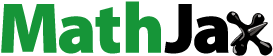
Abstract
In the cytosol of Saccharomyces cerevisiae, most of the free N-glycans (FNGs) are generated from misfolded glycoproteins by the action of the cytoplasmic peptide: N-glycanase (Png1). A cytosol/vacuole α-mannosidase, Ams1, then trims the FNGs to eventually form a trisaccharide composed of Manβ1,4GlcNAc β1,4GlcNAc (Man1GlcNAc2). Whether or not the resulting Man1GlcNAc2 is enzymatically degraded further, however, is currently unknown. The objective of this study was to unveil the fate of Man1GlcNAc2 in S. cerevisiae. Quantitative analyses of the FNGs revealed a steady increase in the amount of Man1GlcNAc2 produced in the post-diauxic and stationary phases, suggesting that this trisaccharide is not catabolized during this period. Inoculation of the stationary phase cells into fresh medium resulted in a reduction in the levels of Man1GlcNAc2. However, this reduction was caused by its dilution due to cell division in the fresh medium. Our results thus indicate that Man1GlcNAc2 is not enzymatically catabolized in S. cerevisiae.
Graphical abstract
Man1GlcNAc2 is the final enzymatic product of free N-glycan catabolism in S. cerevisiae
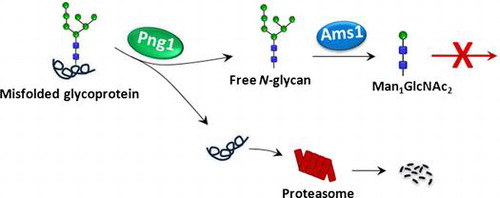
N-Glycosylation, or asparagine-linked glycosylation, is widely recognized as one of the most important translational modifications of eukaryotic proteins. Numerous reports have shown that this modification plays pivotal roles in modulating the physicochemical/physiological properties of carrier proteins.Citation1) The biosynthetic pathway for the precursor of N-glycans has been well clarified in both mammalian cells and yeast, and most, if not all, of the genes encoding the enzymes/transporters involved in the process have been identified.Citation2,3) On the other hand, there are many issues remaining to be unveiled regarding aspects of the catabolism of such N-glycoproteins even in S. cerevisiae, one of the most well studied eukaryotic model organisms. For example, as of this writing, only two enzymes, Png1 and Ams1, have been shown to be involved in the catabolism of the glycan-parts of N-glycoproteins (Fig. ). Png1 is a deglycosylating enzyme that releases N-glycans (referred to as free N-glycans or FNGs) from the misfolded N-glycoproteins,Citation5) while Ams1 catalyzes the removal of α-mannoses from the FNGs.Citation6,7) The concerted action of these enzymes results in the formation of a trisaccharide Man1GlcNAc2 (Manβ1,4GlcNAc β1,4GlcNAc).Citation3,7,8) This trisaccharide is believed to be the end product of FNG catabolism, since no β-mannosidase or β-hexosaminidase to break down Man1GlcNAc2 is known to be produced by this yeast. Chantret et al.Citation8)reported, however, that Man1GlcNAc2 levels decline when stationary cells are re-incubated in fresh medium to resume log (growing) phase, raising the possibility that there might be a log phase-specific catabolic pathway for Man1GlcNAc2.
Fig. 1. Processing/degradation pathways for N-glycoproteins in the ER and cytosol of S. cerevisiae.Citation3,4)
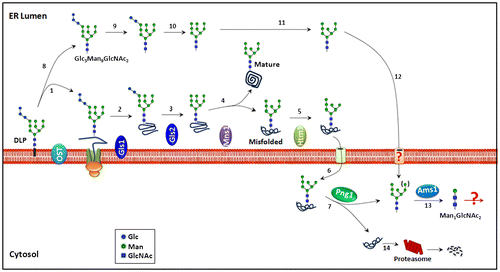
In this study, we attempted to determine the fate of Man1GlcNAc2 to clarify if it is really the final FNG or whether it is metabolized further by enzymatic reactions. We analyzed FNGs in the log phase, post-diauxic phase and stationary phase in yeast cells and consistent with the previous reports, Man1GlcNAc2 levels were found to be increasing after the log phase.Citation8) On the other hand, when the stationary phase day-7 culture was transferred to new media to start the log phase (stationary day-7 to log phase or STL), a reduction in Man1GlcNAc2 was observed. This reduction, however, can be attributed solely to a dilution effect by the cell division, rather than by enzymatic catabolism of this trisaccharide. Our results thus imply that S. cerevisiae does not produce a catabolic enzyme that acts on Man1GlcNAc2 and that this trisaccharide appears to be the end product of the enzymatic catabolic pathway for FNGs in this organism.
Materials and methods
Strains and growth conditions
BY4741 (genotype: MATa his3Δ1 leu2Δ0 met15Δ0 ura3Δ0, American Type Culture Collection) was used in this study. The cells were grown and maintained in YPD (Yeast Peptone Dextrose) medium (1% yeast extract, 2% peptone, 2% glucose) and all cultures were performed in a rotary shaker at 180 rpm at 30 °C. Cell growth was monitored by measuring the optical density (OD) at 600 nm. Yeast cells were streaked from a 15% (v/v) glycerol stock onto YPD plates, and after two days of incubation, cells from the plate were inoculated in 15 mL of YPD medium and cultured overnight. This overnight preculture was then inoculated in 200 mL of fresh YPD medium at a cell concentration of OD600 = 0.5. After 4 h and 1, 3, and 7 days of culture, cells equivalent to 30 OD600 units were harvested from the culture as the log phase (4 h), the post-diauxic phase (day-1 and day-3), and the stationary phase (day-7) samples. For STL, the day-7 culture was inoculated in 400 mL of fresh YPD medium at a cell concentration of OD600 = 0.5. After 0, 1.5, 3, and 4.5 h culture, the cells equivalent to 30 OD600 units were harvested for extraction of FNGs.
Preparation and pyridylamination of FNGs from Saccharomyces cerevisiae
The preparation and pyridylamination of the FNGs were carried out as described previously Citation7,9) with minor modifications. In brief, yeast cells were washed twice with distilled water, resuspended in 1 mL of lysis buffer (20 mM Tris–HCl, pH 7.4, and 10 mM EDTA), and ethanol was then added at a final concentration of 70%. The cells were homogenized by three 10-s vigorous agitations separated by 5-min cooling periods on ice. The homogenate was centrifuged at 15,000×g for 15 min, and the supernatant was recovered as the FNG fraction. The fraction was then evaporated to dryness, and was dissolved in 250 μL water and applied onto a column containing 600 μL each of AG1-X2 (50% slurry; 200–400 mesh; acetate form; Bio-Rad) and AG50-X8 (50% slurry; 200–400 mesh; H+ form; Bio-Rad) resins for desalting. The columns were further washed with 3 mL of water. The flow-through fraction was collected, evaporated to dryness, and fluorescently labeled by incubating the samples in 20 μL of 2.76 g/mL 2-aminopyridine (2-AP) in acetic acid for 1 h at 80 °C. After the reaction, 20 μL of 500 mg/mL dimethylamine borane in acetate was added to the mixture and further incubated for 1 h at 80 °C. The excess amounts of free 2-AP were removed by a MonoFas silica gel spin column (GL Sciences) as described previously.Citation7)
Preparation of the pyridylaminated Man1GlcNAc2
The pyridylaminated Man1GlcNAc2 (Man1GlcNAc2-PA) was prepared from N-glycans released from bovine ribonuclease B (Sigma) by PNGase F (Roche Applied Science), which were further digested with jack bean (JB) α-mannosidase (Seikagaku Corp.) as described previously.Citation7)
Size-fractionation high-performance liquid chromatography
Pyridylaminated-glycans (PA-glycans) were separated by size-fractionation high-performance liquid chromatography (HPLC) with a Shodex NH2P-50 4E column (4.6 × 250 mm; Shodex), as reported previously with minor modifications.Citation7,9) The elution was achieved by two solvent gradients: solvent A [93% acetonitrile in 0.3% acetate (pH adjusted to 7.0 with ammonia)] and solvent B [20% acetonitrile in 0.3% acetate (pH adjusted to 7.0 with ammonia)]. The flow rate was 0.8 mL/min and the column temperature was 25 °C. The gradient program was as follows: 0–0.5 min, 1–10% solvent B; 0.5–45 min, 10–55% solvent A; 45.1–47 min, isocratic 70% solvent B; 47.1–67.1 min, isocratic 1% solvent B. The fluorescence of the PA-glycans was detected at the excitation wavelength (310 nm) and the emission wavelength (380 nm). Total FNGs (α-mannosidase-sensitive peaks) as well as Man1GlcNAc2 glycans were quantitated from the HPLC profile based on the peak area of standard PA-glucose hexamer (PA-Glc6; 2 pmol/μL) in the standard PA-glucose oligomer (degree of polymerization = 3–15; Takara), except for Man2GlcNAc2-PA which co-eluted with a contaminating peak on size-fractionation HPLC. The amount of Man2GlcNAc2 therefore was quantitated by applying the equivalent fraction on size-fractionation HPLC to reversed-phase HPLC as described below. The Man2GlcNAc2 peaks, which corresponded to the authentic samples obtained from Masuda Kagaku Co. (Takamatsu, Japan) on the reversed-phase HPLC, was quantitated, using PA-Glc6 as a quantitation standard.
Reversed-phase HPLC
Reversed-phase HPLC was performed as described previously with minor modificationsCitation7) with a TSK-gel ODS-80TM column (4.6 × 150 mm; TOSOH, Tokyo, Japan). Elution was achieved by two solvent gradients: solvent A (0.1 M ammonium acetate buffer, pH 4.0) and solvent B (0.1 M ammonium acetate buffer, pH 4.0, and 0.5% 1-butanol). The flow rate was 1 mL/min. The column temperature was set to 22 °C. The gradient program was as follows: 0–40 min, 5–55.8% solvent B; 40.1–45 min, 75% isocratic solvent B; 45.1–65 min, isocratic 5% solvent B. Fluorescence of the labeled glycans was detected at the excitation wavelength (320 nm) and the emission wavelength (400 nm).
Glycosidase digestion
Digestion of the PA-FNGs with mannosidases was performed as described previously.Citation7) Briefly, PA-FNGs were digested overnight with JB α-mannosidase (40 mU; Seikagaku Corp., Tokyo, Japan) in 20 μL of 10 mM sodium citrate buffer (pH 4.5) at 37 °C. For β-mannosidase digestion, 12 mU of the enzyme (Sigma) was incubated with PA-FNGs in 20 μL of 10 mM sodium citrate buffer (pH 4.5) at 37 °C overnight.
Estimation of cell concentration and determination of the calculated value of Man1GlcNAc2
To accurately calculate the number of cells, the day-7 stationary phase culture was re-inoculated in a 400-mL of fresh YPD medium at a cell concentration of OD600 = 0.5. The OD600 values were then measured after culturing for 0, 1.5, 3, 4.5, and 5 h, and 3000-fold dilutions of each culture were spread on YPD plates. After incubation for 2 days at 30 °C, the numbers of colonies on the plates were counted. The averages from the full data set were approximated to a linear equation. After three individual experiments, a linear equation (y = 172x − 52) was obtained and was used to calculate the number of cells at each time point from the OD600. In our hands, this equation can be effectively applied from OD600 ranging from 0.5 to 1.7. The calculated amount of Man1GlcNAc2 [N]x at a particular time point (x hours after inoculation) was obtained from the following equation.
where [N]0 is the amount of Man1GlcNAc2 quantitated at 0 h of STL.
Results
Man1GlcNAc2 accumulates in the post-diauxic and stationary phases
The objective of this study was to investigate whether Man1GlcNAc2 is further metabolized or whether it is an end product in S. cerevisiae. To this end, time-dependent changes of the FNGs in the log-phase (4 h), post-diauxic phase (day-1 and day-3), and stationary-phase (day-7) were analyzed. FNGs were extracted from the cells, fluorescently labeled and analyzed by size-fractionation HPLC. In the log-phase, 4 major peaks (peaks b, c, d, and e; Fig. (A) were found to be susceptible to JB α-mannosidase (data not shown). It has previously been shown that these peaks correspond to the Man6–9GlcNAc2 FNGs.Citation7,8) On the other hand, no peak corresponding to Man1GlcNAc2 (peak f) was observed in the log phase cells, suggesting that Man1GlcNAc2 is normally not produced in the log phase by this organism. In the post-diauxic phase (day-1 and day-3), in addition to the Man6–9GlcNAc2, smaller α-mannosidase-sensitive FNGs corresponding to Man3–5GlcNAc2 (peaks g, h, and i) were detected (Fig. (A) day-1 and day-3). Those FNGs (Man3–9GlcNAc2) were barely detected in the stationary phase (Fig. (A); day-7).
Fig. 2. Identification and characterization of Man1GlcNAc2-PA.
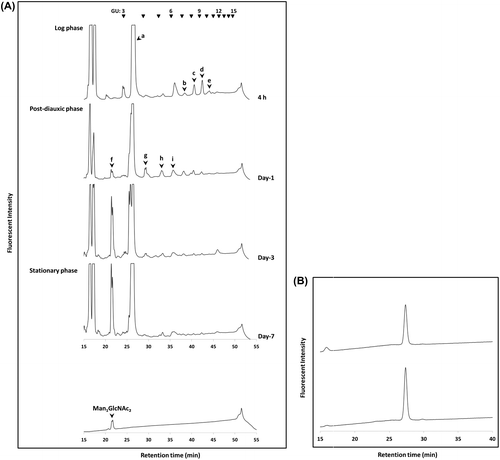
To identify the Man1GlcNAc2-PA on the HPLC chart, authentic Man1GlcNAc2-PA was prepared by the α-mannosidase digestion of FNGs (Man5–9GlcNAc2) released from RNase B by PNGase F. It was found that the authentic Man1GlcNAc2-PA co-migrated with the position of peak f (Fig. (A), bottom panel), indicating that this peak represents Man1GlcNAc2-PA. The isolated peak f also co-migrated with the authentic Man1GlcNAc2-PA in a reversed-phase HPLC analysis (Fig. (B)). The structure was further confirmed by the finding that it was digested by β-mannosidase (data not shown). This result is consistent with the previous findings showing that Man1GlcNAc2 is the predominant FNG in the stationary phase yeast cells.Citation8) While the reason for forming a doublet when Man1GlcNAc2-PA is analyzed by size-fractionation HPLC has not been clarified, it can be safely assumed that this is due to the deterioration of the column since (1) the doublet-formation was not obvious for a new column (cf. see Fig. (A) and (2) even standard PA-Glc oligomers (from trimer to hexamer) were eluted as doublet peaks when the old column was used (data not shown).
When the total amounts of FNGs were quantitated, it was found that there was a steady increase in the total amount of FNGs up to stationary phase day-7, and there was also a rapid increase in the amount of Man1GlcNAc2, which represents ~94% of the total FNGs at stationary phase day-7 (Fig. ). Taking these results into account, it can be safely concluded that Man1GlcNAc2 is not enzymatically catabolized during the post-diauxic/stationary phases.
Man1GlcNAc2 are diluted by cell divisions when the stationary phase cells are inoculated to fresh media
While the active catabolism of Man1GlcNAc2 is not observed during post-diauxic/stationary phases, it was previously reported that the amount of Man1GlcNAc2 that had accumulated in the stationary phase, decreased when the cells were inoculated into fresh medium,Citation8) raising the possibility that Man1GlcNAc2 is catabolized in the log phase. To better understand the catabolic pathway of Man1GlcNAc2 in log phase cells, stationary phase yeast cells, which contain relatively large amounts of Man1GlcNAc2, were transferred to fresh medium and the Man1GlcNAc2 levels were closely monitored at various time points. The results showed that, as the cells started to divide, the levels of Man1GlcNAc2 gradually decreased (Fig. (A–C)). However, this reduction of Man1GlcNAc2 is in good agreement with the “calculated value” of Man1GlcNAc2 which was calculated based on the assumption that the reduction is due to the effect of dilution upon cell division and not by active enzymatic catabolism (Fig. (C)). These results strongly suggest that no enzymatic catabolism of Man1GlcNAc2 occurs, even in the log phase.
Fig. 4. Reduction of Man1GlcNAc2 in STL is only due to cell division.
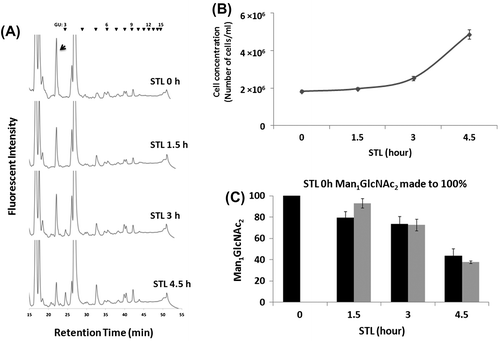
Discussion
In this study, we attempted to clarify the catabolic pathway of FNGs that are generated mainly from the misfolded glycoproteins in S. cerevisiae. Our results fail to provide any evidence to suggest that Man1GlcNAc2 is enzymatically catabolized, strongly indicating that this is the enzymatic end product for S. cerevisiae. First, we observed a steady increase in the amount of Man1GlcNAc2 in yeast cells during an extended period of the culture. Consistent with this observation, there was no evidence to suggest the release of FNGs, including Man1GlcNAc2, into the media (Hossain and Suzuki, unpublished observation). Moreover, when stationary phase cells, in which Man1GlcNAc2 is accumulated, were inoculated to fresh media to resume logarithmic growth, we failed to find any evidence to show that Man1GlcNAc2 is catabolized, even in the log phase. This is in sharp contrast to the case of mammalian cells, which produce lysosomal enzymes (β-mannosidases, chitobiase, or β-hexosaminidase) that are fully capable of degrading FNGs into monomeric sugars.Citation10,11)
Under conditions where carbon sources are limited, cells may attempt to obtain those by digestions of their own molecules. The induction of Ams1 at stationary phase makes sense in this regard, as it can generate free Man from the FNGs in the cytosol/vacuoles, which could then re-enter the biosynthetic pathway of N-glycans or other glycoconjugates. The issue of why yeast does not have an enzymatic system to utilize Man1GlcNAc2 is curious. It should also be noted that S. cerevisiae is also unique for not producing an endo-β-N-acetylglucosaminidase (ENGase). While this enzyme is extensively distributed among eukaryotes including various yeast/fungi, some yeasts such as S. cerevisiae or S. pombe do not possess the gene ortholog of the known ENGases.Citation12–14) Moreover, the enzyme activity could not be detected using S. cerevisiae extract.Citation15) On the other hand, occurrence of β-mannosidase has been reported in a wide variety of yeast/fungi.Citation16–19) Those enzymes, so far identified, belong to a glycoside hydrolase family 2 on CAZy database,Citation20) as is the case with a human lysosomal β-mannosidase. Again, orthologous gene(s) for the β-mannosidase cannot be found in S. cerevisiae or S. pombe. It remains to be seen if a lack of enzymatic degradation of Man1GlcNAc2 is specific for S. cerevisiae or is also associated with other more diverse organisms.
The question remains as to whether there is any alternate route for this yeast to utilize Man1GlcNAc2. While we failed to provide any evidence for the enzymatic catabolism of this glycan, it may be possible that, at least under some specific conditions, this glycan could be degraded non-enzymatically. For example, the hydroxyl free radical-mediated cleavage of N-glycans has been reported.Citation21) Whether similar radical-mediated cleavage of FNGs, including Man1GlcNAc2, occurs in yeast remains to be determined. If it is indeed the case, yeast might degrade FNGs via such a non-enzymatic system.
In summary, we determined the fate of Man1GlcNAc2 in yeast cells. We analyzed FNGs at different growth stages and found that Man1GlcNAc2 is detectable at day-1 and then continues to accumulate during the post-diauxic and stationary phases of growth. When stationary cells with high Man1GlcNAc2 contents were inoculated into fresh media to resume the logarithmic growth phase, Man1GlcNAc2 was found to be metabolically unchanged. Our conclusion is that S. cerevisiae is unable to catabolize Man1GlcNAc2 in an enzyme-dependent fashion.
Author contributions
TJH and TS designed the experiments; TJH performed the experiments; TJH, HH, YH, and TS analyzed the data; TJH and TS wrote the manuscript.
Disclosure statement
No potential conflict of interest was reported by the authors.
Funding
This work was partially supported by the RIKEN International Joint Graduate School Program, and Grants-in-Aid from the Ministry of Education, Culture, Sports, Science, and Technology of Japan [grant number 25291030 to TS].
Acknowledgments
We thank the members of Glycometabolome team for fruitful discussions.
Notes
Abbreviations: FNG, free N-glycan; STL, stationary phase day-7 to log phase; 2-AP, 2-aminopyridine; HPLC, high-performance liquid chromatography; GU, glucose unit.
References
- Varki A. Biological roles of oligosaccharides: all of the theories are correct. Glycobiology. 1993;3:97–130. 10.1093/glycob/3.2.97
- Helenius A, Aebi M. Roles of N-linked glycans in the endoplasmic reticulum. Annu. Rev. Biochem. 2004;73:1019–1049. 10.1146/annurev.biochem.73.011303.073752
- Harada Y, Hirayama H, Suzuki T. Generation and degradation of free asparagine-linked glycans. Cell. Mol. Life Sci. 2015;72:2509–2533. 10.1007/s00018-015-1881-7
- Suzuki T, Harada Y. Non-lysosomal degradation pathway for N-linked glycans and dolichol-linked oligosaccharides. Biochem. Biophys. Res. Commun. 2014;453:213–219. 10.1016/j.bbrc.2014.05.075
- Suzuki T, Park H, Hollingsworth NM, Sternglanz R, Lennarz WJ. PNG1, a yeast gene encoding a highly conserved peptide: N-glycanase. J. Cell Biol. 2000;149:1039–1052. 10.1083/jcb.149.5.1039
- Chantret I, Frénoy JP, Moore SE. Free-oligosaccharide control in the yeast Saccharomyces cerevisiae: roles for peptide: N-glycanase (Png1p) and vacuolar mannosidase (Ams1p). Biochem. J. 2003;373:901–908. 10.1042/BJ20030384
- Hirayama H, Seino J, Kitajima T, Jigami Y, Suzuki T. Free oligosaccharides to monitor glycoprotein endoplasmic reticulum-associated degradation in Saccharomyces cerevisiae. J. Biol. Chem. 2010;285:12390–12404. 10.1074/jbc.M109.082081
- Chantret I, Kodali VP, Lahmouich C, Harvey DJ, Moore SE. Endoplasmic reticulum-associated degradation (ERAD) and free oligosaccharide generation in Saccharomyces cerevisiae. J. Biol. Chem. 2011;286:41786–41800. 10.1074/jbc.M111.251371
- Harada Y, Buser R, Ngwa EM, Hirayama H, Aebi M, Suzuki T. Eukaryotic oligosaccharyltransferase generates free oligosaccharides during N-glycosylation. J. Biol. Chem. 2013;288:32673–32684. 10.1074/jbc.M113.486985
- Suzuki T. Introduction to “Glycometabolome”. Trends Glycosci. Glycotechnol. 2009;21:219–227. 10.4052/tigg.21.219
- Winchester B. Lysosomal metabolism of glycoproteins. Glycobiology. 2005;15(6):1R–15R.
- Suzuki T, Yano K, Sugimoto S, Kitajima K, Lennarz WJ, Inoue S, et al. Endo-β-N-acetylglucosaminidase, an enzyme involved in processing of free oligosaccharides in the cytosol. Proc. Natl. Acad. Sci. 2002;99:9691–9696. 10.1073/pnas.152333599
- Murakami S, Takaoka Y, Ashida H, Yamamoto K, Narimatsu H, Chiba Y. Identification and characterization of endo-β-N-acetylglucosaminidase from methylotrophic yeast Ogataea minuta. Glycobiology. 2013;23:736–744. 10.1093/glycob/cwt012
- Stals I, Samyn B, Sergeant K, White T, Hoorelbeke K, Coorevits A, et al. Identification of a gene coding for a deglycosylating enzyme in Hypocrea jecorina. FEMS Microbiol. Lett. 2010;303:9–17. 10.1111/fml.2010.303.issue-1
- Suzuki T, Park H, Kitajima K, Lennarz WJ. Peptides glycosylated in the endoplasmic reticulum of yeast are subsequently deglycosylated by a soluble peptide: N-glycanase activity. J. Biol. Chem. 1998;273:21526–21530. 10.1074/jbc.273.34.21526
- Ademark P, Lundqvist J, Hägglund P, et al. Hydrolytic properties of a β-mannosidase purified from Aspergillus niger. J. Biotechnol. 1999;75:281–289. 10.1016/S0168-1656(99)00172-8
- Kulminskaya AA, Eneiskaya EV, Isaeva-Ivanova LS, Savel’ev AN, Sidorenko IA, Shabalin KA, et al. Enzymatic activity and β-galactomannan binding property of β-mannosidase from Trichoderm reesei. Enzyme Microb. Technol. 1999;25:372–377. 10.1016/S0141-0229(99)00056-3
- Kanamasa S, Takada G, Kawaguchi T, Sumitani J, Arai M. Overexpression and purification of Aspergillus aculeatus β-mannosidase and analysis of the integrated gene in Aspergillus oryzae. J. Biosci. Bioeng. 2001;92:131–137. 10.1016/S1389-1723(01)80213-9
- Oda Y, Tonomura K. Characterization of β-mannanase and β-mannosidase secreted from the yeast Trichosporon cutaneum JCM 2947. Lett. Appl. Microbiol. 1996;22:173–178. 10.1111/j.1472-765X.1996.tb01136.x
- Cantarel BL, Coutinho PM, Rancurel C, Bernard T, Lombard V, Henrissat B. The carbohydrate-active enzymes database (CAZy): an expert resource for glycogenomics. Nucleic Acids Res. 2009;37:D233–D238. 10.1093/nar/gkn663
- Eguchi H, Ikeda Y, Koyota S, Honke K, Suzuki K, Gutteridge JM, et al. Oxidative damage due to copper ion and hydrogen peroxide induces GlcNAc-specific cleavage of an Asn-linked oligosaccharide. J. Biochem. 2002;131:477–484.