Abstract
N,N,N-trimethyl phytosphingosine-iodide (TMP) was recently developed as an antitumor agent. We examined the effects of TMP on melanogenesis and its related signaling pathways in normal human melanocytes. Our results showed that melanin is significantly reduced in a dose-dependent manner in both cells following liposomal TMP treatment. We also investigated changes in the phosphorylation of extracellular signal-regulated kinase (ERK), which is related to the degradation of microphthalmia-associated transcription factor (MITF). Our results indicated that liposomal TMP treatment leads to the phosphorylation of ERK, which reduces both MITF and tyrosinase protein levels. Treatment with PD98059, an ERK pathway-specific inhibitor, restored liposomal TMP-induced reductions in melanin, abrogated reductions in tyrosinase activity, and downregulated MITF and tyrosinase protein. In conclusion, these results suggest that the inhibitory effects of TMP on melanogenesis are due to MITF and tyrosinase downregulation via ERK activation.
Graphical abstract
Liposomal TMP treatment decreases the expression of both MITF and tyrosinase proteins
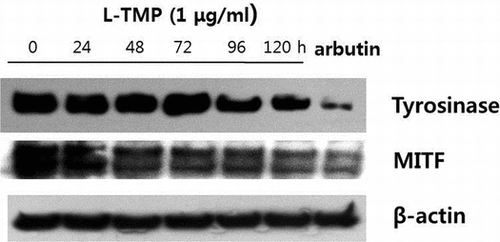
Melanin is produced by specialized organelles in melanocytes called melanosomes. In melanosomes, melanin pigment is synthesized by an enzymatic cascade that is controlled by tyrosinase, tyrosinase-related protein 1 (TRP-1), and tyrosinase-related protein 2 (TRP-2). Tyrosinase plays critical roles in melanogenesis because it catalyzes two rate-limiting steps: (1) the hydroxylation of tyrosine to 3,4-dihydroxyphenylalanine (DOPA), and (2) the oxidation of DOPA to dopaquinone.Citation1) While tyrosinase regulates the rate-limiting steps, TRP-1 and TRP-2 are responsible for modifying melanin.Citation1) Accordingly, many studies on hypomelanogenesis focus on tyrosinase activity under specific conditions. Microphthalmia-associated transcription factor (MITF) is a major transcriptional regulator of tyrosinase, TRP-1, and TRP-2.Citation1)
It is well known that melanogenesis is regulated by balancing a variety of signal transduction pathways, including cyclic adenosine monophosphate/protein kinase A (cAMP/PKA), p38 mitogen-activated protein kinase (p38 MAPK), extracellular signal-regulated kinase (ERK), and phosphoinositide 3-kinase/Akt (PI3K/Akt).Citation2–5) Activating ERK signaling downregulates melanogenesis by inhibiting MITF activity.Citation6) Activating ERK also phosphorylates the Ser73 residue of MITF by recruiting p300, a transcriptional coactivator. These processes lead to MITF ubiquitination and proteasome-mediated degradation.Citation7)
Phytosphingosine is structurally similar to sphingosine and a major component of the membranes produced by plants, fungi, and mammalian tissue.Citation8) Phytosphingosine demonstrates anticancer effects in Jurkat cells and NCI-H460, a human non-small cell lung cancer cell line.Citation9,10) N,N,N-trimethylphytosphingosine-iodide (TMP, Fig. ) was recently developed as an antitumor agent.Citation11) TMP is a novel and potent inhibitor of angiogenesis and metastasis in B16F10 murine melanoma cells and effectively inhibits in vitro cell migration, tube formation, and the expression of angiogenic factors.Citation11) Although TMP is an important molecule in many signaling pathways, no attempts have been made to investigate the effects of TMP on melanogenesis. Using liposomes that contain sphingosine derivatives and TMP as delivery carriers has already been proposed.Citation11) Here, we evaluate the in vitro effects of liposomal TMP (L-TMP) on the regulation of melanogenesis and examine the association between ERK phosphorylation and TMP-mediated hypomelanogenesis.
Materials and methods
Materials
TMP was purchased from Phytos Co., Ltd (Suwon, South Korea). Normal human melanocytes (NHMs) and cell culture reagents were purchased from Invitrogen (Eugene, OR). Antibodies specific to tyrosinase, ERK, p-ERK, p38, p-p38, PKC-β II (Santa Cruz Biotechnology, Inc., Santa Cruz, CA), microphthalmia (NeoMarkers, Fremont, CA), and β-actin (Sigma-Aldrich; St. Louis, MO) were used. All other chemicals were purchased from Sigma-Aldrich Co., unless otherwise indicated.
Preparation of L-TMP
Liposomes containing TMP were prepared by sonication. Briefly, TMP (10 and 100 µg/mL) and soybean lecithin (20 mg/mL) were dissolved in ethanol (10%). Poloxamer 407 (0.5%) and Tween 80 (0.5%) were dissolved in purified water, and this aqueous solution was slowly added to the ethanol solution with vigorous vortexing. The mixed solution was incubated for 30 min by vortexing, and the liposomal solution was then sonicated using a bath-type sonicator for 30 min. The TMP liposomal solution was filtered using a 0.22-µm filter.
Cell culture
NHMs were cultured in Medium 254 (M-254) supplemented with human melanocyte growth supplement. All cultures were fed twice weekly and incubated at 37 °C in a humidified atmosphere containing 5% CO2. NHMs were treated with compounds for 120 h before analysis because melanogenesis is a much longer process in these cells.
Cell viability testing
NHM viability was evaluated using the WST-1 assay. In brief, 5000 cells/well were placed in 96-well plates, supplemented with M-254 growth media, and incubated at 37 °C with 5% CO2 for 48 h. Then, the cells were treated with 0.1–10 μg/mL L-TMP for 48 h. WST-1 reagent (10 μL) was added to the culture medium, and absorbance was measured at 450 nm using a ELISA microplate reader (Molecular Devices, Sunnyvale, CA) after 2 h of incubation.
Melanin content assay
Intracellular melanin was measured as previously described.Citation12) NHMs were seeded onto 6-well tissue culture plates at densities of 2 × 105 and 5 × 105 cells/well, respectively, incubated for 24 h, and then treated with α-melanocyte-stimulating hormone (MSH) (100 nM). NHMs were treated for 120 h with increasing doses ranging between 0.1 and 1 μg/mL L-TMP, respectively. Cells were harvested, and the cell pellets were resuspended in 1 N NaOH for 30 min at 100 °C. Melanin absorbance at 405 nm was determined using an ELISA microplate reader. The specific melanin content was adjusted by the protein amount. In some studies, cells were treated with ERK inhibitors (10 μM PD98059) for 30 min prior to the addition of α-MSH.
Tyrosinase activity
Tyrosinase activity was determined as previously described with slight modifications.Citation13) Briefly, L-TMP-treated NHMs were washed with ice-cold phosphate-buffered saline (PBS) and lysed with phosphate buffer (pH 6.8) containing 1% Triton X-100. Cells were then disrupted by freezing and thawing, and the lysates were obtained by centrifugation at 10,000 g for 5 min. After quantifying the protein levels and adjusting the concentrations using lysis buffer, 90 µL of each lysate containing the same amount of protein was placed in a 96-well plate, and 10 µL of 10 mM L-DOPA was then added to each well. Following incubation at 37 °C, absorbance was measured every 10 min for at least 1 h at 475 nm using an ELISA reader. In some studies, cells were treated with an ERK inhibitor (10 μM PD98059) for 1 h prior to the addition of α-MSH. Cell-free tyrosinase activity was also determined as previously described with slight modifications.Citation14) A 70-μL volume of phosphate buffer containing L-TMP (0.1, 0.5, 1, 5, or 10 μg/mL) was mixed with 20 μL of 10 μg/mL mushroom tyrosinase, and 10 μL of 10 mM L-DOPA was added. Following incubation at 37 °C, absorbance was measured at 405 nm.
Measuring protein kinase C (PKC) activity
PKC activity was assessed using the CycLex® protein kinase C assay kit (CycLex Co., Nagano, Japan). The assay procedures were performed according to CycLex® PKC assay user manual. The PKC inhibitory effects of TMP on NHMs were measured using various concentrations of L-TMP (10, 25, 50, and 100 μg/mL).
Western blot analysis
The protein levels of tyrosinase and MITF in L-TMP-treated NHMs were determined using Western blot analysis. Cells were lysed in Pro-prep lysis buffer (Intron, Seongnam, Korea) using complete protease inhibitor cocktail (Roche, Mannheim, Germany). Protein concentrations were determined using the Bradford assay. Twenty micrograms of protein were separated using sodium dodecyl sulfate polyacrylamide gel electrophoresis (SDS–PAGE) and transferred onto nitrocellulose membranes, which were then saturated with 5% skin milk in tri-buffered saline containing 0.1% Tween 20 (TBST) for 1 h at room temperature. Blots were incubated with primary antibodies against tyrosinase (1:500 dilution), MITF (1:1000), ERK (1:1000), p-ERK (1:1000), p38 MAPK (1:500), p-p38 MAPK (1:500) and PKC-β II after washing with TBST, and all blots were also incubated with horseradish peroxidase-conjugated secondary antibody. Bound antibodies were detected using an enhanced chemiluminescence assay (Pierce, Rockford, IL). Membranes were reprobed with anti-β-actin antibody (diluted 1:5000).
Statistical analysis
The statistical significance of the differences between groups was assessed using analysis of variance (ANOVA), followed by the Student’s t-test. Here, p < 0.05 is considered statistically significant.
Results
Effects of L-TMP on cell viability
L-TMP cytotoxicity was evaluated using the WST-1 cell proliferation assay in order to confirm that the decreased tyrosinase activity and melanin content in the L-TMP-treated cells were not due to cytotoxic effects. There were no significant changes in our morphological assessments of NHMs exposed to 0–5 μg/mL L-TMP (Fig. (A)). According to the results of the WST-1 assay, no significant changes in cell viability were found at doses between 0 and 5 μg/mL L-TMP. Accordingly, 0.1–1 μg/mL L-TMP was used in the NHM experiments (Fig. (B)).
Effects of L-TMP on melanin synthesis in NHMs
L-TMP appeared to significantly whiten NHM pellets (Fig. (A)). L-TMP decreased the melanin content in NHMs (Fig. (B)). NHMs treated with 0.1–1 μg/mL L-TMP exhibited lower pigmentation in comparison with untreated control cells in a dose-dependent manner. We also measured melanin content in NHMs that were treated with arbutin (100 μg/mL). Similar inhibitory effects on melanogenesis were noted at 0.1 μg/ml L-TMP and arbutin. At concentrations >0.1 μg/mL, L-TMP caused a significantly higher reduction in the melanin content in comparison with arbutin (100 μg/mL).
Fig. 3. Effects of L-TMP on melanogenesis.
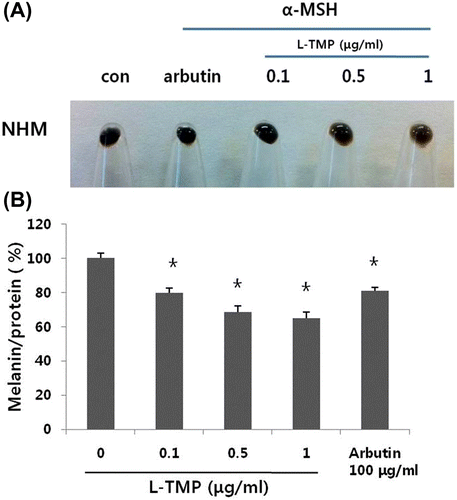
Effects of L-TMP on tyrosinase activity
Tyrosinase activity was assessed because it regulates the rate-limiting steps of melanogenesis. As shown in Fig. , we found that tyrosinase activity was decreased by L-TMP at certain concentrations. NHMs treated with 0.1–1 μg/mL L-TMP demonstrated significantly lower tyrosinase activity in comparison with untreated control cells (Fig. (A)). However, treating NHMs with L-TMP caused less remarkable reductions in tyrosinase activity than arbutin (100 μg/mL). To exclude the possibility of the direct influence of L-TMP on tyrosinase activity, we added L-TMP to mushroom tyrosinase and measured the in vitro tyrosinase activity. L-TMP did not directly regulate tyrosinase activity (Fig. (B)).
Fig. 4. Effects of L-TMP on tyrosinase activity. L-TMP suppressed tyrosinase activity in NHMs.
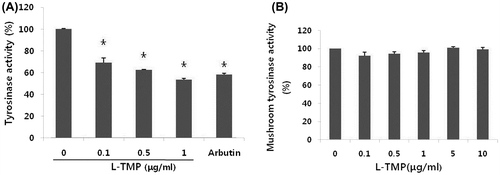
Effects of L-TMP on the expression of tyrosinase and MITF protein in NHMs
To investigate the possible mechanisms responsible for L-TMP-induced decreases in pigmentation, the inhibitory effects of L-TMP on the expression of MITF and tyrosinase in NHMs were also examined using Western blot analysis. As shown in Fig. (A), both MITF and tyrosinase protein expression were reduced following 120-h treatment with 1 μg/mL L-TMP. These results suggest that the downregulated expression of MITF and tyrosinase via L-TMP may be responsible for reduced melanin production.
Fig. 5. Effects of L-TMP on melanogenic protein expression and the ERK, p38 and PKC pathways in NHMs.
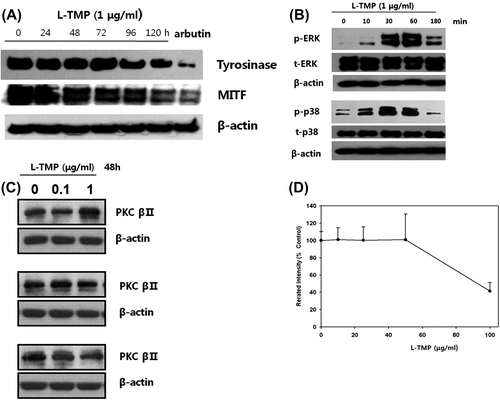
Effects of L-TMP on melanogenesis-related signaling pathways in NHMs
As shown in Fig. (B), the phosphorylation of ERK and p38 MAPK was induced in NHMs after 10 min of L-TMP treatment (1 μg/mL). These findings suggest that the inhibitory effects of L-TMP on melanin production are related to the phosphorylation of ERK and p38 kinase.
Effects of L-TMP on PKC activity in NHMs
The expression and activity of PKC was also assessed because PKC-β activates tyrosinase by phosphorylating the serine residues in its cytoplasmic domain.Citation15,16) As shown in Fig. (C), PKC-β protein expression did not change following a 48-h treatment with 0–1 μg/mL L-TMP. PKC activity was not altered by treatment with 0–40 μg/mL L-TMP (Fig. (D)).
Inhibiting the ERK pathway using PD98059 abrogates L-TMP-induced hypopigmentation
We also assessed if the ERK pathway-specific inhibitor, PD98059, prevents the L-TMP-induced reduction in melanin synthesis in NHMs. NHMs were cultured for 120 h with 1 μg/mL L-TMP in the absence or presence of 10 μM PD98059. Fig. (A) shows that treatment with PD98059 blocked L-TMP-induced ERK phosphorylation. As shown in Fig. (B), cells treated with L-TMP exhibited lower pigmentation than control cells, but L-TMP-induced hypopigmentation was restored by PD98059 pretreatment. Reduced tyrosinase activity by L-TMP treatment was also restored when NHMs were treated with PD98059 (Fig. (C)). Furthermore, we investigated the expression levels of melanogenic proteins following PD98059 treatment. We found that PD98059 prevented L-TMP-induced MITF and tyrosinase downregulation (Fig. (D)). Therefore, our results suggest that inhibiting melanin synthesis using L-TMP is due to ERK phosphorylation.
Fig. 6. Effects of ERK inhibitor (PD98059) on the L-TMP-induced downregulation of melanogenesis.
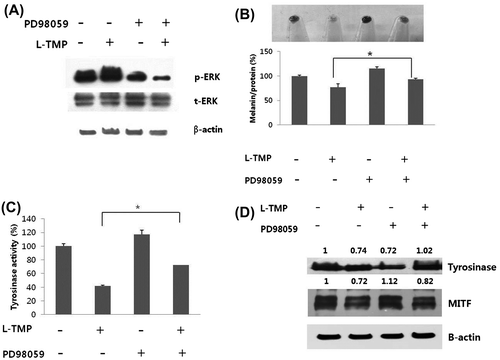
Discussion
In our study, we evaluated the hypopigmentary effects and impact on melanogenesis-related signaling pathways of L-TMP treatment. Our findings show that L-TMP inhibits melanin synthesis by decreasing tyrosinase activity and downregulating MITF and tyrosinase protein expression. These mechanisms involve the activation of the ERK pathway via L-TMP.
Sphingolipids and their metabolites—in particular, ceramide, sphingosine, sphingosine-1-phosphate (S1P), and phytosphingosine—have been identified as important lipid messengers that regulate cellular processes such as cell proliferation, differentiation, senescence, and survival.Citation17) Ceramide and its metabolite (sphingosine) demonstrate antiproliferative functions, inducing cell cycle arrest and apoptosis.Citation18) In contrast, S1P, which is produced by the phosphorylation of sphingosine by sphingosine kinase-1 (SK-1), induces cell growth and inhibits ceramide-mediated apoptosis.Citation19) SK-1 increases the antiapoptotic molecule S1P, reduces ceramide, and is considered an important regulator of ceramide/S1P balance. Sphingosine acts as a negative modulator of protein kinase C (PKC), including SK-1.Citation20) Interestingly, N,N,N-trimethylsphigosine has demonstrated much stronger inhibitory effects on PKC activity than N,N-dimethylsphingosine and unsubstituted sphingosine.Citation21)
Phytosphingosine is structurally similar to sphingosine and ceramide, but differs because phytosphingosine has a hydroxyl group at C-4 of the sphingoid long-chain base, whereas sphingosine and ceramide both contain a trans double bond between C-4 and C-5.Citation9) Synthetic phytosphingosine derivatives, such as N-monomethylphytosphingosine and N,N-dimethylphytosphingosine, demonstrate cytotoxic effects by inhibiting sphingosine kinase-1.Citation22) In addition to its anticancer effects, phytosphingosine stimulates the differentiation of human keratinocytes and inhibits inflammatory epidermal hyperplasia in the skin of hairless mice.Citation23) Phytosphingosine also activates the transcriptional activity of peroxisome proliferator-activated receptors (PPARs), which play important roles in skin barrier homeostasis and epidermal differentiation.Citation23)
Sphingolipids and its metabolites regulate the growth and melanogenesis of human melanocytes. Ceramide, S1P, and sphingosylphosphorylcholine decrease melanogenesis and reduce melanin synthesis by downregulating tyrosinase expression.Citation24) In addition to the effects on melanogenesis, sphingolipid metabolites also contribute to cell survival. S1P has been shown to protect melanocytes from UVB-induced apoptosis.Citation25) These findings suggest that sphingolipid metabolites are involved in the regulation of melanocyte survival and melanogenesis.
The effects of TMP on the growth and melanogenesis of human melanocytes remain unknown. Although TMP is a sphingosine structural analog, the effects of TMP on melanogenesis have not been elucidated. Here, we determined the effects of TMP on melanin synthesis and tyrosinase activity using NHMs. We found that TMP inhibits melanin synthesis and TMP-induced hypomelanogenesis is associated with decreased MITF and tyrosinase protein levels, which are critical to melanogenesis processes. Therefore, reduced MITF and tyrosinase levels and activity may be responsible for reduced melanin synthesis in TMP-treated cells. It has already been demonstrated that when liposomes are used to deliver drugs, the side effects of these agents are reduced and their activities are enhanced.Citation26)
Ceramide, S1P, and other sphingolipid metabolites decrease MITF expression through ERK activation and thereby reduce melanogenesis.Citation6,25,27) It has also been suggested that ERK plays a significant role in melanogenic processes. While ERK-dependent phosphorylation of MITF increases the transcriptional activity of the MITF protein, it has also been reported that sustained ERK activation induces MITF phosphorylation at serine 73 following proteasomal degradation, which thereby reduces tyrosinase protein and melanogenesis.Citation6,7) Therefore, we examined the phosphorylation status of ERK in L-TMP-treated cells. ERK activation was induced by L-TMP treatment, and PD98059, an ERK inhibitor, abrogated L-TMP-induced melanin reduction and prevented MITF and tyrosinase downregulation by L-TMP. These results indicate that L-TMP inhibits MITF and tyrosinase protein levels through ERK activation, which results in the inhibition of melanin synthesis. L-TMP also decreased the levels of MITF and tyrosinase protein. Western blotting analysis showed that the level of MITF protein, but not the level of tyrosinase protein, decreased at 72 h after L-TMP treatment. The level of tyrosinase protein was decreased at 96 h after L-TMP treatment. This sequential phenomenon supports the belief that the activity of tyrosinase is reduced through the degradation of MITF.
Previous studies have shown that activation of p38 MAPK suppresses MITF and tyrosinase activity and thus inhibits melanin synthesis.Citation28,29) We showed that L-TMP treatment induces the phosphorylation of not only ERK, but also p38 MAPK. In our study, the abrogating effect of PD98059 on L-TMP-induced suppression of MITF and tyrosinase activity was weak. This suggests that, although the inhibitory effects of L-TMP on melanogenesis are mainly attributed to the phosphorylation of ERK, other signaling pathways such as the p38 MAPK pathway also partially participate in L-TMP-induced antimelanogenesis.
Sphingosine and its metabolites inhibit PKC, which is required for tyrosinase activation by phosphorylating the serine residues of tyrosinase.Citation16,19,21,22,30) PKC is translocated from the cytoplasm to the membrane, where it is activated by diacylglycerol to further phosphorylate serine/threonine residues on the target proteins. PKC stimulates proliferation and melanogenesis in melanocytes. The PKC-β isoform regulates human melanogenesis by activating tyrosinase without affecting tyrosinase expression.Citation15,16) This study shows that PKC activity and the PKC protein level are not altered by L-TMP treatment, and these indicate that the inhibitory effects of L-TMP on melanogenesis do not depend on PKC activity. PKC activity is reduced by high-concentration L-TMP treatment (>40 μg/mL), but L-TMP did not affect the activity of PKC at the doses used in our experiment (<10 μg/mL).
In summary, based on our current findings, we suggest that L-TMP demonstrates novel antimelanogenic effects. After treating human melanocytes with L-TMP, L-TMP was found to significantly inhibit melanin synthesis in a concentration-dependent manner. The findings indicate that TMP is a potent inhibitor of melanogenesis, and liposomes that contain TMP also retain these properties while demonstrating reduced cytotoxicity. We conclude that L-TMP demonstrates novel hypopigmentary effects by activating the ERK pathway, which leads to the downregulation of MITF and tyrosinase expression.
Author contributions
Woo Jin Lee, Seunghyun Bang, Sung Eun Chang contributed to the study concept and design; Woo Jin Lee, Seunghyun Bang, Hyejung Jung, Sung Eun Chang formed the analysis and interpretation of the data; Woo Jin Lee drafted the manuscript; Bo Young Chung, Eok Soo Oh, Sung Eun Chang carried out the critical revision of the manuscript for important intellectual content; Seunghyun Bang performed the statistical analysis; Woo Jin Lee, Seunghyun Bang, Eok Soo Oh, Sung Eun Chang provided administrative, technical, or material support; Sung Eun Chang supervised the work.
Disclosure statement
No potential conflict of interest was reported by the authors.
Acknowledgments
We thank Dr. Myeong Jun Choi (Kyunggi University, Suwon, Korea) for the gift of L-TMP.
Notes
Abbreviations: ANOVA, Analysis of variance; cAMP/PKA, cyclic adenosine monophosphate/protein kinase A; DOPA, dihydroxyphenylalanine; ERK, extracellular signal-regulated kinase; L-TMP, liposomal TMP; MITF, microphthalmia-associated transcription factor; MSH, melanocyte-stimulating hormone; NHM, normal human melanocyte; p38 MAPK, p38 mitogen-activated protein kinase; PBS, phosphate-buffered saline; PI3K/Akt, phosphoinositide 3-kinase/Akt; PKC, protein kinase C; SDS–PAGE, sodium dodecyl sulfate polyacrylamide gel electrophoresis; TMP, trimethyl phytosphingosine-iodide; TRP-1, tyrosinase-related protein; TRP-2, tyrosinase-related protein 2; WST, water-soluble tetrazolium salt.
References
- Hearing VJ, Tsukamoto K. Enzymatic control of pigmentation in mammals. FASEB J. 1991;5:2902–2909.
- Bellei B, Maresca V, Flori E, Pitisci A, Larue L, Picardo M. p38 regulates pigmentation via proteasomal degradation of tyrosinase. J. Biol. Chem. 2010;285:7288–7299.10.1074/jbc.M109.070573
- D'Orazio J, Fisher DE. Central role for cAMP signaling in pigmentation and UV resistance. Cell Cycle. 2011;10:8–9.10.4161/cc.10.1.14292
- Schiaffino MV. Signaling pathways in melanosome biogenesis and pathology. Int. J. Biochem. Cell Biol. 2010;42:1094–1104.10.1016/j.biocel.2010.03.023
- Oka M, Nagai H, Ando H, et al. Regulation of melanogenesis through phosphatidylinositol 3-kinase-Akt pathway in human G361 melanoma cells. J. Invest. Dermatol. 2000;115:699–703.10.1046/j.1523-1747.2000.00095.x
- Kim DS, Hwang ES, Lee JE, Kim SY, Kwon SB, Park KC. Sphingosine-1-phosphate decreases melanin synthesis via sustained ERK activation and subsequent MITF degradation. J. Cell Sci. 2003;116:1699–1706.10.1242/jcs.00366
- Levy C, Khaled M, Fisher DE. MITF: master regulator of melanocyte development and melanoma oncogene. Trends Mol. Med. 2006;12:406–414.10.1016/j.molmed.2006.07.008
- Jo SY, Kim HC, Woo SW, Seo MJ, Lee G, Kim HR. Synthesis of 1-substituted-phytosphingosine, novel protection of phytosphingosine. Bull. Korean Chem. Soc. 2003;24:267–268.
- Park MT, Kang JA, Choi JA, et al. Phytosphingosine induces apoptotic cell death via caspase 8 activation and Bax translocation in human cancer cells. Clin. Cancer Res. 2003;9:878–885.
- Park MT, Choi JA, Kim MJ, et al. Suppression of extracellular signal-related kinase and activation of p38 MAPK are two critical events leading to caspase-8- and mitochondria-mediated cell death in phytosphingosine-treated human cancer cells. J. Biol. Chem. 2003;278:50624–50634.10.1074/jbc.M309011200
- Song CK, Lee JH, Jahn A, et al. In vitro and in vivo evaluation of N, N, N-trimethylphytosphingosine-iodide (TMP) in liposomes for the treatment of angiogenesis and metastasis. Int. J. Pharm. 2012;434:191–198.10.1016/j.ijpharm.2012.05.042
- Curto EV, Kwong C, Hermersdörfer H, et al. Inhibitors of mammalian melanocyte tyrosinase: in vitro comparisons of alkyl esters of gentisic acid with other putative inhibitors. Biochem. Pharmacol. 1999;57:663–672.10.1016/S0006-2952(98)00340-2
- Busca R, Bertolotto C, Ortonne JP, Ballotti R. Inhibition of the phosphatidylinositol 3-kinase/p70(S6)-kinase pathway induces B16 melanoma cell differentiation. J. Biol. Chem. 1996;271:31824–31830.10.1074/jbc.271.50.31824
- Winder AJ. A stopped spectrophotometric assay for the dopa oxidase activity of tyrosinase. J. Biochem. Biophys. Methods. 1994;28:173–183.10.1016/0165-022X(94)90014-0
- Park HY, Russakovsky V, Ohno S, Gilchrest BA. The beta isoform of protein kinase C stimulates human melanogenesis by activating tyrosinase in pigment cells. J. Biol. Chem. 1993;268:11742–11749.
- Park HY, Perez JM, Laursen R, Hara M, Gilchrest BA. Protein kinase C-beta activates tyrosinase by phosphorylating serine residues in its cytoplasmic domain. J. Biol. Chem. 1999;274:16470–16478.10.1074/jbc.274.23.16470
- Spiegel S, Merrill AH Jr. Sphingolipid metabolism and cell growth regulation. FASEB J. 1996;10:1388–1397.
- Ogretmen B, Hannun YA. Biologically active sphingolipids in cancer pathogenesis and treatment. Nat. Rev. Cancer. 2004;4:604–616.10.1038/nrc1411
- Cuvillier O, Pirianov G, Kleuser B, et al. Suppression of ceramide-mediated programmed cell death by sphingosine-1-phosphate. Nature. 1996;381:800–803.10.1038/381800a0
- Hannun YA, Bell RM. Functions of sphingolipids and sphingolipid breakdown products in cellular regulation. Science. 1989;243:500–507.10.1126/science.2643164
- Endo K, Igarashi Y, Nisar M, Zhou Q, Hakomori S. Cell membrane signaling as target in cancer therapy: inhibitory effect of N, N-dimethyl and N, N, N-trimethyl sphingosine derivatives on in vitro and in vivo growth of human tumor cells in nude mice. Cancer Res. 1991;51:1613–1618.
- Park SR, Cho HJ, Moon KJ, et al. Cytotoxic effects of novel phytosphingosine derivatives, including N, N-dimethylphytosphingosine and N-monomethylphytosphingosine, in human leukemia cell line HL60. Leuk. Lymphoma. 2010;51:132–145.10.3109/10428190903383419
- Kim S, Hong I, Hwang JS, et al. Phytosphigosine stimulates the differentiation of human keratinocytes and inhibits TPA-induced inflammatory epidermal hyperplasia in hairless mouse skin. Mol. Med. 2006;12:17–24.
- Kim DS, Park SH, Kwon SB, et al. Sphingosylphosphorylcholine-induced ERK activation inhibits melanin synthesis in human melanocytes. Pigment Cell Res. 2006;19:146–153.10.1111/pcr.2006.19.issue-2
- Kim DS, Hwang ES, Lee JE, Kim SY, Park KC. Sphingosine-1-phosphate promotes mouse melanocyte survival via ERK and Akt activation. Cell Signal. 2003;15:919–926.10.1016/S0898-6568(03)00055-X
- Gabizon A, Dagan A, Goren D, Barenholz Y, Fuks Z. Liposomes as in vivo carriers of adriamycin:reduced cardiac uptake and preserved antitumor activity in mice. Cancer Res. 1982;42:4734–4739.
- Kim DS, Kim SY, Chung JH, Kim KH, Eun HC, Park KC. Delayed ERK activation by ceramide reduces melanin synthesis in human melanocytes. Cell Signal. 2002;14:779–785.10.1016/S0898-6568(02)00024-4
- Kim SY, Lee SH, Shin JS, et al. A derivative of imidazobenzimidazole, ML106, inhibits melanin synthesis via p38 MAPK activation. Pharmazie. 2014;69:353–357.
- Baek SH, Lee SH, Sesamol decreases melanin biosynthesis in melanocyte cells and zebrafish: Possible involvement of MITF via the intracellular cAMP and p38/JNK signaling pathways, Exp. Dermatol. 2015; E-pub ahead of print. doi: 10.1111/exd.12765.
- Wu H, Park HY. Protein kinase C-beta-mediated complex formation between tyrosinase and TRP-1. Biochem. Biophys. Res. Commun. 2003;311:948–953.10.1016/j.bbrc.2003.10.092