Abstract
Trehalose acts as a stress protectant and an autophagy inducer in mammalian cells. The molecular mechanisms of action remain obscure because intracellular trehalose at micromolar level is difficult to quantitate. Here, we show a novel trehalose monitoring technology based on FRET. FLIPsuc90μ∆1Venus sensor expressed in mammalian cells enables to quickly and non-destructively detect an infinitesimal amount of intracellular trehalose.
Trehalose, the non-reducing disaccharide of glucose molecules linked by an α-1,1-bond, is available as the main carbon source in micro-organisms and invertebrates.Citation1,2) It also possesses highly important properties as a chemical chaperon to prevent aggregation of proteins in conditions of stresses, such as heating, freezing, and desiccation, and it can act as an enhancer of the mTOR-independent autophagy pathway. Thanks to these properties, trehalose alleviates symptoms caused by abnormal protein aggregation, such as Huntington disease, Alzheimer disease, and prion disease in cellular and animal models.Citation3–5) The effective concentration of trehalose in cells is a range from 1 μM to 100 mM. For example, high levels of intracellular trehalose (~20 mM) confer desiccation tolerance to Chinese hamster ovarian (CHO) and hepatoma cellsCitation6,7), whereas low levels (10–500 μM) effectively inhibit aggregation involved in a neurological disorder, spinocerebeller ataxia type 14.Citation8) To study the biological function of trehalose in detail, accurate quantification of the actual cytosolic concentration of trehalose is necessary. High trehalose levels can be measured by conventional detection systems for carbohydrates, such as an HPLC equipped with sugar separation columns;Citation9,10) however, this method is unsuitable for microscale determination of trehalose because the minimum detectable quantity is ≥1 mM. In addition, for accurate quantification, loss of trehalose due to enzymatic or chemical degradation in the process of extraction from the cells has to be minimized. Hence, highly sensitive and non-destructive quantification systems for trehalose in cells are desirable to overcome these difficulties.
Genetically encoded Förster Resonance Energy Transfer (FRET)-based sensors provide an attractive molecular recognition tool with high spatiotemporal resolution and sensitivity in individual cells, and are capable of quantifying and visualizing small molecules such as sugars, ions, amino acids, and other metabolites.Citation11–14) FRET sensors show spectral changes in fluorescence as a consequence of analytes to a ligand binding protein fused to two fluorophores.Citation15) The FLIPSuc-YFP sensor (alternative acceptor, VenusCitation16)) was engineered by fusion of a FRET pair of fluorescent proteins to the N- and C-terminus of the bacterial periplasmic binding protein, AtThuE, from Agrobacterium.Citation17) The original sucrose sensor, FLIPSuc4μ was able to detect disaccharides such as maltose, turanose, and trehalose.Citation17) The improved sucrose sensor FLIPSuc90μ∆1Venus showed increased selectivity for sucrose. Here, we show that FLIPSuc90μ∆1Venus recognizes trehalose with similar affinity as sucrose. Previous studies using this sensor had shown that sucrose did not trigger spectral changes in various human cell types expressing FLIPSuc90μ∆1Venus indicating that the intrinsic sucrose uptake activities in the cells were below the detection levels.Citation18) The sensors were functional in human cells because they responded in cells co-expressing sucrose transporters of the plant SWEET family.Citation18) To detect intracellular trehalose using the FRET sensor, we co-expressed the trehalose transporter 1 (PvTRET1) which mediates trehalose influx into the cells by facilitated diffusion across the membranes down sugar concentration gradients.Citation9) In the present study, we characterize the properties of the sensor and perform continuous trehalose monitoring in human cell lines.
The genetically encoded FRET-based sucrose sensor, FLIPSuc90μ∆1YFP-pRSET-B was obtained from Addgene (No. 14475). The eYFP was replaced with mVenus obtained from the Miyawaki laboratory (Riken, Japan) as an alternative acceptor.Citation16) The construct of FLIPSuc90μ∆1Venus was transferred into Escherichia coli BL21 (DE3) for bacterial protein expression. Sensor protein purifications had been described previously.Citation15) Sensors were purified using Ni–NTA chromatography (Profinity™ IMAC Ni-charged Resin, Bio-Rad, Hercules, CA). In vitro analyses were performed with different concentrations of trehalose in 20 mM sodium phosphate buffer, pH 7 at 25 °C. Trehalose titration curves were obtained using a fluorescence plate reader (MTP-601Lab, Hitachi High-Technologies Corp., Tokyo, Japan) with excitation at 433 nm and emission maxima of 485 and 528 nm for CFP and Venus, respectively (half band width 15 nm). The ratio of Venus/CFP fluorescence intensity was determined. The Kd of FLIPSuc90μ∆1Venus for trehalose was determined by fitting ligand titration curves to a single-site binding isotherm: S = (r–rapo)/rsat–rapo) = [L]/(Kd)+[L]). S means saturation, [L] is the trehalose concentration, r is the ratio of observed fluorescence between Venus and CFP, rapo is the ratio under trehalose-free condition, and rsat is the ratio at saturation. The biological samples were prepared in three technical replicates. The Kd values were analyzed using PRISM 6 (GraphPad Software, La Jolla, CA). To test whether the sucrose sensor can report trehalose levels, we expressed FLIPSuc90μ∆1Venus in E. coli and purified the sensor and examined the affinity for trehalose in vitro. FLIPSuc90μ∆1Venus proteins were then transferred to microtiter plates containing various trehalose concentrations and the Venus/CFP ratio were measured (Fig. ). The fitting ligand titration curve was characterized by a Kd of 100.3 ± 16.9 μM for trehalose, which is similar to that for sucrose determined in previous in vitro analyses.Citation17) We conclude that FLIPSuc90μ∆1Venus allows detection of trehalose in a range from ~10 to ~1000 μM.
Fig. 1. In vitro characterization of FLIPSuc90µ∆1Venus for trehalose.
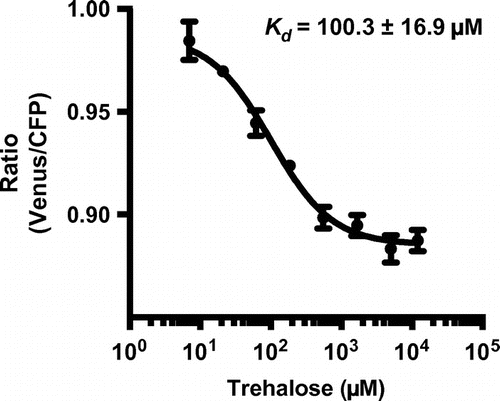
The pPvTRET1-IRES2-AcGFP1 (PvTRET1 accession No. AB272983) was previously constructed in a mammalian cell expression vector.Citation9) The vector was digested with Bam HI and Not I restriction enzymes to remove the region encoding AcGFP1, and filled in both of the digested termini with Klenow polymerase. pPvTRET1-IRES2 was created by self-ligation of the treated fragment. An amplified DNA fragment for FLIPSuc90μ∆1Venus with primers (forward primer, 5′-ATG CGG GGT TCT CAT CAT CAT C-3′ and reverse primer, 5′-TTA CTT GTA CAG CTC GTC CAT G-3′) was directly integrated into the mammalian cell expression vector pcDNA3.1/V5-His (Life technologies, Carlsbad, CA).
Human embryonic kidney 293T (HEK293T) cells were cultured in D-MEM (Sigma-Aldrich, St. Louis, MO) supplemented with 10% FBS, 100 units/ml penicillin, and 100 μg/ml streptomycin (Sigma-Aldrich) at 37 °C, an atmosphere of 5% CO2, and 95% relative humidity. HEK293T cells were seeded on the 35 mm cell culture dishes, and incubated for 24 h. FLIPSuc90μ∆1Venus-pcDNA3.1 and pPvTRET1-IRES2 were co-transfected to the cells using Opti-MEM® (Life technologies) containing the transfection reagent, FuGENE® HD (Promega, Madison, WI) according to the manufactural protocol. The fluorescent imaging analyses were performed at 48 h after the transfection. HEK293T cells expressing FLIPSuc90μ∆1Venus without PvTRET1 were analyzed for the endogenous disaccharide uptake activities.
The FRET analysis system equipped with a circulatory buffer flow system was described previously.Citation19) Cells co-expressing sensor and transporter were cultured on glass bottom dishes or glass coverslips. Before the sugar monitoring assays, cells were rinsed with Dulbecco’s PBS (Sigma-Aldrich), then media were replaced with fresh Hank’s buffered saline solution (HBSS; combining 137 mM NaCl, 5.4 mM KCl, 0.3 mM Na2HPO4, 0.4 mM KH2PO4, 4.2 mM NaHCO3, 1.3 mM CaCl2, 0.5 mM MgCl2, and 0.4 mM MgSO4, pH 7.4). The cytoplasmic fluorescent emission intensities were monitored under the excitation of a confocal microscopy (TCS SP8, Leica microsystems, Germany) installed with filters (CFP excitation 430/20, CFP emission: 475/20, Venus emission: 530/15), using a 40x objective (HC PL APO CS2, 40x/1.30 oil). The pinhole size was set to 65.3 μm. Cells were perfused with HBSS containing 5–40 mM disaccharides. Imaging data were analyzed using the software, Leica Application Suite Advanced Fluorescence (LAS AF, Leica). Fluorescence intensities of the cytoplasmic Venus/CFP ratio in HEK293T cells expressing FLIPSuc90μ∆1Venus were monitored during the addition of trehalose to the chamber (Fig. (A)). No significant changes in the fluorescence intensity ratio were detected in the cells, indicating that intrinsic trehalose influx was not induced in HEK293T cells. Thus, endogenous trehalose uptake activities in HEK293T cells must be below the range detectable by the sensor. These findings are consistent with results from Xenopus oocytes and mammalian cells indicating low or no significant uptake capacity for trehalose.Citation9,10) To corroborate these findings, we co-expressed PvTRET1, which can promote trehalose influx into cells with the FLIPSuc90μ∆1Venus sensor. When the fluorescence intensity ratio was monitored during the addition of trehalose to the buffer, rapid changes in the intensity ratio was detected immediately following trehalose addition (Fig. (A)). The FRET sensor enabled to detect and visualize intracellular trehalose in individual human cells (Fig. (B)), Supplemental movie 1). The maximum of fluorescent ratio changes of the sensor caused by PvTRET1-mediated uptake of trehalose were reached after 0.25 min, indicating that PvTRET1 is capable of rapid uptake. The advanced monitoring tool for trehalose would be possible to detect a small amount of ones in a few seconds and in localized spaces such as nucleus, cytoplasmic organella, or transmembranes.
Fig. 2. Intracellular trehalose monitoring by FLIPSuc90µ∆1Venus.
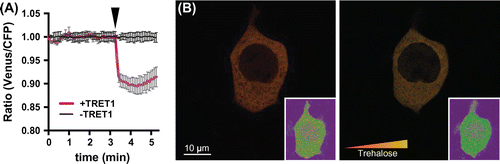
We monitored temporal trehalose levels in the individual cells expressing the FRET sensor equipped with the trehalose perfusion system, and also evaluated transport activities by PvTRET1 (Fig. (A)). PvTRET1 appears to function as a uniporter.Citation9) Moreover, human kidney cells such as HEK293T express trehalase activity.Citation20) We therefore tested whether the trehalose accumulation was reversible. Removal of substrate after addition showed clear reversibility, which can be due to either metabolism or efflux (Fig. (A)). Intracellular trehalose concentrations are calculated as follows: [Trehalose]i = Kd (r–rmin)/(rmax–r). Kd value was obtained in the analysis under in vitro conditions as indicated above, r is the measured ratio, rmin is the minimum ratio with unbound form of FLIPSuc90μ∆1Venus, and rmax is the maximum ratio change of the sensor. The sensor response was concentration-dependent. Full saturation was obtained at an external supply of 40 mM, half saturation at 5–10 mM. Given that the sensor itself has a Kd for trehalose of ~100 μM, uptake of trehalose is by PvTRET1 is limiting, or incoming trehalose is at least in part metabolized. The intracellular trehalose concentration was estimated based on the assumption that the sensor is saturated at a supply level of 40 mM, and that the sensor affinity in the cytosol of HEK293T cells is comparable to that in vitro (Fig. B). When cell were perfused with 5, 10, and 40 mM trehalose, the calculated concentrations of intracellular trehalose were 84.4 ± 0.3 μM, 245.8 ± 2.5 μM, and 2.6 ± 0.3 mM, respectively. The present study demonstrates that the sucrose sensor FLIPSuc90μ∆1Venus recognizes trehalose with approximately equal affinity as sucrose. We also demonstrate that the sensor is suitable for monitoring cytosolic trehalose accumulation and elimination with high temporal and spatial resolution.
Fig. 3. Sensitivity of FLIPSuc90µ∆1Venus in cytoplasm.

We propose that the FRET sensor is applicable for studying the physiological role of trehalose under various conditions such as the alleviating effect of the improper ordered protein aggregates or prevention of osteoporosis.Citation8,21)
Author contribution
S.K., W.B.F., and T.K. designed research; S.K., B.-H.·H., and T.K. performed research; S.K., B-H·H., R.S., W.B.F., and T.K. analyzed data; S.K., W.B.F., and T.K. wrote the paper.
Supplemental material
The supplemental material for this paper is available at http://dx.doi.org/10.1080/09168451.2015.1069699.
Disclosure statement
No potential conflict of interest was reported by the authors.
Funding
This work was supported by Japan Society for the Promotion of Science (JSPS) KAKENHI [grant number 12J110839] and [grant number 26850216] for SK and the Division of Chemical Sciences, Geosciences and Biosciences, Office of Basic Energy Sciences at the US Department of Energy (DOE) under [grant number DE-FG02–04ER15542]. The mVenus construct was obtained from Miyawaki Lab. (Riken BSI, Saitama, Japan). HEK293T cells were provided from RIKEN Cell Bank (Tsukuba, Japan).
Series005RATIO_v2.avi
Download Microsoft Video (AVI) (7 MB)Acknowledgments
We thank Tomoe Shiratori for maintenance of HEK293T cells. We would like to thank Dr Tsuyoshi Tanaka and Mr Toru Honda at Tokyo University of Agriculture and Technology (Tokyo, Japan) in in vitro analyses using the fluorescence plate reader.
Notes
Abbreviations: FRET, Förster Resonance Energy Transfer; FBS, fetal bovine serum; D-MEM, Dulbecco’s modified Eagle medium; HBSS, Hank’s buffered saline solution; HEK293T, human embryonic kidney 293T; TRET1, trehalose transporter 1.
References
- Argüelles JC. Physiological roles of trehalose in bacteria and yeasts: a comparative analysis. Arch. Microbiol. 2000;174:217–224.
- Wyatt G. The biochemistry of insect hemolymph. Annu. Rev. Entomol. 1961;6:75–102.10.1146/annurev.en.06.010161.000451
- Crowe JH, Hoekstra FA, Crowe LM. Anhydrobiosis. Annu. Rev. Physiol. 1992;54:579–599.10.1146/annurev.ph.54.030192.003051
- Sarkar S, Davies JE, Huang Z, Tunnacliffe A, Rubinsztein DC. Trehalose, a novel mTOR-independent autophagy enhancer, accelerates the clearance of mutant huntingtin and α-Synuclein. J. Biol. Chem. 2007;282:5641–5652.10.1074/jbc.M609532200
- Sakurai M, Furuki T, Akao K, et al. Vitrification is essential for anhydrobiosis in an African chironomid, Polypedilum vanderplanki. Proc. Natl. Acad. Sci. 2008;105:5093–5098.10.1073/pnas.0706197105
- Chakraborty N, Menze MA, Elmoazzen H, et al. Trehalose transporter from African chironomid larvae improves desiccation tolerance of Chinese hamster ovary cells. Cryobiology. 2012;64:91–96.10.1016/j.cryobiol.2011.11.007
- Li S, Chakraborty N, Borcar A, Menze MA, Toner M, Hand SC. Late embryogenesis abundant proteins protect human hepatoma cells during acute desiccation. Proc. Natl. Acad. Sci. 2012;109:20859–20864.10.1073/pnas.1214893109
- Seki T, Abe-Seki N, Kikawada T, et al. The effect of trehalose on the properties of mutant γPKC, which causes spinocerebellar ataxia type 14 (SCA14), in neuronal cell lines and cultured Purkinje cells. J. Biol. Chem. 2010;285:33252–33264.10.1074/jbc.M110.146704
- Kikawada T, Saito A, Kanamori Y, et al. Trehalose transporter 1, a facilitated and high-capacity trehalose transporter, allows exogenous trehalose uptake into cells. Proc. Natl. Acad. Sci. 2007;104:11585–11590.10.1073/pnas.0702538104
- Kanamori Y, Saito A, Hagiwara-Komoda Y, et al. The trehalose transporter 1 gene sequence is conserved in insects and encodes proteins with different kinetic properties involved in trehalose import into peripheral tissues. Insect Biochem. Mol. Biol. 2010;40:30–37.10.1016/j.ibmb.2009.12.006
- San Martín A, Ceballo S, Ruminot I, Lerchundi R, Frommer WB, Barros LF. A genetically encoded FRET lactate sensor and its use to detect the Warburg effect in single cancer cells. PLoS ONE. 2013;8:e57712.10.1371/journal.pone.0057712
- Okumoto S, Looger LL, Micheva KD, Reimer RJ, Smith SJ, Frommer WB. Detection of glutamate release from neurons by genetically encoded surface-displayed FRET nanosensors. Proc. Natl. Acad. Sci. 2005;102:8740–8745.10.1073/pnas.0503274102
- Miyawaki A, Llopis J, Heim R, et al. Fluorescent indicators for Ca2+ based on green fluorescent proteins and calmodulin. Nature. 1997;388:882–887.
- Heim R, Tsien RY. Engineering green fluorescent protein for improved brightness, longer wavelengths and fluorescence resonance energy transfer. Curr. Biol. 1996;6:178–182.10.1016/S0960-9822(02)00450-5
- Fehr M, Frommer WB, Lalonde S. Visualization of maltose uptake in living yeast cells by fluorescent nanosensors. Proc. Natl. Acad. Sci. 2002;99:9846–9851.10.1073/pnas.142089199
- Nagai T, Ibata K, Park ES, Kubota M, Mikoshiba K, Miyawaki A. A variant of yellow fluorescent protein with fast and efficient maturation for cell-biological applications. Nat. Biotechnol. 2002;20:87–90.10.1038/nbt0102-87
- Lager I, Looger LL, Hilpert M, Lalonde S, Frommer WB. Conversion of a putative Agrobacterium sugar-binding protein into a FRET sensor with high selectivity for sucrose. J. Biol. Chem. 2006;281:30875–30883.10.1074/jbc.M605257200
- Chen L-Q, Qu X-Q, Hou B-H, et al. Sucrose efflux mediated by SWEET proteins as a key step for phloem transport. Science. 2012;335:207–211.10.1126/science.1213351
- Hou B-H, Takanaga H, Grossmann G, et al. Optical sensors for monitoring dynamic changes of intracellular metabolite levels in mammalian cells. Nat. Protocols. 2011;6:1818–1833.10.1038/nprot.2011.392
- Robinson PS, Goochee CF. Kidney-specific enzyme expression by human kidney cell lines generated through oncogene transfection. J. Cell Physiol. 1991;148:54–59.10.1002/(ISSN)1097-4652
- Nishizaki Y, Yoshizane C, Toshimori Y, et al. Disaccharide-trehalose inhibits bone resorption in ovariectomized mice. Nutr. Res. 2000;20:653–664.10.1016/S0271-5317(00)00155-X