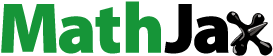
Abstract
Pyrroloquinoline quinone (PQQ), an aromatic tricyclic o-quinone, was identified initially as a redox cofactor for bacterial dehydrogenases. Although PQQ is not biosynthesized in mammals, trace amounts of PQQ have been found in human and rat tissues because of its wide distribution in dietary sources. Importantly, nutritional studies in rodents have revealed that PQQ deficiency exhibits diverse systemic responses, including growth impairment, immune dysfunction, and abnormal reproductive performance. Although PQQ is not currently classified as a vitamin, PQQ has been implicated as an important nutrient in mammals. In recent years, PQQ has been receiving much attention owing to its physiological importance and pharmacological effects. In this article, we review the potential health benefits of PQQ with a focus on its growth-promoting activity, anti-diabetic effect, anti-oxidative action, and neuroprotective function. Additionally, we provide an update of its basic pharmacokinetics and safety information in oral ingestion.
Graphical abstract
Structure and redox reaction of pyrroloquinoline quinone (PQQ).
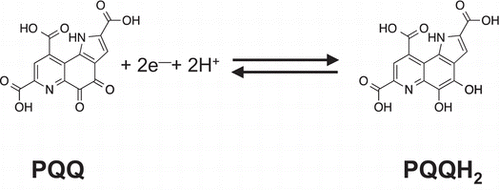
Pyrroloquinoline quinone (PQQ, Fig. ) is an aromatic tricyclic o-quinone that serves as a redox cofactor of a number of prokaryotic dehydrogenases, such as alcohol and sugar dehydrogenases.Citation1,2) More recently, the first eukaryotic PQQ-dependent sugar oxidoreductase has been discovered in a mushroom, the basidiomycete Coprinopsis cinerea.Citation3) Although PQQ is not biosynthesized in mammals, trace amounts of PQQ have been found in human and rat tissues at picomolar to nanomolar levels,Citation4) and an especially large amount has been found in human milkCitation5) because of its presence in daily foods, including vegetables and meats.Citation6–8) PQQ is a ubiquitous molecule that influences a multitude of physiological and biochemical processes and has been established to be beneficial for growth and stress tolerance in both bacteria and higher organisms.Citation9,10) Most importantly, nutritional studies have revealed that PQQ deficiency in mice and rats exhibits various systemic responses, including growth impairment, compromised immune responsiveness, abnormal reproductive performance, and reduced respiratory quotient.Citation11–13) Moreover, in 2003, Kasahara and Kato reported that PQQ could qualify as a newcomer to the B group of vitamins.Citation14) These authors cloned a presumed mouse homolog (U26) of the yeast gene, 2-aminoadipic acid reductase (LYS2), and proposed that U26 could be involved in the metabolic degradation of dietary lysine, acting as a PQQ-dependent 2-aminoadipic 6-semialdehyde dehydrogenase, because U26 contained the putative PQQ-binding motif that is conserved among bacterial PQQ-dependent dehydrogenases.Citation14) However, claims for a mammalian vitamin have been questioned because conclusive evidence for the existence of a mammalian PQQ-dependent enzyme is lacking.Citation15,16) Although currently there remains controversy over whether PQQ is indeed an essential vitamin in mammals, PQQ has been discovered to have a diverse range of physiological properties that could be beneficial to human health over the last decade. The objectives of this review were to, first, present an overview of the recent insights gained on the potential health benefits of PQQ in anti-diabetic, anti-oxidative, and neuroprotective actions, and second, update its metabolism and safety information in pharmacological applications.
I. Chemical nature of PQQ
PQQ (4,5-dihydro-4,5-dioxo-1H-pyrrolo[2,3-f]quinoline-2,7,9-tricarboxylic acid) is a redox active o-quinone that can be reversibly reduced to pyrroloquinoline quinol (4,5-dihydroxy-1H-pyrrolo[2,3-f]quinoline-2,7,9-tricarboxylic acid, PQQH2) through a semiquinone intermediate (Fig. ).Citation17) It has been demonstrated that PQQ stably acts as an efficient electron transfer catalyst from a number of organic substrates to molecular oxygen (O2), constructing quinoprotein model reactions. In the presence of ascorbate, NAD(P)H, and thiol compounds such as glutathione, PQQ undergoes a two-electron reduction to form PQQH2.Citation18–20) Subsequently, the generated PQQH2 is oxidized back to the original quinone via the reduction of two equivalents of O2 to super oxide anion (), which spontaneously or enzymatically dismutates to hydrogen peroxide (H2O2).Citation21) It is noteworthy that PQQ has the ability to catalyze continuous redox cycling so that picomole amounts of PQQ are capable of generating micromolar amounts of product.Citation17,22) Meanwhile, PQQ can exert pro-oxidant actions by the formation of reactive oxygen species (ROS), such as
and H2O2, via its redox cycling under certain conditions and induce oxidative protein modifications, including the oxidation of cysteinyl thiols.Citation23) PQQ also catalyzes the oxidation of primary amines, including the ε-amino group of lysine residues in elastin and collagen, via Schiff base formation under aerobic conditions.Citation24) Elastin oxidation by PQQ in the presence of Cu2+ results in the formation of 2-aminoadipic semialdehyde residues and eventually its derived covalent cross-links. On the other hand, PQQ easily reacts with amino acids to form imidazole derivatives, such as imidazolopyrroloquinoline quinone, in biological samples, and these derivatives are biologically active in some cases.Citation5,8) The protonated form of PQQ shown in Fig. dissolves only slightly in water, and the tricarboxylic acid of PQQ dissociates in neutral pH water. Therefore, PQQ disodium salt (PQQ Na2) is generally used in various examinations because of its high solubility in waster. The atomic geometry of PQQ Na2 (commercially available as BioPQQ™, a trademark of Mitsubishi Gas Chemical Co., Inc., (Tokyo, Japan)) was resolved using single-crystal X-ray structure analysis as PQQ Na2 tri-hydrate.Citation25) PQQ Na2 has an advantage in the application to various experiments compared with free-form PQQ, because it can be handled easily owing to its water-soluble property. BioPQQ™, chemically well-characterized, was used in the following studies.
II. Natural source of PQQ
It is well known that PQQ is distributed ubiquitously in nature and found in numerous dietary sources, including fermented soy beans (natto), tea, green peppers, parsley, kiwi fruit, and human milk.Citation5,6) Various methods for instrumental analyses and bioassays for PQQ have been developed, but the PQQ content in foods varies in different reports because PQQ is chemically reactive and prone to form derivatives or condensation products with other nutrients.Citation5,6,8,26,27) Kumazawa et al. have developed a method based on gas chromatography/mass spectrometry (GC/MS) with isotopic dilution for free PQQ after derivatization with phenyltrimethylammonium hydroxide.Citation4,6,7) Using this analytical method, the levels of free PQQ in various foods, including vegetables, fruits, and teas, were determined to be in the range of 3.7–61 ng/g wet weight or ng/mL in liquid foods. Recent analyses of PQQ using a reliable liquid chromatography/electrospray-ionization tandem mass spectrometry (LC/MS/MS) method elucidated that free PQQ was present in various food samples in the range of 0.19–7.02 ng/g fresh weight or ng/mL in liquid foods.Citation8) Based on available food composition data,Citation4–7) it is estimated that humans consume 0.1–1.0 mg PQQ and its derivatives per day.Citation28)
The biosynthesis of PQQ in higher organisms has not been shown, and therefore, the major source of PQQ in these organisms, including plants and animals, is believed to be derived from microorganisms. The details of PQQ biosynthesis have not been resolved yet, but a putative pathway has been proposed on the basis of the functions of conserved genes in numerous bacteria.Citation29) The majority of PQQ-producing bacteria contain six genes (pqqABCDEF) in an operon.Citation10,30) These genes have been expressed in Escherichia coli, a non-PQQ producer and lead to the production of PQQ.Citation31,32) Genetic knockout studies of each of these genes show that four of the six gene products (PqqA, PqqC, PqqD, and PqqE) are absolutely required for PQQ production.Citation32,33) In all cases, pqqA encodes a small polypeptide, typically 20–30 amino acids in length, containing a conserved glutamate and tyrosine that serve as the backbone in PQQ biogenesis.Citation34,35) The glutamate and tyrosine undergo post-translational modifications to form the intermediate 3a-(2-amino-2-carboxyethyl)-4,5-dioxo-4,5,6,7,8,9-hexahydroquinoline-7,9-dicarboxylic acid (AHQQ).Citation32,34) PqqC is the most characterized and has been shown to catalyze the eight-electron oxidation and ring cyclization of AHQQ to form PQQ.Citation35) A large number of bacteria extracellularly excrete PQQ and is indicated as micrograms per mL of broth culture.Citation36) On the other hand, common strains of bacteria in the human intestinal tract appear to synthesize little PQQ,Citation37,38) and hence, it seems that dietary intake is the major source of PQQ in the human body.
III. Growth-promoting activity
Although no enzymes in animals have been identified that exploit PQQ as a cofactor, PQQ has been shown to be essential for normal growth and development in animals. When PQQ is omitted from a chemically defined diet fed to mice and rats, various systemic responses are observed including growth impairment, immune dysfunction, decreased reproductive performance, and reduced respiratory quotient.Citation11–14) Oral supplementation of PQQ (above 300 ng/g diet) improves reproduction and enhances neonatal rates of growth compared with the response from diets devoid of PQQ.Citation12) More recently, dietary supplementation of PQQ Na2 in broiler chicks has been shown to improve growth performance, carcass yield, immunity, and plasma status.Citation39) Thus, this unique compound is characterized as an important growth factor or putative essential nutrient in animals, whereas the nutritional benefits of PQQ for human growth and development are still unknown. Although the detailed mechanism of PQQ action in animals still remains unclear, the ability to carry out continuous redox cycling suggests a role for PQQ as a cofactor, redox signaling molecule, or anti-oxidant.
In cultured human and mouse cells, PQQ also functions as a potential growth factor to promote cell proliferation when added to culture media.Citation40–42) PQQ enhances the incorporation of [3H]-thymidine into human skin fibroblasts cultured in medium containing PQQ at concentrations as low as 3 nM. Kumazawa et al. have observed that PQQ treatment stimulates activation of extracellular signal-regulated kinase 1/2 (ERK 1/2) in c-Ha-ras transformed NIH/3T3 mouse fibroblasts, resulting in increased cell proliferation.Citation41) ERK, one of the mitogen-activated protein kinases, activates transcription in the ras-signaling pathway and plays a pivotal role in cell proliferation and survival.Citation43) This signal transduction by sequential phosphorylation often is initiated by the binding of peptide growth factors to receptor tyrosine kinases (RTKs). Recently, we showed that PQQ also significantly enhanced proliferation of human epithelial A431 cells at concentrations above 10 nM.Citation42) Moreover, we found that PQQ induces the activation (tyrosine autophosphorylation) of epidermal growth factor receptor (EGFR), a RTK of the ErbB family, and its downstream target ERK 1/2 in a ligand-independent manner. The activation of the ERK pathway accompanying EGFR phosphorylation via binding of EGF plays a prominent role in the proliferation of epithelial cells. On the other hand, EGFR signaling is negatively regulated by protein tyrosine phosphatase 1B (PTP1B), which catalyzes tyrosine dephosphorylation of activated EGFR, and the inhibition of PTP1B has been reported to evoke a ligand-independent activation of EGFR.Citation44,45) Recent findings also indicate that PTP1B activity is modulated by post-translational modification, such as oxidation and alkylation of an extremely reactive cysteine residue at the catalytic center.Citation46) On the basis of these facts, we have elucidated that PQQ inhibits PTP1B through the oxidation of catalytic cysteinyl thiol by H2O2 produced during its redox cycling, thereby inducing the ligand-independent activation of EGFR (Fig. ). PTP1B has a substrate-specific ability to dephosphorylate RTKs, including the insulin receptor (IR),Citation47) insulin-like growth factor-I receptor,Citation47) platelet-derived growth factor receptor,Citation48) vascular endothelial growth factor receptor,Citation49) and nerve growth factor (NGF) receptor,Citation50) implicating the modulation of multiple growth factor-activated signaling pathways. Hence, our data suggests that inhibition of PTP1B via redox cycling by PQQ might induce a diverse range of physiological effects through potentiated RTK-mediated signaling and gene expression and exert a growth factor-like action.
Fig. 2. Proposed mechanism for the ligand-independent activation of epidermal growth factor receptor (EGFR) signaling through redox cycling of pyrroloquinoline quinone (PQQ). PQQ undergoes redox cycling in the presence of reductants, such as ascorbate and glutathione, and then produces and H2O2. The generated H2O2 inactivates protein tyrosine phosphatase 1B (PTP1B) via the oxidation of catalytic cysteinyl thiol (Cys-215) to the corresponding sulfenic acid (–SOH), sulfinic acid (–SO2H), and sulfonic acid (–SO3H). The inhibition of PTP1B evokes the EGF-independent activation (tyrosine phosphorylation) of EGFR and subsequent activation (serine/threonine phosphorylation) of ERK 1/2.
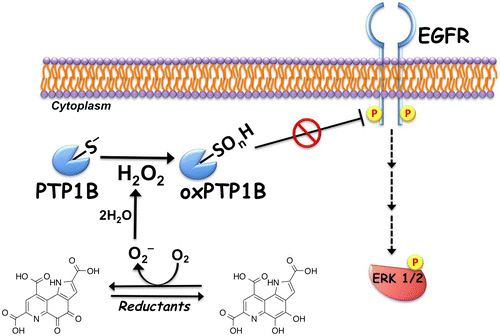
IV. Anti-diabetic effects
Accounting for 90–95% of diabetic population, type 2 diabetes mellitus (T2DM) has increased rapidly in recent decades worldwide, and the morbidity and mortality associated with secondary complications of the disease, such as retinopathy, nephropathy, and cardiovascular disease, also have increased significantly.Citation51) T2DM is characterized by mitochondrial disorder and chronic hyperglycemia and dyslipidemia resulting from insulin resistance of the peripheral tissues and impaired insulin secretion from the pancreas.Citation52) Mitochondria regulate metabolic pathways through signal transduction that is essential for metabolic homeostasis and cellular function. Recent studies show that mitochondrial dysfunction of diabetic subjects is closely related to lifestyle factors, including diet, physical activity, sleep, and stress.Citation53,54) Prolonged exercise and diet intervention can reverse, at least partly, the mitochondrial deficiency and improve the metabolic flexibility and insulin sensitivity in patients with T2DM.Citation54,55) Recently, dietary PQQ supplementation has been revealed to enhance mitochondrial function and biogenesis and improve metabolic homeostasis in mice and rats.Citation56–58) PQQ deficiency in young mice increases the plasma glucose level, reduces hepatic mitochondrial content by 20–30%, and suppresses mitochondrial respiration.Citation56) Similarly, rats fed a diet deficient in PQQ exhibit elevated plasma lipid and ketone bodies owing to lower mitochondrial content and decreased energy expenditure.Citation57) More importantly, PQQ supplementation reverses the mitochondrial alterations and metabolic impairment and significantly improves the lipid profile in diabetic UCD-T2DM rats.Citation56,57) Mechanistically, mitochondrial biogenesis and function are stimulated by the transcriptional coactivator, peroxisome proliferator-activated receptor gamma coactivator-1 alpha (PGC-1α), through activation of the nuclear respiratory factor (NRF-1 and NRF-2).Citation59) The transcription factor cAMP-responsive element-binding protein (CREB) increases transcription of PGC-1α via a conserved CREB-binding site in the proximal promoter and is activated by exercise or fasting.Citation60) Indeed, the exposure of mouse Hepa 1–6 hepatocytes to PQQ elevates PGC-1α promoter activity by enhancing CREB transcriptional activity and stimulating mitochondrial biogenesis (Fig. (A)).Citation57,61) PQQ exposure also increases the levels of NRF-1 and NRF-2, resulting in the upregulation of the mitochondrial transcription factor A (Tfam) and mitochondrial gene expression. However, the molecular mechanism underlying the activation of CREB-PGC-1α signaling pathway by PQQ remains unclear.
Fig. 3. Pyrroloquinoline quinone (PQQ)-induced activation of cAMP-responsive element-binding protein (CREB)-peroxisome proliferator-activated receptor gamma coactivator-1 alpha (PGC-1α) and insulin signaling. (A) Proposed mechanism for PQQ-induced activation of CREB-PGC-1α signaling pathway. PQQ stimulates the phosphorylation and activation of CREB and enhances PGC-1α expression. Increased PGC-1α binds to and coactivates the transcriptional function of nuclear respiratory factor (NRF)-1/2 on the mitochondrial transcription factor A (Tfam) promoter. Tfam plays a crucial role in regulating mtDNA amplification and mitochondrial biogenesis. (B) Proposed mechanism for the ligand-independent activation of insulin signaling through redox cycling of PQQ. PQQ inhibits protein tyrosine phosphatase 1B (PTP1B) to oxidatively modify the catalytic cysteine through its redox cycling activity. The inhibition of PTP1B evokes the insulin-independent activation (tyrosine phosphorylation) of the insulin receptor (IR) and subsequent phosphorylation of insulin receptor substrate-1 (IRS-1) and Akt. Phosphorylated Akt stimulates translocation of glucose transporter 4 (GLUT4) to the plasma membrane, resulting in increased cellular glucose uptake.
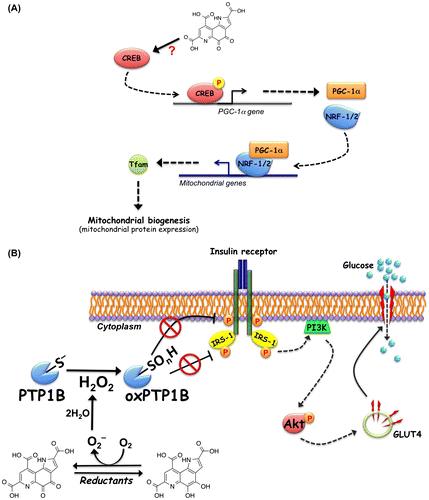
Insulin resistance, defined as a dysfunction of insulin target cells, such as hepatocytes, skeletal muscle cells, and adipocytes, to respond to the action of insulin, plays a pivotal role in the development of several metabolic abnormalities and T2DM. Mitigating insulin resistance has been considered as a primary clinical strategy to improve metabolic control in T2DM subjects. The underlying molecular pathophysiology of insulin resistance still is not well understood, but a number of lines of evidence point to a critical role of PTP1B in insulin resistance. PTP1B negatively regulates insulin signaling by catalyzing dephosphorylation of tyrosine residues in activated IRand IR substrate-1 (IRS-1).Citation47,62) Recently, a correlation between insulin resistance states and up-regulation of PTP1B expression in adipose and muscle tissues in humans has been reported.Citation63–65) Furthermore, transgenic overexpression of PTP1B in muscle attenuates the tyrosyl phosphorylation of IR and IRS-1, leading to insulin resistance.Citation66) On the other hand, PTP1B-knockout mice exhibit an elevated sensitivity to insulin with increased tyrosyl phosphorylation of the IR in the liver and muscle.Citation67,68) Thus, the inhibition of PTP1B has emerged as a potential therapeutic strategy to treat T2DM.Citation69) More recently, we found that PQQ elicits the ligand-independent activation of insulin signaling by inhibiting cellular PTP1B and enhances glucose uptake through the translocation of glucose transporter 4 in mouse C2C12 myotubes (Fig. (B)).Citation70) In addition, we demonstrated that oral administration of PQQ (20 mg·kg−1 day−1) for two weeks improved impaired glucose tolerance in type 2 diabetic KK-Ay mice. Our findings clearly suggest that PQQ can be useful in anti-diabetic treatment for T2DM subjects.
V. Anti-oxidative action
PQQ is reduced easily to PQQH2 by reaction with reducing agents such as NADPH, sodium borohydride, glutathione, or cysteine. A couple of in vitro studies demonstrated that the reduced form of PQQ (PQQH2) exhibits anti-oxidative capacity.Citation71–75) The aroxyl radical-scavenging activity of PQQH2 was 7.4-fold higher than that of vitamin C, which is known as the most active water-soluble anti-oxidant.Citation73) The singlet oxygen-quenching activity of PQQH2 was found to be 6.3-fold higher than that of vitamin C.Citation74) Interestingly, PQQH2 works as catalyst in the singlet oxygen-quenching reactions. Moreover, it has been clarified that PQQH2 may rapidly convert two molecules of α-tocopheroxyl radicals to α-tocopherol.Citation75) These results indicate that the pro-oxidant effect of α-tocopheroxyl radicals is suppressed by the coexistence of PQQH2. The summary of the radical quenching reaction is shown in Fig. .
Fig. 4. The summary of radical quenching reactions. Pyrroloquinoline quinol (PQQH2) can be made from pyrroloquinoline quinone (PQQ) by reduction of NADPH, cysteine, and glutathione. Aroxyl radicals, singlet oxygen, and α-tocopheroxyl radicals are quenched by PQQH2.

In experiments using cultured cells, it was reported that PQQ Na2 prevents oxidative stress-induced neuronal death.Citation76,77) It has been shown that PQQ prevented 6-hydroxydopamine (6-OHDA)-induced cell death of the dopaminergic neuroblastoma cell line SH-SY5Y and primary rat neurons and that its preventive effect was stronger than that of vitamin C and E.Citation76) 6-OHDA is a well-known neurotoxin that compromises mitochondria complex I, resulting in the production of ROS, such as , hydroxyl radicals, and H2O2. Similar results were obtained in the experiment using H2O2.Citation77)
Moreover, marked decreases in ischemia damage are found in in vivo rat models, such as cardiovascularCitation78,79) or cerebral ischemia models.Citation80,81) The underlying mechanisms elucidated were that PQQ acts as an anti-oxidant by scavenging and protects mitochondria from oxidative stress-induced damage.Citation58)
In humans, following a single dose of PQQ Na2 (0.2 mg/kg body weight), thiobarbituric acid reactive products (TBARS), which are measured by the malondialdehyde generated from lipid hydroperoxides, significantly decreased over the time course of the study.Citation28) In addition, the change of TBARS values correlated significantly with the maximum plasma concentration (Cmax) for PQQ Na2. These results suggest that PQQ has a potential as an anti-oxidant.
VI. Neuroprotection and brain function
VI.I. In vitro studies
Neurons are susceptible to receive lethal damage from oxidative stress. This neuronal death is regarded as a cause of neurodegenerative diseases such as Alzheimer’s disease and Parkinson’s disease. In in vitro studies, the ability of PQQ Na2 to protect human neuroblastoma SH-SY5Y cells from oxidative stress by 6-OHDA or H2O2 was tested.Citation76,77) The cell viabilities were recovered in a dose-dependent manner by adding PQQ Na2. The inhibitory activity of PQQ Na2 was much higher than that of vitamin C or vitamin E at the concentrations tested. These results suggest that the protective effect of PQQ on 6-OHDA or H2O2-induced neurotoxicity is involved in its function as a radical scavenger, especially .
One of the interesting effects of PQQ is enhancement of NGF production. NGF, a protein composed of 118 amino acid residues, is well known as a neurotrophic factor required for the development and maintenance of peripheral sympathetic and sensory neurons. PQQ is shown to have a stimulatory effect on NGF synthesis/secretion in astroglial cells and in fibroblast cells without cytotoxicity.Citation82,83) The precise mechanism of enhancement of NGF by PQQ is not yet clear; however, cyclooxygenase activation is supposed to be an essential process, because the induction of NGF is inhibited by cyclooxygenase inhibitor or dexamethasone.Citation84)
VI.II. In vivo studies
The effects of PQQ on the learning and memory function of young rats were investigated using the Morris water maze test.Citation85) The rats in this study were fed a diet supplemented 20 mg PQQ Na2/(kg body weight/day) for nine weeks. Rats fed a PQQ Na2-supplemented diet showed significantly better learning ability than the control rats. In addition, after receiving hyperoxia to induce oxidative stress for 48 h, rats fed PQQ Na2-supplemented diets showed better memory function than the control rats. The combination of PQQ Na2 (20 mg PQQ/(kg body weight/day)) with Coenzyme Q10 (300 mg/(kg body weight/day)) showed synergistic effects on memory function. The effect is independent of vitamin E, because the vitamin E-deficient rats did not show the effect with PQQ Na2-supplemented diets. Similar effects were observed in aged rats.Citation86) These results suggest that PQQ is potentially effective for preventing neurodegeneration caused by oxidative stress.
VI.III. Human studies
A placebo-controlled, double-blinded study using the repeatable battery for the assessment of neuropsychological status (RBANS) was conducted with the participation of 65 Japanese subjects between 50 and 70 years old who presented with self-identified forgetfulness or forgetfulness identified by a family member, colleague, or acquaintance.Citation87) RBANS is a neuropsychological battery developed by Randolph in the United States.Citation88) The neuropsychological battery questions allow repeated and quick evaluation of higher brain function disorders with a variety of brain disease complications. The content of the RBANS consists of five subtests of neurocognitive test paradigms [immediate memory, visuospatial/constructional, language, attention, and delayed memory]. Although the PQQ Na2 (20 mg/day) and PQQ Na2 (20 mg/day) + Coenzyme Q10 (300 mg/day) groups showed significantly better total score over time, a similar improvement over time was seen in the placebo group. Differences in immediate memory scores at week eight were significantly better in the PQQ Na2 + Coenzyme Q10 group than in the placebo group. For analysis of immediate memory, subjects were stratified into two subgroups according to baseline total scores. Although no significant difference was present between groups in the high-scoring subgroup, the PQQ Na2 + Coenzyme Q10 group in the low-scoring subgroup showed a significantly better score at week 8 and week 16 than the placebo group. This finding shows that individuals with lower RBANS scores may achieve a better degree of improvement in response to PQQ Na2-supplementation than individuals with higher scores.
The result of another human clinical study was reported very recently.Citation89) A randomized, placebo-controlled, double-blinded study to examine the effect of PQQ Na2 on cognitive functions was conducted with 41 elderly healthy subjects. Subjects were administered orally 20 mg of PQQ Na2/day or placebo for 12 weeks. For cognitive functions, selective attention by the Stroop and reverse Stroop testCitation90) and visual-spatial cognitive function by the laptop tablet Touch MCitation91) were evaluated. In the Stroop test, the change of Stroop interference ratios for the PQQ Na2 group was significantly smaller than for the placebo group. In the Touch M test, the stratification analyses dividing each group into two groups showed that the score significantly increased only in the lower group of the PQQ Na2 group (initial score < 70).
Relating to cognitive functions, PQQ Na2 shows effects on stress, fatigue, and sleep. Seventeen adult and female subjects participated in a clinical trial using an open-label trial to evaluate the effectiveness of PQQ Na2 on stress, fatigue, quality of life, and sleep.Citation92) The participants ingested 20 mg of PQQ Na2 daily for eight weeks. The results in the Profile of Mood States–Short Form showed that all six measures of vigor, fatigue, tension-anxiety, depression, anger-hostility, and confusion significantly improved following PQQ Na2 supplementation compared with scores for those measures before supplementation of PQQ Na2. The results of the Oguri–Shirakawa–Azumi Sleep Inventory (Middle-aged and Aged version) showed significant improvement in drowsiness at awaking, sleep onset and maintenance, and sleep duration. For validation, the Pittsburgh Sleep Quality Index Japanese version also showed significant improvement in sleep-related behavior. Furthermore, the changes in these global scores were correlated with changes in the cortisol awakening response, i.e., the effects of PQQ Na2 on improvement of sleep quality are supported by a biomarker.
Recently, two papers were published regarding the effect of PQQ Na2 on health benefit in humans.Citation93,94) PQQ Na2 is helpful for the improvement of skin conditions and lipid metabolism. PQQ Na2 may be useful not only for the improvement of brain functions but also for various health benefits. The underlying mechanisms of the effects of PQQ Na2 should be elucidated further.
VII. Safety
Since 2009, dietary supplements containing PQQ Na2 have been commercialized in the United States after the official acceptance of notification by the Food and Drug Administration, and no adverse effects have been reported. As for oral toxicity studies, a 14-day preliminary study and a 28-day repeated dose study, as acute studies, and a 13-week subchronic study were performed in rats.Citation95) The median lethal dose was 1000–2000 mg PQQ Na2/kg body weight in male and 500–1000 mg PQQ Na2/kg body weight in female rats. In the 14-day study, high doses of PQQ Na2 resulted in increases in relative kidney weights with associated histopathology in female rats only, while a follow-up 28-day study in female animals resulted in increases in urinary protein and crystals. These findings were reversible and resolved during the recovery period. In the 13-week study, a number of clinical chemistry findings and histopathological changes were noted, which were deemed to be of no toxicological significance, as the levels were within the historical control range, were not dose-dependent, occurred at a similar frequency in control groups, or occurred only in the control group. Based on these findings, a no-observed-adverse effect level (NOAEL) of 100 mg PQQ Na2/kg body weight was determined in rats, the highest dose tested in the 13-week study. A recent study reported that the NOAEL of PQQ Na2 in rats is considered to be 400 mg PQQ Na2/kg body weight for both sex, the highest dose tested.Citation96)
Additionally, the genotoxic potential of PQQ Na2 was evaluated in a core battery of genotoxicity tests.Citation97) The results of the bacterial mutation assay (Ames test) were negative. Weak positive results were obtained in two separate in vitro chromosomal aberration tests at the highest dosage in Chinese hamster lung fibroblasts. Upon testing in an in vitro chromosomal aberration test in human peripheral blood lymphocytes, no genotoxic activity was noted. In the in vivo micronucleus assay in mice, PQQ Na2 at doses up to 2000 mg/kg body weight demonstrated that no genotoxic effects are expressed in vivo in bone marrow erythrocytes. From these results, PQQ was concluded to have no genotoxic activity in vivo.
A placebo-controlled, double-blinded safety studies in humans have been reported.Citation98) PQQ Na2 at 20 or 60 mg/day or placebo was administered for four weeks to healthy volunteers. No adverse effects were observed in standard clinical blood tests at both dosages of PQQ Na2. In the 60 mg PQQ Na2/day dosage test, the urinary concentration of N-acetyl-β-d-glucosaminidase (NAG), which is a sensitive biomarker for renal tubular damage, did not change after the administration of PQQ Na2.
[14C]PQQ was administered orally to mice to estimate absorption. PQQ was readily absorbed (62%) in the lower intestine and was excreted by the kidney (81%) within 24 h.Citation99) Following a single dose of PQQ (0.2 mg PQQ Na2/kg body weight), levels of PQQ peaked in the serum after 3 h of administration at a concentration around 10 nM. The rise and clearance of PQQ Na2 in serum paralleled the change in urine.Citation28) From these studies, the pharmacokinetic behavior of PQQ Na2 seems similar to other water-soluble vitamin B group compounds. This suggests that PQQ does not accumulate considerably in the body to produce severe damage. In rats, when PQQ Na2 was injected intraperitoneally daily for 4 days at a dose of 11.5 mg/kg body weight, functional, and morphologic changes of the kidney were observed.Citation100) The oral administration does not give as high a blood concentration of PQQ as intraperitoneal injection, but it is necessary to monitor for excess dosage.
VIII. Conclusions
PQQ has been shown to be a ubiquitous molecule that influences a multitude physiological and biochemical processes. In this review, we have presented recent studies supporting the role of PQQ in maintaining and improving human health. There are potential benefits from PQQ supplementation related to lipidemic and glycemic control, prevention of cardiovascular and neurodegenerative diseases, and improvement of brain functions. Recent evidence suggests that PQQ can be useful for various health benefits through different mechanisms including redox activity, radical-scavenging activity, and modulation of cell signaling pathways. According to recent observations, PQQ shows no toxicity and genotoxicity in oral administration, and thus, oral supplementation of PQQ would be a promising approach to improving health status. On the other hand, the precise molecular mechanism underlying the action of PQQ is not understood fully. The mechanistic studies that aid in defining the function of PQQ could provide further benefits for human health.
Disclosure statement
No potential conflict of interest was reported by the authors.
References
- Goodwin PM, Anthony C. The biochemistry, physiology and genetics of PQQ and PQQ-containing enzymes. Adv. Microb. Physiol. 1998;40:1–80.
- Anthony C. Pyrroloquinoline quinone (PQQ) and quinoprotein enzymes. Antioxid. Redox Signal. 2001;3:757–774.10.1089/15230860152664966
- Matsumura H, Umezawa K, Takeda K, et al. Discovery of a eukaryotic pyrroloquinoline quinone-dependent oxidoreductase belonging to a new auxiliary activity family in the database of carbohydrate-active enzymes. PLoS One. 2014;9:e104851. 10.1371/journal.pone.0104851
- Kumazawa T, Seno H, Urakami T, Matsumoto T, Suzuki O. Trace levels of pyrroloquinoline quinone in human and rat samples detected by gas chromatography/mass spectrometry. Biochim. Biophys. Acta. 1992;1156:62–66. 10.1016/0304-4165(92)90096-D
- Mitchell AE, Jones AD, Mercer RS, Rucker RB. Characterization of pyrroloquinoline quinone amino acid derivatives by electrospray ionization mass spectrometry and detection in human milk. Anal. Biochem. 1999;269:317–325. 10.1006/abio.1999.4039
- Kumazawa T, Sato K, Seno H, Ishii A, Suzuki O. Levels of pyrroloquinoline quinone in various foods. Biochem. J. 1995;307:331–333.
- Kumazawa T, Seno H, Suzuki O. Failure to verify high levels of pyrroloquinoline quinone in eggs and skim milk. Biochem. Biophys. Res. Commun. 1993;193:1–5. 10.1006/bbrc.1993.1581
- Noji N, Nakamura T, Kitahata N, et al. Simple and sensitive method for pyrroloquinoline quinone (PQQ) analysis in various foods using liquid chromatography/electrospray-ionization tandem mass spectrometry. J. Agric. Food Chem. 2007;55:7258–7263. 10.1021/jf070483r
- Ghosh S, Chakraborty R, Raychaudhuri U. Pyrroloquinoline quinone a redox cofactor and its involvement in biological system. Int. J. Sci. Nat. 2013;4:371–380.
- Choi O, Kim J, Kim JG, et al. Pyrroloquinoline quinone is a plant growth promotion factor produced by Pseudomonas fluorescens B16. Plant Physiol. 2008;146:657–668.
- Killgore J, Smidt C, Duich L, et al. Nutritional importance of pyrroloquinoline quinone. Science. 1989;245:850–852. 10.1126/science.2549636
- Steinberg FM, Gershwin ME, Rucker RB. Dietary pyrroloquinoline quinone: growth and immune response in BALB/c mice. J. Nutr. 1994;124:744–753.
- Steinberg F, Stites T, Anderson P, et al. Pyrroloquinoline quinone improves growth and reproductive performance in mice fed chemically defined diets. Exp. Biol. Med. 2003;228:160–166.
- Kasahara T, Kato T. Nutritional biochemistry: a new redox-cofactor vitamin for mammals. Nature. 2003;422:832.10.1038/422832a
- Felton LM, Anthony C. Biochemistry: role of PQQ as a mammalian enzyme cofactor? Nature. 2005;433:E10. 10.1038/nature03322
- Rucker R, Storms D, Sheets A, Tchaparian E, Fascetti A. Biochemistry: is pyrroloquinoline quinone a vitamin? Nature. 2005;433:E10–E11.10.1038/nature03323
- Paz MA, Martin P, Flückiger R, Mah J, Gallop PM. The catalysis of redox cycling by pyrroloquinoline quinone (PQQ), PQQ derivatives, and isomers and the specificity of inhibitors. Anal. Biochem. 1996;238:145–149. 10.1006/abio.1996.0267
- Itoh S, Kato N, Ohshiro Y, Agawa T. Catalytic oxidation of thiols by coenzyme PQQ. Chem. Lett. 1985;14:135–136.10.1246/cl.1985.135
- Itoh S, Kato N, Mure M, Ohshiro Y. Kinetic studies on the oxidation of thiols by coenzyme PQQ. Bull. Chem. Soc. Jpn. 1987;60:420–422.10.1246/bcsj.60.420
- Itoh S, Kinugawa M, Mita N, Ohshiro YJ. Efficient NAD+-regeneration system with heteroaromatic o-quinones and molecular oxygen. Chem. Soc. Chem. Commun. 1989;1989:694–695. 10.1039/c39890000694
- Itoh S, Ohshiro Y, Agawa T. Reaction of reduced PQQ(PQQH2) and molecular oxygen. Bull. Chem. Soc. Jpn. 1986;59:1911–1914. 10.1246/bcsj.59.1911
- Stites TE, Mitchell AE, Rucker RB. Physiological importance of quinoenzymes and the O-quinone family of cofactors. J Nutr. 2000;130:719–727.
- Ishii T, Akagawa M, Naito Y, et al. Pro-oxidant action of pyrroloquinoline quinone: characterization of protein oxidative modifications. Biosci. Biotechnol. Biochem. 2010;74:663–666. 10.1271/bbb.90764
- Shah MA, Bergethon PR, Boak AM, Gallop PM, Kagan HM. Oxidation of peptidyl lysine by copper complexes of pyrroloquinoline quinone and other quinones. A model for oxidative pathochemistry. Biochim. Biophys. Acta. 1992;1159:311–318. 10.1016/0167-4838(92)90061-H
- Ishida T, Doi M, Tomita K, Hayashi H, Inoue M, Urakami T. Molecular and crystal structure of PQQ (methoxatin), a novel coenzyme of quinoproteins: extensive stacking character and metal ion interaction. J. Am. Chem. Soc. 1989;111:6822–6828. 10.1021/ja00199a050
- Ameyama M, Nonobe M, Shinagawa E, Matsushita K, Adachi O. Method of enzymatic determination of pyrroloquinoline quinone. Anal. Biochem. 1985;151:263–267. 10.1016/0003-2697(85)90174-5
- Bergethon PR. Amperometric electrochemical detection of pyrroloquinoline quinone in high-performance liquid chromatography. Anal. Biochem. 1990;186:324–327. 10.1016/0003-2697(90)90089-R
- Harris CB, Chowanadisai W, Mishchuk DO, Satre MA, Slupsky CM, Rucker RB. Dietary pyrroloquinoline quinone (PQQ) alters indicators of inflammation and mitochondrial-related metabolism in human subjects. J. Nutr. Biochem. 2013;24:2076–2084.10.1016/j.jnutbio.2013.07.008
- Puehringer S, Metlitzky M, Schwarzenbacher R. The pyrroloquinoline quinone biosynthesis pathway revisited: a structural approach. BMC Biochem. 2008;9:8. 10.1186/1471-2091-9-8
- Shen YQ, Bonnot F, Imsand EM, RoseFigura JM, Sjölander K, Klinman JP. Distribution and properties of the genes encoding the biosynthesis of the bacterial cofactor, pyrroloquinoline quinone. Biochemistry. 2012;51:2265–2275.10.1021/bi201763d
- Meulenberg JJ, Sellink E, Loenen WA, Riegman NH, Kleef M, Postma PW. Cloning of Klebsiella pneumoniae pqq genes and PQQ biosynthesis in Escherichia coli. FEMS Microbiol. Lett. 1990;71:337–343. 10.1111/fml.1990.71.issue-3
- Klinman JP, Bonnot F. Intrigues and intricacies of the biosynthetic pathways for the enzymatic quinocofactors: PQQ, TTQ, CTQ, TPQ, and LTQ. Chem. Rev. 2014;114:4343–4365.10.1021/cr400475g
- Velterop JS, Sellink E, Meulenberg JJ, David S, Bulder I, Postma PW. Synthesis of pyrroloquinoline quinone in vivo and in vitro and detection of an intermediate in the biosynthetic pathway. J. Bacteriol. 1995;177:5088–5098.
- Bonnot F, Iavarone AT, Klinman JP. Multistep, eight-electron oxidation catalyzed by the cofactorless oxidase, pqqc: identification of chemical intermediates and their dependence on molecular oxygen. Biochemistry. 2013;52:4667–4675. 10.1021/bi4003315
- Magnusson OT, Toyama H, Saeki M, et al. Quinone biogenesis: Structure and mechanism of PqqC, the final catalyst in the production of pyrroloquinoline quinone. Proc. Natl. Acad. Sci. USA. 2004;101:7913–7918.10.1073/pnas.0402640101
- van Kleef MA, Duine JA. Factors relevant in bacterial pyrroloquinoline quinone production. Appl. Environ. Microbiol. 1989;55:1209–1213.
- Smidt CR, Bean-Knudsen D, Kirsch DG, Rucker RB. Does the intestinal microflora synthesize pyrroloquinoline quinone? Biofactors. 1991;3:53–59.
- Matsushita K, Arents JC, Bader R, Yamada M, Adachi O, Postma PW. Escherichia coli is unable to produce pyrroloquinoline quinone (PQQ). Microbiology. 1997;143:3149–3156.10.1099/00221287-143-10-3149
- Samuel KG, Zhang HJ, Wang J, et al. Effects of dietary pyrroloquinoline quinone disodium on growth performance, carcass yield and antioxidant status of broiler chicks. Animal. 2015;9:409–416. 10.1017/S1751731114002328
- Naito Y, Kumazawa T, Kino I, Suzuki O. Effects of pyrroloquinoline quinone (PQQ) and PQQ-oxazole on DNA synthesis of cultured human fibroblasts. Life Sci. 1993;52:1909–1915.10.1016/0024-3205(93)90631-C
- Kumazawa T, Hiwasa T, Takiguchi M, Suzuki O, Sato K. Activation of Ras signaling pathways by pyrroloquinoline quinone in NIH3T3 mouse fibroblasts. Int. J. Mol. Med. 2007;19:765–770.
- Kimura K, Takada M, Ishii T, Tsuji-Naito K, Akagawa M. Pyrroloquinoline quinone stimulates epithelial cell proliferation by activating epidermal growth factor receptor through redox cycling. Free Radic. Biol. Med. 2012;53:1239–1251.10.1016/j.freeradbiomed.2012.07.015
- McKay MM, Morrison DK. Integrating signals from RTKs to ERK/MAPK. Oncogene. 2007;26:3113–3121. 10.1038/sj.onc.1210394
- Lee SR, Kwon KS, Kim SR, Rhee SG. Reversible inactivation of protein-tyrosine phosphatase 1B in A431 cells stimulated with epidermal growth factor. J. Biol. Chem. 1998;273:15366–15372. 10.1074/jbc.273.25.15366
- Iwamoto N, Sumi D, Ishii T, et al. Chemical knockdown of protein-tyrosine phosphatase 1B by 1,2-naphthoquinone through covalent modification causes persistent transactivation of epidermal growth factor receptor. J. Biol. Chem. 2007;282:33396–33404. 10.1074/jbc.M705224200
- Chiarugi P, Cirri P. Redox regulation of protein tyrosine phosphatases during receptor tyrosine kinase signal transduction. Trends Biochem. Sci. 2003;28:509–514. 10.1016/S0968-0004(03)00174-9
- Kenner KA, Anyanwu E, Olefsky JM, Kusari J. Protein-tyrosine phosphatase 1B is a negative regulator of insulin- and insulin-like growth factor-i-stimulated signaling. J. Biol. Chem. 1996;271:19810–19816. 10.1074/jbc.271.33.19810
- Chang Y, Ceacareanu B, Zhuang D, et al. Arterioscler. Counter-regulatory function of protein tyrosine phosphatase 1B in platelet-derived growth factor- or fibroblast growth factor-induced motility and proliferation of cultured smooth muscle cells and in neointima formation. Thromb. Vasc. Biol. 2006;26:501–507.
- Nakamura Y, Patrushev N, Inomata H, et al. Role of protein tyrosine phosphatase 1B in vascular endothelial growth factor signaling and cell-cell adhesions in endothelial cells. Circ. Res. 2008;102:1182–1191.10.1161/CIRCRESAHA.107.167080
- Shibata T, Nakahara H, Kita N, et al. A food-derived synergist of NGF signaling: identification of protein tyrosine phosphatase 1B as a key regulator of NGF receptor-initiated signal transduction. J. Neurochem. 2008;107:1248–1260.10.1111/jnc.2008.107.issue-5
- Garber AJ. Obesity and type 2 diabetes: which patients are at risk? Diabetes Obes. Metab. 2012;14:399–408.10.1111/dom.2012.14.issue-5
- Saltiel AR. New perspectives into the molecular pathogenesis and treatment of type 2 diabetes. Cell. 2001;104:517–529.10.1016/S0092-8674(01)00239-2
- van Tienen FH, Praet SF, de Feyter HM, et al. Physical activity is the key determinant of skeletal muscle mitochondrial function in type 2 diabetes. J. Clin. Endocrinol. Metab. 2012;97:3261–3269.10.1210/jc.2011-3454
- Rabøl R, Boushel R, Dela F. Mitochondrial oxidative function and type 2 diabetes. Appl. Physiol. Nutr. Metab. 2006;31:675–683.10.1139/h06-071
- Meex RC, Schrauwen-Hinderling VB, Moonen-Kornips E, et al. Restoration of muscle mitochondrial function and metabolic flexibility in type 2 diabetes by exercise training is paralleled by increased myocellular fat storage and improved insulin sensitivity. Diabetes. 2010;59:572–579.10.2337/db09-1322
- Stites T, Storms D, Bauerly K, et al. Pyrroloquinoline quinone modulates mitochondrial quantity and function in mice. J. Nutr. 2006;136:390–396.
- Bauerly K, Harris C, Chowanadisai W, et al. Altering pyrroloquinoline quinone nutritional status modulates mitochondrial, lipid, and energy metabolism in rats. PLoS One. 2011;6:e21779.10.1371/journal.pone.0021779
- Tao R, Karliner JS, Simonis U, et al. Pyrroloquinoline quinone preserves mitochondrial function and prevents oxidative injury in adult rat cardiac myocytes. Biochem. Biophys. Res. Commun. 2007;363:257–262. 10.1016/j.bbrc.2007.08.041
- Handschin C, Spiegelman BM. Peroxisome proliferator-activated receptor γ coactivator 1 coactivators, energy homeostasis, and metabolism. Endocr. Rev. 2006;27:728–735. 10.1210/er.2006-0037
- Feige JN, Auwerx J. Transcriptional coregulators in the control of energy homeostasis. Trends Cell Biol. 2007;17:292–301. 10.1016/j.tcb.2007.04.001
- Chowanadisai W, Bauerly KA, Tchaparian E, Wong A, Cortopassi GA, Rucker RB. Pyrroloquinoline quinone stimulates mitochondrial biogenesis through cAMP response element-binding protein phosphorylation and increased PGC-1α expression. J Biol Chem. 2010;285:142–152. 10.1074/jbc.M109.030130
- Byon JC, Kusari AB, Kusari J. Protein-tyrosine phosphatase-1B acts as a negative regulator of insulin signal transduction. Mol. Cell. Biochem. 1998;182:101–108. 10.1023/A:1006868409841
- Ahmad F, Azevedo JL, Cortright R, Dohm GL, Goldstein BJ. Alterations in skeletal muscle protein-tyrosine phosphatase activity and expression in insulin-resistant human obesity and diabetes. J. Clin. Invest. 1997;100:449–458. 10.1172/JCI119552
- Cheung A1, Kusari J, Jansen D, Bandyopadhyay D, Kusari A, Bryer-Ash M. Marked impairment of protein tyrosine phosphatase 1B activity in adipose tissue of obese subjects with and without type 2 diabetes mellitus. J. Lab. Clin. Med. 1999;134:115–123.10.1016/S0022-2143(99)90115-4
- Cheung A1, Kusari J, Jansen D, Bandyopadhyay D, Kusari A, Bryer-Ash M. Marked impairment of protein tyrosine phosphatase 1B activity in adipose tissue of obese subjects with and without type 2 diabetes mellitus. Diabetes. 2012;61:1415–1422.
- Zabolotny JM, Haj FG, Kim YB, et al. Transgenic overexpression of protein-tyrosine phosphatase 1B in muscle causes insulin resistance, but overexpression with leukocyte antigen-related phosphatase does not additively impair insulin action. J. Biol. Chem. 2004;279:24844–24851.10.1074/jbc.M310688200
- Klaman LD, Boss O, Peroni OD, et al. Increased energy expenditure, decreased adiposity, and tissue-specific insulin sensitivity in protein-tyrosine phosphatase 1B-deficient mice. Mol. Cell Biol. 2000;20:5479–5489. 10.1128/MCB.20.15.5479-5489.2000
- Zinker BA, Rondinone CM, Trevillyan JM, et al. PTP1B antisense oligonucleotide lowers PTP1B protein, normalizes blood glucose, and improves insulin sensitivity in diabetic mice. Proc. Natl. Acad. Sci. USA. 2002;99:11357–11362. 10.1073/pnas.142298199
- Popov D. Novel protein tyrosine phosphatase 1B inhibitors: interaction requirements for improved intracellular efficacy in type 2 diabetes mellitus and obesity control. Biochem. Biophys. Res. Commun. 2011;410:377–381. 10.1016/j.bbrc.2011.06.009
- Takada M, Sumi M, Maeda A, et al. Pyrroloquinoline quinone, a novel protein tyrosine phosphatase 1B inhibitor, activates insulin signaling in C2C12 myotubes and improves impaired glucose tolerance in diabetic KK-Ay mice. Biochemn. Biophys. Res. Commun. 2012;428:315–320. 10.1016/j.bbrc.2012.10.055
- Miyauchi K, Urakami T, Abeta H, Shi H, Noguchi N, Niki E. Action of pyrroloquinolinequinol as an antioxidant against lipid peroxidation in solution. Antiox. Redox. Signal. 1994;1:547–554.
- He K, Nukada H, Urakami T, Murphy MP. Antioxidant and pro-oxidant properties of pyrroloquinoline quinone (PQQ): implications for its function in biological systems. Biochem. Pharmacol. 2003;65:67–74.10.1016/S0006-2952(02)01453-3
- Ouchi A, Nakano M, Nagaoka S, Mukai K. Kinetic study of the antioxidant activity of pyrroloquinolinequinol (PQQH2, a reduced form of pyrroloquinolinequinone) in micellar solution. J. Agric. Food Chem. 2009;57:450–456.10.1021/jf802197d
- Mukai K, Ouchi A, Nakano M. Kinetic study of the quenching reaction of singlet oxygen by pyrroloquinolinequinol (PQQH2, a reduced form of pyrroloquinolinequinone) in micellar solution. J. Agri. Food Chem. 2011;59:1705–1712.10.1021/jf104420y
- Ouchi A, Ikemoto K, Nakano M, Nagaoka S, Mukai K. Kinetic study of aroxyl radical scavenging and α-tocopheroxyl regeneration rates of pyrroloquinolinequinol (PQQH2, a reduced form of pyrroloquinolinequinone) in dimethyl sulfoxide solution: finding of synergistic effect on the reaction rate due to the coexistence of α-tocopherol and PQQH2. J. Agric. Food Chem. 2013;61:11048–11060. 10.1021/jf4040496
- Hara H, Hiramatsu H, Adachi T. Pyrroloquinoline quinone is a potent neuroprotective nutrient against 6-hydroxydopamine-induced neurotoxicity. Neurochem. Res. 2007;32:489–495.10.1007/s11064-006-9257-x
- Nunome K, Miyazaki S, Nakano M, Iguchi-Ariga S, Ariga H. Pyrroloquinoline quinone prevents oxidative stress-induced neuronal death probably through changes in oxidative status of DJ-1. Biol. Pharm. Bull. 2008;31:1321–1326. 10.1248/bpb.31.1321
- Zhu B, Zhou H, Teerlink JR, Karliner JS. Pyrroloquinoline quinone (PQQ) decreases myocardial infarct size and improves cardiac function in rat models of ischemia and ischemia/reperfusion. Cardiovasc. Drugs. Ther. 2004;18:421–431. 10.1007/s10557-004-6219-x
- Zhu B, Simonis U, Cecchini G, et al. Comparison of pyrroloquinoline quinone and/or metoprolol on myocardial infarct size and mitochondrial damage in a rat model of ischemia/reperfusion injury. J. Cardiovasc. Pharm. Ther. 2006;11:119–128.10.1177/1074248406288757
- Jensen FE, Gardner GJ, Williams AP, Gallop PM, Aizenman E, Rosenberg PA. The putative essential nutrient pyrroloquinoline quinone is neuroprotective in a rodent model of hypoxic/ischemic brain injury. Neuroscience. 1994;62:399–406. 10.1016/0306-4522(94)90375-1
- Zhang Y, Feustel P, Kimelberg HK. Neuroprotection by pyrroloquinoline quinone (PQQ) in reversible middle cerebral artery occlusion in the adult rat. Brain Res. 2006;1094:200–206. 10.1016/j.brainres.2006.03.111
- Yamaguchi K, Sasano A, Urakami T, Tsuji T, Kondo K. Stimulation of nerve growth factor production by pyrroloquinoline quinone and its derivatives in vitro and in vivo. Biosci. Biotech. Biochem. 1993;57:1231–1233. 10.1271/bbb.57.1231
- Murase K, Hattori A, Kohno M, Hayashi K. Stimulation of nerve growth factor synthesis/secretion in mouse astroglial cells by coenzymes. Biochem. Mol. Biol. Internat. 1993;30:615–621.
- Yamaguchi K, Tsuji T, Uemura D, Kondo K. Cyclooxygenase induction is essential for NGF synthesis enhancement by NGF inducers in L-M cells. Biosci. Biotech. Biochem. 1996;60:92–94.10.1271/bbb.60.92
- Ohwada K, Takeda H, Yamazaki M, et al. Pyrroloquinoline quinone (PQQ) prevents cognitive deficit caused by oxidative stress in rats. J. Clin. Biochem. Nutr. 2008;42:29–34.10.3164/jcbn.2008005
- Takatsu H, Owada K, Abe K, Nakano M, Urano S. Effect of Vitamin E on learning and memory deficit in aged rats. J. Nutr. Sci. Vitaminol. 2009;55:389–393.10.3177/jnsv.55.389
- Koikeda T, Nakano M, Masuda K. Pyrroloquinoline quinone disodium salt improves higher brain function. Med. Consult. New Remedies. 2011;48:519–527.
- Randolph C, Tierney MC, Mohr E, Chase TN. The repeatable battery for the assessment of neuropsychological status (RBANS): preliminary clinical validity. J. Clin. Exp. Neuropsychol. 1989;20:310–319.
- Itoh Y, Hine K, Miura H, et al. Effect of the antioxidant supplement pyrroloquinoline quinone disodium salt (BioPQQ™) on cognitive functions. Adv. Exp. Med. Biol. in press
- Hakoda Y, Sasaki M. Group version of the stroop and reverse-stroop test–the effects of reaction mode, order and practice–jap. J. Educational Psychol. 1990;38:389–394 (in Japanese).
- Hayashi Y, Kijima T, Satou K, Murakami S. Examination of the evaluation method of visual-spatial cognitive function using the touch screen device. Jap. J. Geria. Psychiatry. 2011;22:439–447 (in Japanese).
- Nakano M, Yamamoto T, Okumura H, Tsuda A, Kowatari Y. Effects of oral supplementation with pyrroloquinoline quinone on stress, fatigue, and sleep. Funct. Foods Health Dis. 2012;2:307–324.
- Nakano M, Kamimura A, Watanabe F, et al. Effects of orally administered pyrroloquinoline quinone disodium salt on dry skin conditions in mice and healthy female subjects. J. Nutr. Sci. Vitaminol. 2015;61:242–247.
- Nakano M, Kawasaki Y, Suzuki N, Takara T. Effects of pyrroloquinoline quinone disodium salt intake on the serum cholesterol levels of healthy Japanese adults. J. Nutr. Sci. Vitaminol. 2015;61:234–241.
- Nakano M, Takahashi H, Koura S, Chung C, Tafazoli S, Roberts A. Acute and subchronic toxicity studies of pyrroloquinoline quinone (PQQ) disodium salt (BioPQQ™) in rats. Regul. Toxicol. Pharmacol. 2014;70:107–121. 10.1016/j.yrtph.2014.06.024
- Liang C, Zhang X, Wang W, Song Y, Jia X. A subchronic oral toxicity study on pyrroloquinoline quinone (PQQ) disodium salt in rats. Food Chem. Toxicol. 2015;75:146–150.10.1016/j.fct.2014.11.005
- Nakano M, Suzuki H, Imamura T, Lau A, Lynch B. Genotoxicity of pyrroloquinoline quinone (PQQ) disodium salt (BioPQQ™). Regul. Toxicol. Pharmacol. 2013;67:189–197.10.1016/j.yrtph.2013.07.007
- Rucker R, Chowanadisai E, Nakano M. Potential physiological importance of pyrroloquinoline quinone. Altern. Med. Res. 2009;14:268–277.
- Smidt CR, Unkefer CJ, Houck DR, Rucker RB. Intestinal absorption and tissue distribution of [14C]pyrroloquinoline Quinone in mice. Proc. Soc. Exp. Biol. Med. 1991;197:27–31.10.3181/00379727-197-43219
- Watanabe A, Hobara N, Ohsawa T, Higashi T, Tsuji T. Nephrotoxicity of pyrroloquinoline quinone in rats. Hiroshima J. Med. Sci. 1989;38:49–51.