, which was supported by data obtained from electron cryomicrography of the bovine clathrin structure.Citation27) Therefore, the structure of the Arabidopsis clathrin cage appears to be similar to that of the mammalian cage; however, further experiments are needed to confirm this.
Another group recently reported the same types of Venus-based BiFC vectors as our Gateway V10-BiFC system, which was a non-Gateway binary vector applied to high-throughput screening of PPI analyses in planta by transient expression through agroinfiltration.Citation10) This binary system is an ingenious tool because it uses a single binary vector harboring a pair of any type of split Venus-tagged protein and CFP as a marker protein for agrobacterium-mediated transformation; however, the evaluation of specific PPI by the binary BiFC system requires further development. We herein applied the plant V10-BiFC system to a high-throughput quantitative plant PPI analysis in combination with a competitive BiFC assay. Competitor proteins need to exist in competitive BiFC tests before the expression of a pair of proteins fused to BiFC reporters or all proteins need to be expressed simultaneously in order to evaluate the specificity of PPI because the interaction between split GFP variants is an irreversible reaction, at least in vitro.Citation21) Our transient high-throughput V10-BiFC system by biolistic bombardment is suitable for performing a competitive BiFC analysis because of the feasibility for adjusting optimal amounts of competitors against a pair of BiFC fusion proteins. Therefore, it is possible to routinely use our system for a domain–domain interaction analysis with high specificity as conventional PPI methods including a yeast two-hybrid assay or as transient PPI experiments in mammalian cell lines such as HEK293 cells. Taken together, our newly developed plant Gateway V10-BiFC system is expected to become a useful high-throughput tool for elucidating interactions between proteins of interest, thereby providing valuable information on PPI in plant living cells.
Author contributions
Nishimura, K., Ishikawa, S., Matsunami, E., Yamauchi, J., Homma, K., Faulkner, C., Oparka, K., Jisaka, M., Nagaya, T., Yokota, K., and Nakagawa, T. designed research; Nishimura, K., Ishikawa, S., Matsunami, E., Yamauchi, J., and Homma, K. performed research; Nishimura, K. and Matsunami, E. contributed analytic tools; Nishimura, K., Matsunami, E., Yamauchi, J., and Homma, K. analyzed data; and Nishimura, K., Yamauchi, J., Homma, K., Faulkner, C., and Nakagawa, T. wrote the paper.
Supplemental data
Supplemental Table S1 and Figs. S1-S3 for this article can be accessed at http://dx.doi.org/10.1080/09168451.2015.1060847.
Disclosure statement
No potential conflict of interest was reported by the authors.
Funding
This work was supported by the Japan Society for the Promotion of Science under the Japan—UK Research Cooperative Program from the Ministry of Education, Culture, Sports, Science, and Technology of Japan (to KN).
TableS1.tif
Download TIFF Image (8.3 MB)FigureS1.ppt
Download MS Power Point (263.5 KB)FigureS2.ppt
Download MS Power Point (1 MB)FigureS3.ppt
Download MS Power Point (371 KB)Acknowledgments
We thank Dr. Atsushi Miyawaki in the RIKEN BSI for the gift of the Venus clone, and the RIKEN BRC and ABRC for distributing full-length Arabidopsis clathrin clones. We are also grateful to Ms. Kanae Shibata of Leica Microsystems for the quantification of fluorescence images.
Notes
Abbreviations: Ampr, ampicillin resistance marker; AP, adaptor protein; BiFC, bimolecular fluorescence complementation; CaMV, cauliflower mosaic virus; ccdB, negative selection marker; CCV, clathrin-coated vesicle; CHC, clathrin heavy chain; CLC, clathrin light chain; Cmr, chloramphenicol resistance marker; ColE1 ori, ColE1 replication origin; cV, C-terminal region (211-239) of Venus; DMEM, Dulbecco’s modified Eagle’s medium; EDTA, ethylenediaminetetraacetic acid; FBS, fetal bovine serum; ECFP, enhanced cyan fluorescent protein; GFP, green fluorescent protein; Kmr, kanamycin resistance marker; Tnos, nopaline synthase terminator; nV, N-terminal region (1-210) of Venus; ORF, open reading frame; P35S, 35S promoter; PCR, polymerase chain reaction; PPI, protein–protein interaction; PVDF, polyvinylidene fluoride; SD, standard deviation; SDS-PAGE, SDS‐polyacrylamide gel electrophoresis; sGFP, synthetic GFP with S65T; TGN, trans-Golgi network; YFP, yellow fluorescent protein.
References
- Ghosh I, Hamilton AD, Regan L. Antiparallel leucine zipper-directed protein reassembly: application to the green fluorescent protein. J. Am. Chem. Soc. 2000;122:5658–5659.10.1021/ja994421w
- Xu Y, Piston DW, Johnson CH. A bioluminescence resonance energy transfer (BRET) system: application to interacting circadian clock proteins. Proc. Natl. Acad. Sci. USA. 1999;96:151–156.
- Heim R, Tsien RY. Engineering green fluorescent protein for improved brightness, longer wavelengths and fluorescence resonance energy transfer. Curr. Biol. 1996;6:178–182.10.1016/S0960-9822(02)00450-5
- Kodama Y, Hu CD. Bimolecular fluorescence complementation (BiFC): a 5-year update and future perspectives. BioTechniques. 2012;53:285–298.
- Shyu YJ, Liu H, Deng X, et al. Identification of new fluorescent protein fragments for bimolecular fluorescence complementation analysis under physiological conditions. BioTechniques. 2006;40:61–66.10.2144/000112036
- Kerppola TK. Bimolecular fluorescence complementation (BiFC) analysis as a probe of protein interactions in living cells. Annu. Rev. Biophys. 2008;37:465–487.10.1146/annurev.biophys.37.032807.125842
- Shyu YJ, Hu CD. Fluorescence complementation: an emerging tool for biological research. Trends Biotechnol. 2008;26:622–630.10.1016/j.tibtech.2008.07.006
- Hino T, Tanaka Y, Kawamukai M, et al. Two Sec13p homologs, AtSec13A and AtSec13B, redundantly contribute to the formation of COPII transport vesicles in Arabidopsis thaliana. Biosci. Biotechnol. Biochem. 2011;75:1848–1852.10.1271/bbb.110331
- Ohashi K, Kiuchi T, Shoji K, et al. Visualization of cofilin-actin and Ras-Raf interactions by bimolecular fluorescence complementation assays using a new pair of split Venus fragments. BioTechniques. 2012;52:45–50.
- Gookin TE, Assmann SM. Significant reduction of BiFC non-specific assembly facilitates in planta assessment of heterotrimeric G-protein interactors. Plant J. 2014;80:553–567.10.1111/tpj.2014.80.issue-3
- Zacharias DA, Violin JD, Newton AC, et al. Partitioning of lipid-modified monomeric GFPs into membrane microdomains of live cells. Science. 2002;296:913–916.10.1126/science.1068539
- Nakagawa T, Kurose T, Hino T, et al. Development of series of gateway binary vectors, pGWBs, for realizing efficient construction of fusion genes for plant transformation. J. Biosci. Bioeng. 2007;104:34–41.10.1263/jbb.104.34
- Bonifacino JS, Traub LM. Signals for sorting of transmembrane proteins to endosomes and lysosomes. Annu. Rev. Biochem. 2003;72:395–447.10.1146/annurev.biochem.72.121801.161800
- Torii T, Miyamoto Y, Sanbe A, et al. Cytohesin-2/ARNO, through its interaction with focal adhesion adaptor protein paxillin, regulates preadipocyte migration via the downstream activation of Arf6. J. Biol. Chem. 2010;285:24270–24281.10.1074/jbc.M110.125658
- Standley DM, Toh H, Nakamura H. ASH structure alignment package: sensitivity and selectivity in domain classification. BMC Bioinf. 2007;8:116.10.1186/1471-2105-8-116
- Fujimoto M, Ueda T. Conserved and plant-unique mechanisms regulating plant post-Golgi traffic. Front. Plant Sci. 2012;3:197.
- Kirchhausen T, Owen D, Harrison SC. Molecular structure, function, and dynamics of clathrin-mediated membrane traffic. Cold Spring Harb. Perspect. Biol. 2014;6:a016725.10.1101/cshperspect.a016725
- Scheele U, Holstein SE. Functional evidence for the identification of an Arabidopsis clathrin light chain polypeptide. FEBS Lett. 2002;514:355–360.10.1016/S0014-5793(02)02439-0
- Van Damme D, Gadeyne A, Vanstraelen M, et al. Adaptin-like protein TPLATE and clathrin recruitment during plant somatic cytokinesis occurs via two distinct pathways. Proc. Natl. Acad. Sci. USA. 2011;108:615–620.10.1073/pnas.1017890108
- Chen CY, Reese ML, Hwang PK, et al. Clathrin light and heavy chain interface: α-helix binding superhelix loops via critical tryptophans. EMBO J. 2002;21:6072–6082.10.1093/emboj/cdf594
- Hu CD, Chinenov Y, Kerppola TK. Visualization of interactions among bZIP and Rel family proteins in living cells using bimolecular fluorescence complementation. Mol. Cell. 2002;9:789–798.10.1016/S1097-2765(02)00496-3
- Ybe JA, Brodsky FM, Hofmann K, et al. Clathrin self-assembly is mediated by a tandemly repeated superhelix. Nature. 1999;399:371–375.10.1038/20708
- Zeng MH, Liu SH, Yang MX, et al. Characterization of a gene encoding clathrin heavy chain in maize up-regulated by salicylic acid, abscisic acid and high boron supply. Int. J. Mol. Sci. 2013;14:15179–15198.10.3390/ijms140715179
- Baisa GA, Mayers JR, Bednarek SY. Budding and braking news about clathrin-mediated endocytosis. Curr. Opin. Plant Biol. 2013;16:718–725.10.1016/j.pbi.2013.09.005
- Ybe JA, Greene B, Liu SH, et al. Clathrin self-assembly is regulated by three light-chain residues controlling the formation of critical salt bridges. EMBO J. 1998;17:1297–1303.10.1093/emboj/17.5.1297
- Kirchhausen T, Toyoda T. Immunoelectron microscopic evidence for the extended conformation of light chains in clathrin trimers. J. Biol. Chem. 1993;268:10268–10273.
- Fotin A, Cheng Y, Sliz P, et al. Molecular model for a complete clathrin lattice from electron cryomicroscopy. Nature. 2004;432:573–579.10.1038/nature03079