Abstract
Serratia marcescens 2170 produces three different types of chitinases and chitin-binding protein CBP21. We found that transposon insertion into the 5′ untranslated region (5′ UTR) of chiPQ-ctb led to defective chitinase and CBP21 production. ChiX small RNA possessed the complementary sequence of the 5′ UTRs of the chiPQ-ctb and chiR and repressed the expression of chiP and chiR. ChiX was detected in a medium containing glucose, glycerol, GlcNAc, and (GlcNAc)2, but the expression of both chiP and chiR was only observed in a medium containing (GlcNAc)2. ∆chiX mutant produced chitinases, CBP21, and chitobiase without induction. chiP transcripts were more abundant than those of chiR or chiX in a medium containing (GlcNAc)2. These results suggest that the constitutively expressed ChiX binds to the highly abundant chiP 5′ UTR, thereby leading to the induction of chiR mRNA translation and the subsequent expression of chitinases and CBP21.
Graphical abstract
Small RNA, ChiX, coordinates the synchronized expression of chitin-degrading enzymes and the system for incorporating chitin degradation products in Serratia marcescens.
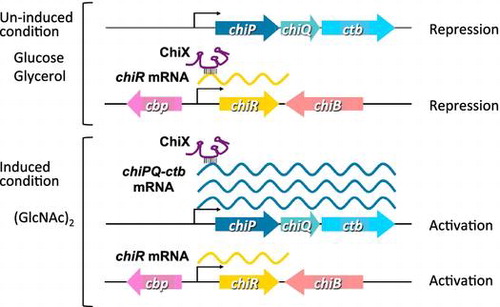
Poly-β-1,4-N-acetylglucosamine (GlcNAc), also known as chitin, is the second most abundant carbohydrate polymer in nature after cellulose. It mainly exists in an insoluble crystalline form in the exoskeleton of crustaceans and arthropods as well as in the cell walls of fungi. Bacteria that produce chitin-degrading enzyme, chitinase, are the primary degraders of chitin. Chitin hydrolysis by chitinases is the most critical step in the degradation and utilization of chitin by bacteria. Most of the chitinolytic bacteria, including Bacillus circulans,Citation1) Aeromonas sp.,Citation2) Alteromonas sp.,Citation3) StreptomycesCitation4,5), and Serratia marcescens,Citation6) have been reported to produce multiple chitinases. Among them, S. marcescens, a Gram-negative bacterium in the family Enterobacteriaceae, is an efficient biological degrader of chitin and one of the most extensively studied chitinolytic bacteria. This bacterium is also well known for producing the red pigment, prodigiosin,Citation7,8) and is involved in nosocomial infections. The chitinolytic system of S. marcescens is one of the best-known insoluble polysaccharide degradation systems. S. marcescens 2170 produces at least four chitin-degrading enzymes, i.e. three chitinases (ChiA, B, and C1) and a chitin-binding protein (CBP21), when grown in the presence of chitin.Citation9) All of the chitinases produced by this bacterium belong to glycoside hydrolase family 18, as defined in carbohydrate-active enzymes (CAZy) database.Citation10,11) Bacterial family 18 chitinases can be further classified into subfamilies A, B, and C on the basis of amino acid sequence similarities of their catalytic domains.Citation12) ChiA and ChiB belong to subfamily A, which have a deep substrate-binding cleft formed by an α + β-fold insertion domain in the catalytic domain, whereas ChiC1 belongs to subfamily B, which lack an α + β-fold insertion domain. A clear synergism in the hydrolysis of powdered chitin was observed with combinations of these chitinases.Citation13) ChiA and ChiB are predicted to work in a specific order and to facilitate chitin hydrolysis in different directions,Citation14) where the catalytic ordered movements of ChiA and ChiB in opposite directions on crystalline β-chitin have been visualized using high-speed atomic force microscopy.Citation15) CBP21 was recently found to be a lytic polysaccharide monooxygenase that introduces chain breaks on the surface of crystalline chitin by oxidative cleavage.Citation16) CBP21 was classified as a carbohydrate-binding module in family 33, but it has now been reclassified in the auxiliary activities family 10 (AA10) according to the CAZy database.Citation17) CBP21 was reported to boost the activity of chitinase.Citation16,18) In order to identify genes that affect the chitin degradation and utilization system, we performed transposon mutations in S. marcescens 2170.Citation6) After screening for chitinase-deficient transposon mutants, we found that chiR, which encodes a LysR-type transcriptional activator, is essential for the production of all chitinases (ChiA, ChiB, and ChiC1) and CBP21 in S. marcescens 2170.Citation19) In addition, N,N′-diacetylchitobiose [(GlcNAc)2], which is the major product of chitin hydrolysis by the chitinases in S. marcescens, is the minimal substrate for chitinase and CBP21 induction in this bacterium.Citation6,13) The uptake of (GlcNAc)2 is mediated by the phosphoenolpyruvate-dependent sugar phosphotransferase system (PTS), and a (GlcNAc)2-specific enzyme II permease is present in the PTS system of S. marcescens 2170. Disruption of the (GlcNAc)2-specific enzyme II permease, which is encoded by the chb operon, drastically reduces the induction of chitinases and CBP21.Citation20) S. marcescens 2170 also produces a 98.5-kDa chitobiase, which is mainly located in the periplasm and hydrolyzes the β-1,4-glycosidic bond of (GlcNAc)2.Citation21) A portion of (GlcNAc)2 enters the periplasm and is taken up into the cytoplasm by the (GlcNAc)2-specific enzyme II permease. The remaining (GlcNAc)2 is converted into GlcNAc by chitobiase, and GlcNAc is taken up via GlcNAc-specific PTS permease. The uptake of both (GlcNAc)2 and GlcNAc is important for the efficient utilization of (GlcNAc)2 in S. marcescens on the basis of the observation that (GlcNAc)2 is fermented less efficiently by S. marcescens 2170 in the absence of chitobiase.Citation21) Both GlcNAc and (GlcNAc)2 induce chitobiase production, but (GlcNAc)2 is a more effective inducer than GlcNAc.Citation21) Therefore, the uptake of (GlcNAc)2 plays an important role in the induction of chitobiase as well as that of chitinases and CBP21. In addition, it was shown that the chitobiase-deficient mutant TT327, which had a transposon inserted in the 5′ untranslated region (5′ UTR) of the chiPQ-ctb cluster encoding putative chitoporin, putative lipoprotein, and chitobiase, was also chitinase deficient.Citation22) The ectopic expression of the 5′ UTR of chiP restored chitinase production. The region from −59 to + 3 bp relative to the translation start codon of chiP is the minimum requirement for the production of chitinases. In this study, we found that a small RNA (sRNA), ChiX, is involved in the regulation of chitinase production by the chiP 5′ UTR. The chiP 5′ UTR has a 17-nucleotide Shine-Dalgarno (SD) sequence, which perfectly complements the single-stranded region of ChiX. The complementary region of the chiP 5′ UTR containing 12 nucleotides is also identical to the 5′ UTR of chiR, which encodes the essential transcriptional factor for the induction of all chitinases and CBP21 production. We demonstrate that ChiX represses the expression of chiR. We also discuss the coordinated regulation of the expression of chiPQ-ctb and chiR by ChiX sRNA.
Materials and methods
Bacterial strains, plasmids, and culture conditions
All of the S. marcescens and Escherichia coli strains and plasmids used in this study are listed in Table . S. marcescens 2170 and the chiX deletion mutant, ∆chiX, were grown at 33 °C with aeration in a yeast extract-supplemented minimal (YEM) medium containing various carbon sources.Citation23) The yeast extract concentration in the medium was 0.05%, unless stated otherwise. YEM agar plates containing 0.2% colloidal chitin were used to test for chitinase production. S. marcescens 2170 and ∆chiX mutant harboring plasmids were grown in YEM medium containing kanamycin. E. coli DH5α for DNA cloning was grown at 37 °C in Luria–Bertani medium containing appropriate antibiotics. Growth of bacterial strains was monitored based on the OD660 values determined using a mini-photo 518 photometer (Taitec, Koshigaya, Japan). Antibiotics were used at the following concentrations: ampicillin, 100 μg mL−1; kanamycin, 50 μg mL−1.
Table 1. Bacterial strains and plasmids used in this study.
Isolation of total RNA
Bacterial cultures were mixed with 2 vol. of RNAprotect Bacterial Reagent (Qiagen, Hilden, Germany) and incubated for 5 min at room temperature. Total cellular RNA was then prepared and treated three times with DNase I using a MasterPure RNA Purification Kit, according to the manufacturer’s instructions (Epicentre, Madison, WI).
5′-Rapid amplification of cDNA ends (5′-RACE)
S. marcescens 2170 was grown at 33 °C with aeration in YEM medium containing 0.1% (GlcNAc)2 until the mid-exponential phase. Total RNA was prepared as described above. The 5′-RACE assay was performed essentially as described previously.Citation24) Each RNA sample was treated with tobacco acid pyrophosphatase (TAP, Epicentre, Madison, WI) to convert 5′ triphosphates into monophosphates. The RNA adapter (5′-RACE adapter, 5′-GAUAUGCGCGAAUUCCUGUAGAACGAACACUAGAAGAAA-3′) was ligated to the 5′-end of RNA using T4 RNA ligase. Each cDNA was obtained using the ThermoScript™ RT-PCR System (Invitrogen, Carlsbad, CA) with the primers: chiX3′RT, chiR3′RT, cbp3′RT, chiA3′RT, chiB3′RT, and chiC3′RT. The cDNA products were amplified by PCR with the primers: 5′-adapter and chiX-3′PCR, chiR-3′PCR, cbp-3′PCR, chiA-3′PCR, chiB-3′PCR, or chiC-3′PCR. The primers are listed in Table S1. The PCR products were separated on an agarose gel and purified, and then cloned using a TOPO TA cloning kit (Invitrogen, Carlsbad, CA). Four to 12 plasmids were prepared from each of the transformants and sequenced using a CEQ8000 DNA Analysis System (Beckman Coulter, Fullerton, CA).
Reverse transcription-PCR (RT-PCR)
S. marcescens strains were grown at 33 °C with aeration in YEM medium containing various carbon sources until the exponential phase. Total RNA was prepared as described above. RT-PCR was conducted using a Titan One Tube RT-PCR Kit (Roche Applied Science, Mannheim, Germany) according to the manufacturer’s protocol. The primers used for detecting transcripts of chiX (primers chiX-RT-F and chiX-RT-R), chiR (primers chiR-RT-F and chiR-RT-R), chiP (primers chiP-RT-F and chiP-RT-R), cbp (primers cbp-RT-F and cbp-RT-R), chiA (primers chiA-RT-F and chiA-RT-R), chiB (primers chiB-RT-F and chiB-RT-R), and chiC (primers chiC-RT-F and chiC-RT-R) are listed in Table S1. The gene encoding isocitrate dehydrogenase, icd, was used as a control for RT-PCR. The primers chiPF2, chiQR, chiQF2, and ctbR were used to detect the chiPQ-ctb region. Samples were analyzed by agarose gel electrophoresis on a 2% agarose gel, and cDNA was visualized by ethidium bromide staining. The signals were visualized with a LAS3000 system (Fuji Film, Tokyo, Japan).
Cloning of the chiX gene
The plasmid pChiX was constructed by PCR amplification of the chiX gene from S. marcescens 2170 chromosomal DNA with KOD polymerase and the primers chiX-FBam and chiX-RBam (Table S1). The amplified 488-bp DNA fragment, which included the ybaK-ybaP intergenic region (IGR), was ligated into the BamHI site of pACYC177, and the nucleotide sequence of the inserted DNA was determined.
Secondary structure prediction of ChiX by mfold and sequence analysis
The secondary structures of ChiX and its mutants were predicted using the mfold software (http://mfold.rna.albany.edu/).Citation25) Putative promoters were identified using the Neural Network Promoter Prediction Program.
Chemicals
N-acetylglucosamine was purchased from Seikagaku Corporation (Tokyo, Japan). N,N′-diacetylchitobiose was kindly provided by Kazuhide Totani (National Institute of Technology, Ichinoseki College, Ichinoseki, Japan) and Yaizu Suisankagaku Industry (Shizuoka, Japan). Water-soluble chitin was kindly provided by Yaizu Suisankagaku Industry (Shizuoka, Japan). Colloidal chitin was prepared from powdered chitin purchased from Funakoshi Chemical Co. (Tokyo, Japan) according to the method described by Jeuniaux.Citation26) 4-Methylumbeliferyl N-acetyl-β-d-glucosaminide (4-MU-GlcNAc) was purchased from Sigma-Aldrich (St. Louis, MO).
SDS–PAGE and activity staining
SDS–PAGE was carried out as described by Laemmli using 12.5% polyacrylamide gels under reducing conditions.Citation27) Proteins separated by SDS–PAGE were stained with Coomassie Brilliant Blue R-250. To detect the chitobiase activity after SDS–PAGE, the renaturation of the enzymes in the gel and detection of the chitobiase activity (using an agarose gel sheet containing 0.1 mM 4-MU-GlcNAc) were conducted as described previously.Citation21) To detect chitinase activity after SDS–PAGE, the renaturation of the enzymes in the gel and detection of the chitinase activity using an agarose gel sheet were performed as described previously, with one alteration, 0.1% water-soluble chitin was included in the agarose gel sheet instead of glycol chitin.Citation28)
Results
5′ UTR of chiP induces the production of chitinases and CBP21
Previously, we reported that the chitobiase-deficient mutant TT327, where the transposon was inserted at a position located −59 bp relative to the translation start codon of chiP (formerly ybfM), did not exhibit any chitinase activity on a colloidal chitin plate. However, the production of chitinase by TT327 was recovered by plasmids carrying the predicted 5′ UTR and the first several codons of chiP, such as pkrTer−137 + 117-F, carrying the region from −137 to +117 bp relative to the translation start codon of chiP (the first 39 codons). The minimum requirement region for chitinase production in TT327 was −59 to +3 bp, which corresponds to the ATG start codon.Citation22) To examine whether the effect of the 5′ UTR in chiP extends to the production of all three chitinases and CBP21, we cultured TT327 harboring a control vector or plasmid, carrying part of the 5′ UTR and open reading frame (ORF) of chiP in YEM medium supplemented with (GlcNAc)2 or glycerol. The supernatant was then analyzed by SDS–PAGE (Fig. ). We found that all three chitinases (ChiA, ChiB, and ChiC1) and CBP21 were induced by (GlcNAc)2, whereas they were not induced by glycerol in the wild-type strain.Citation13) The mutant TT327 harboring control vector pTH18kr did not produce any chitinases and CBP21 grew in the presence of either (GlcNAc)2 or glycerol. TT327 harboring pTHybfM containing 5′ UTR and the ORF of chiP produced chitinases but also CBP21 in both growth conditions. In addition, TT327 harboring plasmids carrying the 5′ UTR and part of the ORF of chiP in the forward orientation under the control of the lac promoter produced all three chitinases and CBP21, even in un-induced conditions. The plasmids carrying the 5′ UTR of chiP in the opposite orientation of the lac promoter, i.e. pkrTer−137 + 117-R and pkrTer−75 + 117-R, did not produce any chitinases or CBP21. As we reported previously, the length of the cloned region in the plasmids affected chitinase activity. These results indicate that the minimum region required for the production of all chitinases and CBP21 was from −75 to +3 bp relative to the translation start codon of chiP as we predicted based on the previous report.Citation22) The transcriptional start site of chiP was identified previously by 5′-RACE as −136-bp upstream of the ATG start codon of chiP (Fig. S1).Citation22) Therefore, the plasmid used in this study carried the chiP 5′ UTR, and one additional codon or part of its ORF. These results indicate that the expression of the chiP 5′ UTR induces the production of all three chitinases and CBP21.
Fig. 1. Effect of 5′ UTR of chiP on production of chitinases and CBP21.
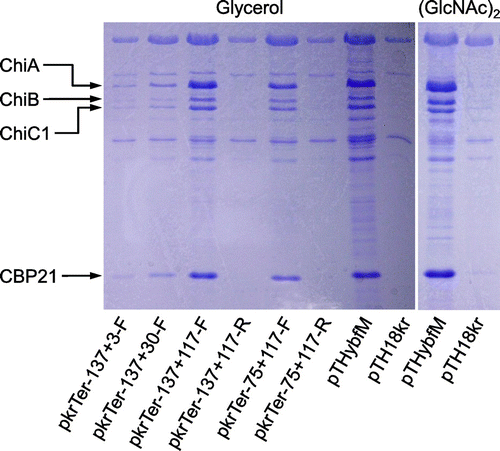
Putative target for the 5′ UTR of chiP
It was difficult to understand how the 5′ UTR of chiP could directly regulate three chitinases and CBP21 production in TT327 even in their un-induced conditions, so we predicted that another factor must influence this phenomenon. In E. coli and Salmonella enterica serovar Typhimurium, it has been reported that a base-pairing small regulatory RNA, ChiX (formerly MicM), interacts with the 5′ UTR of chiP mRNA and the mRNA is degraded.Citation29,30) The nucleotide sequence of the ChiX-binding region in the 5′ UTR of chiP mRNA is conserved in E. coli, Salmonella, Shigella, and also in S. marcescens.Citation30) Therefore, we assumed that a chiX homolog must be present in the genome of S. marcescens 2170. First, we searched for the ChiX homolog and identified it in the S. marcescens Db11 genome database (http://www.sanger.ac.uk/cgi-bin/blast/submitblast/s_marcescens), because the S. marcescens 2170 genome database is not complete. Next, a PCR primer pair was designed based on the nucleotide sequence of chiX in S. marcescens Db11. The corresponding gene region in the genome of S. marcescens 2170 was amplified and cloned into pACYC177. The chiX homolog was identified in the cloned DNA region. Fig. shows the secondary structure of ChiX sRNA in S. marcescens 2170, which was predicted by mfold. ChiX (accession no. LC054829) was predicted to have a length of 88 nucleotides and to contain three stem-loop structures, including a rho-independent terminator. The 5′ end of ChiX in S. marcescens 2170 was identified by 5′-RACE. Seventeen continuous nucleotides in the single-stranded region between the second stem-loop structure and a terminator of ChiX were complementary to the chiP 5′ UTR, including the presumed SD sequence (Fig. (A)).
ChiX represses the production of chitinases and CBP21
To investigate whether ChiX affects the production of chitinases and CBP21, the plasmid carrying chiX, pChiX, was introduced into the wild-type strain, which was grown on YEM plates supplemented with 0.2% colloidal chitin (Fig. (A)). The wild-type strain harboring the control vector, pACYC177, grew normally and formed a clear zone of colloidal chitin around the colonies, thereby indicating the production of chitinases and the utilization of chitin degradation products. By contrast, the wild-type strain harboring pChiX grew poorly and did not form any clear zones around colonies. Subsequently, these two strains were grown in YEM medium containing 0.1% (GlcNAc)2 and the culture supernatant was analyzed by SDS–PAGE, followed by activity staining analyses to detect chitinases and chitobiase activity (Fig. (B)–(D)). In the wild-type strain harboring pACYC177, all three chitinases, including ChiC2 (which is the proteolytically processed catalytic domain of ChiC1Citation12)) and CBP21, were detected by SDS–PAGE, while chitinase and chitobiase activities were detected by activity staining analyses. The activities of ChiC1 and ChiC2 were detected on the agarose gel sheet containing water-soluble chitin. However, the activities of ChiA and ChiB were not clearly detectable on the same sheet used for the activity staining analyses (Fig. (C)) because of their low activity against soluble chitin. By contrast, the wild-type strain harboring pChiX did not produce chitinases, CBP21, or chitobiase. These results indicate that ChiX represses the production of all chitinases, CBP21, and chitobiase.
Fig. 3. Repression of Chitinases, CBP21, and Chitobiase production by ChiX.
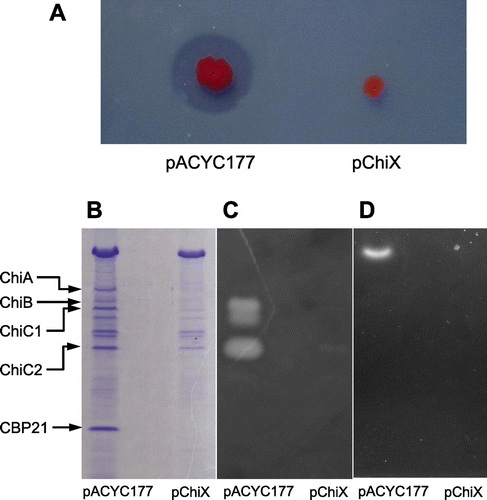
chiPQ-ctb operon
ChiX repressed chitobiase production (Fig. (D)). A 17 base segment in the single-stranded region of ChiX complemented the 5′ UTR of chiP, including its SD sequence (Fig. (A)). The chitobiase gene is located downstream from chiQ and the genomic organization of the chiP, chiQ, and ctb genes has been predicted to comprise a chiPQ-ctb operon.Citation22) To examine whether chiP, chiQ (formerly ybfN), and ctb comprise an operon, we conducted RT-PCR to amplify the chiP-chiQ, chiQ-ctb, and chiP-ctb regions when grown in YEN medium supplemented with (GlcNAc)2. In the early exponential phase of growth, each region was amplified by RT-PCR (Fig. S2). These results indicate that chiP-chiQ-ctb does comprise an operon.
The secondary target of ChiX is the 5′ UTR of chiR
Similar to ChiX in E. coli and Salmonella, the ChiX in S. marcescens 2170 was predicted to be an antisense small RNA responsible for the regulation of chiP expression. In addition, Serratia ChiX repressed the production of all chitinases and CBP21. This result suggested two possibilities: ChiX might base pair with the transcripts of all chitinases and CBP21 genes individually, or the transcript of the chiR gene, which is the transcriptional activator of all chitinases and CBP21 expression. Therefore, we first identified the transcription start site of each gene by 5′-RACE (Fig. S1) and then searched for the complementary sequence of ChiX in each transcript. The ChiX complementary sequence was not present in the 5′ UTR of the chitinases, the cbp gene, or in their ORFs. However, 12 continuous nucleotides, including the presumed SD sequence in chiR 5′ UTR, complemented the ChiX single-stranded region located immediately upstream of the rho-independent terminator, which overlapped with the complementary sequence of the chiP 5′ UTR (Fig. (B)). Therefore, we analyzed the effect of chiX overexpression on the gene expression levels of all chitinases, CBP21, and ChiR by RT-PCR (Fig. ). Strains harboring the control vector or chiX plasmid were grown in YEM medium supplemented with (GlcNAc)2. Transcripts of all chitinase genes, cbp, chiR, chiP, and chiX, were detected in the wild-type strain harboring the control vector, pACYC177, in the presence of (GlcNAc)2. chiP transcripts were more abundant than those of chiR or chiX in a medium containing (GlcNAc)2. The mRNA levels of chiR, chiP, chiA, chiB, chiC, and cbp in the chiX overexpression background were significantly lower than those in the control. Multicopy chiX was expressed ectopically in the chiX overexpression strain, but the chiX RNA level was only slightly lower than that in the control. The direct targets of ChiX were predicted to be the chiR and chiP 5′ UTRs based on their overlapping complementary nucleotide sequences. The transcript abundance of chiP decreased significantly, whereas that of chiR was not detected with chiX overexpression. These results suggest that ChiX represses chitinase and CBP21 gene expression via the posttranscriptional repression of chiR.
Fig. 4. RT-PCR Analysis of chiP, chiR, chiX, and Chitinase and CBP21 genes.
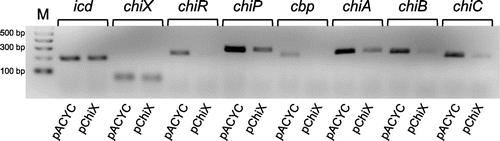
∆chiX mutant produces chitinases, CBP21, and chitobiase without induction
Overexpression of the 5′ UTR of chiP, including the ChiX complementary sequence, led to the production of chitinases and CBP21 (Fig. ), whereas the overexpression of chiX suppressed their production (Fig. ). To identify the correlations between the expression of chiX, chiP, and chiR, the wild-type strain was grown in YEM medium containing glucose, glycerol, GlcNAc, and (GlcNAc)2 to detect chiX, chiR, and chiP transcripts, as well as the production of all chitinases, CBP21, and chitobiase (Fig. ). The chiP transcript level was low in the GlcNAc-supplemented medium, but significant levels were detected in the medium supplemented with (GlcNAc)2 (Fig. (D)). The chiP transcript level was strongly correlated with the production of chitobiase (Fig. (C)). chiR was expressed only in the medium supplemented with (GlcNAc)2 (Fig. (D)). chiR expression level was correlated with the production of all chitinases and CBP21 (Fig. (A), (B) and (D)). However, ChiX was detected in all growth conditions (Fig. (D)). In addition, as shown in Fig. , the expression of chiP 5′ UTR led to the production of all chitinases and CBP21 even in un-induced conditions. Therefore, we hypothesized that ChiX is expressed constitutively and repressed by chiR expression due to base pairing with the 5′ UTR; thus, chiR can be expressed only when chiP is expressed and the chiP transcript sequesters ChiX.
Fig. 5. Effect of various carbon sources on the production of chitinases, CBP21, and chitobiase, and the expression of chiR, chiP, and chiX in wild-type strain and ∆chiX mutant.
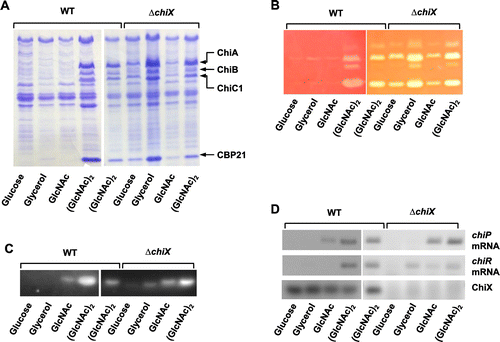
To confirm this hypothesis, a chiX deletion mutant, ∆chiX, was grown in YEM medium containing various carbon sources (Fig. ). The chitobiase activity in the ∆chiX mutant was low in the medium containing glucose and glycerol (Fig. (C)), but the chiP transcript was not detected in these conditions (Fig. (D)). Therefore, the expression of chiP in the ∆chiX mutant was expected to be very low in these conditions. The chitobiase production and expression levels of chiP in the ∆chiX mutant were low in the GlcNAc-supplemented medium, but significant levels were detected in the medium supplemented with (GlcNAc)2 (Fig. (D)). The expression of chiR and the production of chitinases and CBP21 in the ∆chiX mutant occurred simultaneously in the medium containing (GlcNAc)2, but also in a medium with glucose, glycerol, and GlcNAc (Fig. (A), (B) and (D)). These results suggest that: (i) chiR is transcribed constitutively, (ii) chiX is also transcribed constitutively, (iii) ChiX represses the translation of chiR in these conditions; and (iv) a high amount of chiP transcripts will sequester ChiX and/or lead to the degradation of ChiX, thereby allowing chiR translation.
Discussion
S. marcescens produces three types of chitinases and CBP21. The production of different types of chitin-degrading enzymes yields a strong synergistic effect during the degradation of insoluble crystalline chitin by S. marcescens.Citation13,16,18) The production of all of these enzymes is co-regulated and induced by (GlcNAc)2, which is the main chitin degradation product of Serratia chitinases. A large proportion of the proteins secreted in the culture when S. marcescens is grown in medium containing chitin are these chitin-degrading enzymes. Previously, we have studied the regulatory mechanism involved in the expression of the chitinase system in S. marcescens, thereby identifying two factors, ChiR and the 5′ UTR of chiP, which affect the production of chitin-degrading enzymes. Transposon insertion into the 5′ UTR of chiP abolishes chitinase production,Citation22) whereas the ectopic expression of the 5′ UTR of chiP restores chitinase production. The results of the present study indicate that the 5′ UTR of chiP is essential for the production of CBP21 as well as for all chitinases (Fig. ). Furthermore, we found that the correlation between chiR and the 5′ UTR of chiP during the expression of the chitinase system is attributable to ChiX sRNA.
Previously, we demonstrated that ChiP is an outer membrane porin, which is essential for the incorporation of chitin oligosaccharides larger than (GlcNAc)3, and that ChiP is necessary for the efficient incorporation of (GlcNAc)2 in the presence of lower (GlcNAc)2 concentrations.Citation31) Disruption of the (GlcNAc)2-specific PTS permease in the inner membrane, which is encoded by the chb operon, dramatically decreases the production of chitinases and CBP21.Citation20) Transposon insertion into the 5′ UTR of chiP yields a chitinase-deficient phenotype, whereas insertion into the chiP, chiQ, or ctb ORFs, which comprise a tricistronic operon, does not affect chitinase production.Citation22) Therefore, the functions of ChiP, ChiQ, and chitobiase do not affect the expression of the chitinase system, and only the 5′ UTR of chiP is an essential factor. In E. coli and Salmonella, chiP is kept silent by base paring with ChiX sRNA at the 5′ UTR of chiP mRNA.Citation29,30) Rasmussen et al. showed that the chiP and chiX genes are conserved and that their complementarity is retained in a wide range of enterobacteria including S. marcescens.Citation30) Thus, ChiX sRNA was suggested to be the key factor responsible for the expression of the chitinase system in S. marcescens. We demonstrated that the ectopic expression of chiX repressed the expression of the chitinase system in S. marcescens (Fig. ). By contrast, the deletion of chiX induced the chitinase system even in un-induced conditions (i.e. grown in the presence of glycerol or glucose) (Fig. ). In most cases, antisense sRNA inhibits the translational initiation of the target mRNA by base paring with a complementary sequence near the translational initiation site, including the SD sequence.Citation32,33) Overall, these results suggest that ChiX is critical for the regulation of the chitinase system by base pairing with the target mRNAs.
In E. coli and Salmonella, ChiX is trapped by an extended target sequence in the chbBC mRNA IGR of the chb operon, which encodes genes related to (GlcNAc)2 transport and its metabolism.Citation29,30) However, the corresponding target sequence, which is the complementary sequence of Serratia ChiX, is not present in the chb operon of S. marcescens. There are several differences between the S. marcescens and E. coli chb operons. Unlike the E. coli chbBCARFG operon, the S. marcescens chb operon does not include a chbA homolog encoding a putative (GlcNAc)2-specific enzyme IIA or a chbF homolog encoding a putative phosphochitobiase. However, a bglA homolog encoding a putative 6-phospho-β-glucosidase is located between chbC and chbR.Citation21) The nucleotide sequence of chbB-chbC IGR in E. coli is entirely different from the sequence of the corresponding region in S. marcescens, and a complementary sequence to ChiX is not present in S. marcescens. In E. coli and Salmonella, the transcript of the chbB-chbC IGR acts as decoy target for ChiX.Citation29,30) ChiX is destabilized and degraded by base pairing with the transcript of chbB-chbC IGR, and thus, the transcription of the chb operon leads to the derepression of chiPQ mRNA in E. coli and Salmonella. In the case of S. marcescens, Serratia ChiX inhibits the translation of chiP by base pairing, but it cannot base pair with the chb transcript in the same way as E. coli ChiX. This indicates that the function of Serratia ChiX is different from that of E. coli and Salmonella. We discovered that another target for Serratia ChiX is the chiR 5′ UTR, which includes the SD sequence (Figs. and ). The chiR encoding the LysR-type transcriptional activator ChiR is essential for the production of all chitinases and CBP21 in S. marcescens. Therefore, in S. marcescens, chitin degradation (regulation by chiR) and the uptake of chitin degradation products (chiPQ-ctb operon) are regulated in a coordinated manner by ChiX. The coordinated and prompt regulation of these two systems is critical for the effective utilization of chitin in S. marcescens.
After S. marcescens recognizes chitin due to the uptake of (GlcNAc)2, the chitin degradation and degradation product uptake systems are induced simultaneously. (GlcNAc)2 is the minimum-inducing factor during the production of all chitinases and CBP21. Thus, we hypothesized that a specific substrate generated during or after the uptake of (GlcNAc)2, such as (GlcNAc)2-6P, must be involved in the activation of chitinases and CBP21 production via ChiR. A defective chiX gene abrogated chitinase induction by (GlcNAc)2 but allowed the production of chitinases and CBP21, even in the medium containing glycerol (Fig. ). The production of chitinases and CBP21 in the medium containing glycerol also occurred in the chiP 5′ UTR overexpression strain (Fig. ). Therefore, the involvement of (GlcNAc)2 in the induction of chitinases and CBP21 occurred upstream of the chiP-ChiX-chiR regulation cascade.
Fig. shows a model of the post-transcriptional regulation of the chiPQ-ctb operon and chiR by ChiX. ChiX is expressed constitutively and represses the translational initiation of chiR mRNA by base paring with the ribosome binding site, thereby destabilizing the chiR mRNA. Serratia ChiX is suggested to be an Hfq-dependent antisense sRNA in a similar manner to those of E. coli and Salmonella.Citation29,30) Hfq may bind to Serratia ChiX and chiR mRNA, which could involve the ChiX-mediated decay of the chiR mRNA. When the chiPQ-ctb operon is fully expressed by (GlcNAc)2, ChiX binds to the chiP 5′ UTR, which is presumably mediated by Hfq. In this case, base pairing between ChiX and the chiP 5′ UTR relieves repression on the translational initiation of the chiR mRNA. Thus, the chiR mRNA accumulates and ChiR is synthesized, which activates the production of chitin-degrading enzymes. In contrast to the ChiX found in E. coli and Salmonella, Serratia ChiX is not trapped by chb mRNA. The region of ChiX-chiP interaction is slightly longer than that of ChiX-chiR, but we do not know whether the activity of ChiX is controlled by a mechanism that involves an RNA trap. Our proposed model for regulation by ChiX sRNA in S. marcescens is based on the experimental results obtained in this study. Further research is necessary to understand the mechanism responsible for the regulation cascade mediated by ChiX in S. marcescens.
Fig. 6. Model for the regulation of the chitinase system by ChiX in S. marcescens 2170.
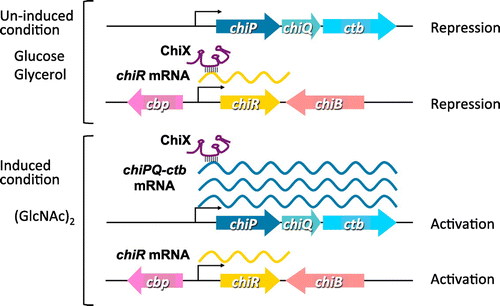
Author contributions
K.S. conceived the project, designed the experiments, and wrote the manuscript. H.S. and T.W. involved in study conception, interpretation of data, and critical reading of the draft. M.S., N.S., C.O., and H.M. performed the experiments.
Supplemental material
The supplemental material for this article is available at http://dx.doi.org/10.1080/09168451.2015.1083399.
Funding
This work was supported by the JSPS KAKENHI [grant number 25450097].
Fig_S2_2_.ppt
Download MS Power Point (370 KB)Fig_S1_2_.ppt
Download MS Power Point (196.5 KB)Table_S1.doc
Download MS Word (28 KB)Acknowledgments
We thank Prof. Kazuhide Totani, National Institute of Technology, Ichinoseki College, for providing N,N′-diacetylchitobiose, Yaizu Suisankagaku Industry for N,N′-diacetylchitobiose and water-soluble chitin.
Disclosure statement
No potential conflict of interest was reported by the authors.
Notes
Abbreviations: GlcNAc, N-acetylglucosamine; (GlcNAc)2, N,N′-diacetylchitobiose; 5′ UTR, 5′ untranslated region; RT-PCR, reverse transcription polymerase chain reaction; RACE, rapid amplification of cDNA ends; sRNA, small RNA; IGR, intergenic region; YEM medium, yeast extract-supplemented minimal medium; CBM, carbohydrate binding module; PTS, phosphoenolpyruvate-dependent sugar phosphotransferase system.
References
- Alam M, Mizutani T, Isono M, et al. Three chitinase genes (chiA, chiC and chiD) comprise the chitinase system of Bacillus circulans WL-12. J. Ferment. Bioeng. 1996;82:28–36.10.1016/0922-338X(96)89450-5
- Shiro M, Ueda M, Kawaguchi T, et al. Cloning of a cluster of chitinase genes from Aeromonas sp. No. 10S-24. Biochim. Biophys. Acta. 1996;1305:44-48.10.1016/0167-4781(95)00213-8
- Tsujibo H, Orikoshi H, Shitomi K, et al. Characterization of chitinase C from a marine bacterium, Alteromonas sp. strain O-7, and its corresponding gene and domain structure. Appl. Environ. Microbiol. 1998;64:472–478.
- Miyashita K, Fujii T, Watanabe A, et al. Nucleotide sequence and expression of a gene (chiB) for a chitinase from Streptomyces lividans. J. Ferment. Bioeng. 1997;83:26–31.10.1016/S0922-338X(97)87322-9
- Kawase T, Yokokawa S, Saito A, et al. Comparison of enzymatic and antifungal properties between family 18 and 19 chitinases from S. coelicolor A3(2). Biosci. Biotechnol. Biochem. 2006;70:988–998.10.1271/bbb.70.988
- Watanabe T, Kimura K, Sumiya T, et al. Genetic analysis of the chitinase system of Serratia marcescens 2170. J. Bacteriol. 1997;179:7111–7117.
- Dauenhauer SA, Hull RA, Williams RP. Cloning and expression in Escherichia coli of Serratia marcescens genes encoding prodigiosin biosynthesis. J. Bacteriol. 1984;158:1128–1132.
- Hejazi A, Falkiner FR. Serratia marcescens. J. Med. Microbiol. 1997;46:903–912.10.1099/00222615-46-11-903
- Suzuki K, Suzuki M, Taiyoji M, et al. Chitin binding protein (CBP21) in the culture supernatant of Serratia marcescens 2170. Biosci. Biotechnol. Biochem. 1998;62:128–135.10.1271/bbb.62.128
- Henrissat B. A classification of glycosyl hydrolases based on amino acid sequence similarities. Biochem. J. 1991;280:309–316.10.1042/bj2800309
- Henrissat B, Bairoch A. New families in the classification of glycosyl hydrolases based on amino acid sequence similarities. Biochem. J. 1993;293:781–788.10.1042/bj2930781
- Suzuki K, Taiyoji M, Sugawara N, et al. The third chitinase gene (chiC) of Serratia marcescens 2170 and the relationship of its product to other bacterial chitinases. Biochem. J. 1999;343:587–596.10.1042/bj3430587
- Suzuki K, Sugawara N, Suzuki M, et al. Chitinases A, B, and C1 of Serratia marcescens 2170 produced by recombinant Escherichia coli: enzymatic properties and synergism on chitin degradation. Biosci. Biotechnol. Biochem. 2002;66:1075–1083.10.1271/bbb.66.1075
- Hult EL, Katouno F, Uchiyama T, et al. Molecular directionality in crystalline β-chitin: hydrolysis by chitinases A and B from Serratia marcescens 2170. Biochem. J. 2005;388:851–856.10.1042/BJ20050090
- Igarashi K, Uchihashi T, Uchiyama T, et al. Two-way traffic of glycoside hydrolase family 18 processive chitinases on crystalline chitin. Nat. Commun. 2014;5:3975.
- Vaaje-Kolstad G, Westereng B, Horn SJ, et al. An oxidative enzyme boosting the enzymatic conversion of recalcitrant polysaccharides. Science. 2010;330:219–222.10.1126/science.1192231
- Levasseur A1, Drula E, Lombard V, et al. Expansion of the enzymatic repertoire of the CAZy database to integrate auxiliary redox enzymes. Biotechnol. Biofuels. 2013;6:41.10.1186/1754-6834-6-41
- Vaaje-Kolstad G, Horn SJ, van Aalten DM, et al. The non-catalytic chitin-binding protein CBP21 from Serratia marcescens is essential for chitin degradation. J. Biol. Chem. 2005;280:28492–28497.10.1074/jbc.M504468200
- Suzuki K, Uchiyama T, Suzuki M, et al. LysR-type transcriptional regulator ChiR is essential for production of all chitinases and a chitin-binding protein, CBP21, in Serratia marcescens 2170. Biosci. Biotechnol. Biochem. 2001;65:338–347.10.1271/bbb.65.338
- Uchiyama T, Kaneko R, Yamaguchi J, et al. Uptake of N,N′-diacetylchitobiose [(GlcNAc)2] via the phosphotransferase System is essential for chitinase production by Serratia marcescens 2170. J. Bacteriol. 2003;185:1776–1782.10.1128/JB.185.6.1776-1782.2003
- Toratani T, Shoji T, Ikehara T, et al. The importance of chitobiase and N-acetylglucosamine (GlcNAc) uptake in N,N′-diacetylchitobiose [(GlcNAc)2] utilization by Serratia marcescens 2170. Microbiology. 2008;154:1326–1332.10.1099/mic.0.2007/016246-0
- Toratani T, Suzuki K, Shimizu M, et al. Regulation of chitinase production by the 5'-untranslated region of the ybfM in Serratia marcescens 2170. Biosci. Biotechnol. Biochem. 2012;76:1920–1924.10.1271/bbb.120403
- Monreal J, Reese ET. The chitinase of Serratia marcescens. Can. J. Microbiol. 1969;15:689–696.10.1139/m69-122
- Argaman L, Hershberg R, Vogel J, et al. Novel small RNA-encoding genes in the intergenic regions of Escherichia coli. Curr. Biol. 2001;11:941–950.10.1016/S0960-9822(01)00270-6
- Zuker M. Mfold web server for nucleic acid folding and hybridization prediction. Nucleic Acids Res. 2003;31:3406–3415.10.1093/nar/gkg595
- Jeuniaux C. Chitinases. Methods Enzymol. 1966;8:644–650.10.1016/0076-6879(66)08117-5
- Laemmli UK. Cleavage of structural proteins during the assembly of the head of bacteriophage T4. Nature. 1970;227:680–685.10.1038/227680a0
- Watanabe T, Oyanagi W, Suzuki K, et al. Chitinase system of Bacillus circulans WL-12 and importance of chitinase A1 in chitin degradation. J. Bacteriol. 1990;172:4017–4022.
- Figueroa-Bossi N, Valentini M, Malleret L, et al. Caught at its own game: regulatory small RNA inactivated by an inducible transcript mimicking its target. Genes Dev. 2009;23:2004–2015.10.1101/gad.541609
- Rasmussen AA, Johansen J, Nielsen JS, et al. A conserved small RNA promotes silencing of the outer membrane protein YbfM. Mol. Microbiol. 2009;72:566–577.10.1111/mmi.2009.72.issue-3
- Takano S, Honma S, Miura T, et al. Construction and basic characterization of deletion mutants of the genes involved in chitin utilization by Serratia marcescens 2170. Biosci. Biotechnol. Biochem. 2014;78:524–532.10.1080/09168451.2014.882755
- Morita T, Mochizuki Y, Aiba H. Translational repression is sufficient for gene silencing by bacterial small noncoding RNAs in the absence of mRNA destruction. Proc. Nat. Acad. Sci. 2006;103:4858–4863.10.1073/pnas.0509638103
- Bouvier M, Sharma CM, Mika F, et al. Small RNA binding to 5′ mRNA coding region inhibits translational initiation. Mol. Cell. 2008;32:827–837.10.1016/j.molcel.2008.10.027
- Palomar J, Guasch JF, Regué M, et al. The effect of nuclease on transformation efficiency in Serratia marcescens. FEMS Microbiol. Lett. 1990;69:255–258.10.1111/fml.1990.69.issue-3
- Sambrook J, Russell DW. Molecular cloning: a laboratory manual. 3rd ed. Cold Spring Harbor, NY: Cold Spring Harbor Laboratory Press; 2001.