Abstract
Auxin is an important plant hormone essential for many aspects of plant growth and development. Indole-3-acetic acid (IAA) is the most studied auxin in plants, and its biosynthesis pathway has been investigated for over 70 years. Although the complete picture of auxin biosynthesis remains to be elucidated, remarkable progress has been made recently in understanding the mechanism of IAA biosynthesis. Genetic and biochemical studies demonstrate that IAA is mainly synthesized from l-tryptophan (Trp) via indole-3-pyruvate by two-step reactions in Arabidopsis. While IAA is also produced from Trp via indole-3-acetaldoxime in Arabidopsis, this pathway likely plays an auxiliary role in plants of the family Brassicaceae. Recent studies suggest that the Trp-independent pathway is not a major route for IAA biosynthesis, but they reveal an important role for a cytosolic indole synthase in this pathway. In this review, I summarize current views and future prospects of IAA biosynthesis research in plants.
Graphical abstract
Indole-3-acetic acid (IAA), the most important auxin in plants, is mainly synthesized from l-tryptophan via indole-3-pyruvate (IPA) by the TAA and YUC families in Arabidopsis.

In 1880, Charles and Francis Darwin discovered that light was perceived by the tips of grass coleoptiles and proposed that the stimulus was transmitted to the lower tissues to promote a tropic growth toward light.Citation1–3) They collected a growth-promoting substance, later named auxin, from grass coleoptile tips and demonstrated that the phototropic response was caused by the asymmetric distribution of this substance.Citation3) In the 1930s, indole-3-acetic acid (IAA) was identified as the first auxin.Citation2,3) The following decade, Haagen-Smit et al. provided an evidence for the occurrence of IAA in plants.Citation2,4) Since then, several studies have demonstrated that auxin is a key signaling molecule that regulates plant cell division, elongation, and differentiation in a concentration-dependent manner.Citation3,5,6) We now know that this auxin is essential for almost every aspect of plant growth and development, including embryogenesis and tropism.Citation7–9)
Plant biologists have studied extensively the molecular mechanisms undergirding the biosynthesis, inactivation, transport, signal transduction, and biological functions of auxins (Fig. ).Citation3,7,10) Plants have different types of plasma membrane-localized proteins, such as PIN-FORMED (PIN) auxin efflux carriers, AUXIN1/LIKE AUX1 (AUX1/LAX) influx transporters, and B-type ATP-binding cassette (ABCB) transporters for auxin transport (Fig. ).Citation10,11) PIN efflux carriers are thought to play a crucial role in polar auxin transport and the formation of IAA concentration gradients. As for auxin signal transduction, IAA binds to nuclear auxin receptors, TRANSPORT INHIBITOR RESPONSE 1/AUXIN SIGNALING F-BOX (TIR1/AFB) proteins comprising SKP1 (S-PHASE KINASE-ASSOCIATED PROTEIN 1)-CULLIN-F-BOX TIR1 (SCFTIR1) E3 ligase complex, and enhances the interaction of this complex with AUXIN/INDOLE-3-ACETIC ACID (Aux/IAA) transcriptional repressors (Fig. ).Citation10,12,13) This results in the ubiquitination of Aux/IAAs by the SCFTIR1 E3 ligase complex. Finally, the 26S-proteasome-mediated degradation of polyubiquitinated Aux/IAAs induces the expression of various auxin-responsive genes via auxin response factors (ARFs). AUXIN-BINDING PROTEIN 1 (ABP1) has been extensively studied as a plasma membrane-localized auxin receptor, but its putative role has been questioned by recent data.Citation14) To control auxin levels, multiple metabolic reactions including oxidation,Citation15) amino acid conjugation,Citation16) glucosylation,Citation17–19) and methylationCitation20) function to inactivate IAA in plants (Fig. ). Recent studies show that a significant amount of IAA is inactivated to 2-oxindole-3-acetic acid (OxIAA) and then OxIAA-glucoside in Arabidopsis.Citation18,21,22)
Fig. 1. A schematic model of auxin biosynthesis, inactivation, transport, and signal transduction in plants.
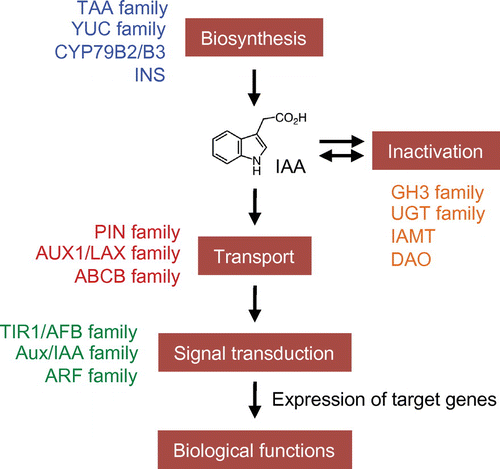
Auxin biosynthesis has been studied for over 70 years. While the complete picture of auxin biosynthesis is yet to be fully elucidated, remarkable progress has been made in the last decade to understand the molecular mechanism of IAA biosynthesis in plants (Fig. ).Citation23–26) Several research groups have independently demonstrated that TRYPTOPHAN AMINOTRANSFERASE OF ARABIDOPSIS (TAA) and YUCCA (YUC) flavin-containing monooxygenase families produce IAA from l-tryptophan (Trp) via indole-3-pyruvate (IPA) in two-step reactions in Arabidopsis (Fig. ).Citation27–29) Genetic and biochemical studies now suggest that the TAA and YUC families play a crucial role in IAA biosynthesis in various plant species, including Arabidopsis, maize, rice, and liverwort.Citation23,24,30) In addition, several lines of evidence indicate that cytochrome P450 monooxygenases CYP79B2 and CYP79B3 catalyze the conversion of Trp into indole-3-acetaldoxime (IAOx) in the first step of both IAA and indole glucosinolate (IG) biosynthesis in Arabidopsis, although the pathway leading to IAA is yet to be fully understood (Fig. ).Citation31,32) Indole-3-acetamide (IAM), indole-3-acetaldehyde (IAAld), and tryptamine (TAM) have been proposed as IAA precursors derived from Trp, but pathways involving these biosynthesis intermediates are still largely unknown (Fig. ).Citation24,33) On the other hand, a recent reverse genetic study suggests that the Trp-independent IAA biosynthesis pathway may play a role in early embryogenesis in Arabidopsis (Fig. ).Citation34) In this review, I focus on current views of IAA biosynthesis in plants. Progress in the regeneration of IAA from its metabolites, e.g. IAA-amino acid conjugates, IAA-methyl ester, indole-3-butylic acid, and IAA-glucoside, can be overviewed in recent published review articles.Citation2,25)
Fig. 2. A schematic model of the IAA biosynthetic pathways and secondary metabolic pathways in Arabidopsis.
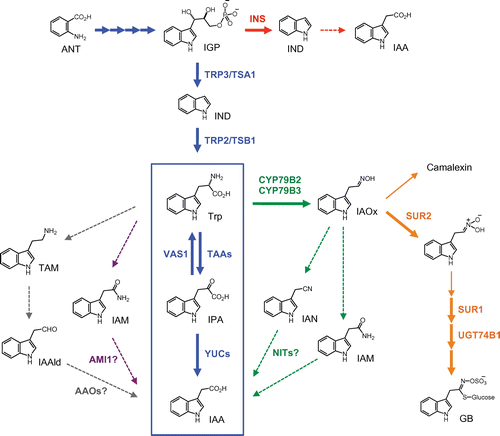
Biosynthesis of IAA from the IPA pathway
There are several key criteria in evaluating the proposed IAA biosynthesis pathways in plants: (1) identification of genes, (2) characterization of enzyme activities, (3) quantification of metabolites, (4) determination of physiological role, and (5) distribution in plant kingdom (Table ). Based on these criteria, the IPA pathway is the first complete and universally conserved IAA biosynthesis pathway in plants (Fig. , Table ).Citation23,24,27) In Arabidopsis, the SHADE AVOIDANCE 3 (SAV3)/WEAK ETHYLENE INSENSITIVE 8 (WEI8)/TRANSPORT INHIBITOR RESPONSE 2 (TIR2) encodes TAA1, which functions in the first step of the IPA pathway.Citation8,35,36) Recombinant TAA1 protein catalyzes the conversion of Trp into IPA in vitro.Citation8,35) TAA1-RELATED 1 (TAR1) and TAR2 probably have redundant roles as Trp aminotransferases in Arabidopsis.Citation8) Endogenous amounts of IAA and IPA are reduced in deficient mutants of the TAA family.Citation27–29) In contrast, induction of TAA1 increases the endogenous amounts of IPA in Arabidopsis.Citation27) The TAA gene family plays a crucial role in plant growth and development. Multiple mutations in TAA1 and TAR genes cause severe auxin-deficient phenotypes in various biological processes, including embryogenesis, flower development, seedling growth, vascular patterning, lateral root formation, tropism, shade avoidance, and temperature-dependent hypocotyl elongation, indicating a fundamental role of the TAA family in plant development (Fig. (A)).Citation8,35,36) Orthologs of the TAA gene family are widely distributed in vascular and nonvascular plants, indicating that TAA genes are evolutionarily conserved in the plant kingdom (Table ).Citation30,37–39) For example, VANISHING TASSEL 2 (VT2) and FISH BONE (FIB) genes are co-orthologs of the TAA family in maize and rice, respectively, and mutations in these genes lead to decreased IAA levels and result in pleiotropic auxin-deficient phenotypes.Citation37,40)
Table 1. Current view of IAA biosynthesis pathways in Arabidopsis.
Fig. 3. IAA biosynthesis gene knockout mutants in Arabidopsis.
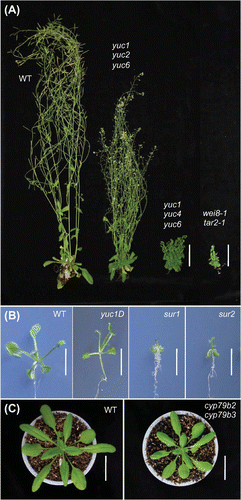
YUC genes were originally discovered by a genetic screen of activation tag lines in Arabidopsis.Citation41) Gain-of-function mutants of YUC1 (yuc1D) showed high-auxin phenotypes such as epinastic cotyledons and long hypocotyls under light conditions (Fig. (B)).Citation41) YUC genes encode flavin-containing monooxygenases and 11 YUC genes are found in Arabidopsis (Table ). Overexpression of each YUC gene leads to increased levels of IAA and results in the high-auxin phenotypes in Arabidopsis, suggesting that YUC proteins catalyze a rate-limiting step in IAA biosynthesis.Citation22,27,41–43) Recombinant YUC1 protein was first characterized to catalyze N-hydroxylation of TAM.Citation23,41) Hence, TAA and YUC families have been placed in independent pathways of IAA biosynthesis.Citation8,31,35) However, recent genetic and biochemical studies have suggested that these two genes function sequentially in the same pathway (Fig. ).Citation27–29) Co-expression of TAA1 and YUC considerably increases both IAA production and high-auxin phenotypes in Arabidopsis.Citation27) Endogenous IPA increases in multiple yuc knockout mutants and decreases in YUC overexpression plants.Citation27,28) Finally, re-evaluation of YUC enzyme activity clarified that recombinant YUC2 proteins catalyze the conversion of IPA to IAA in a NADPH-dependent manner.Citation27) In addition, YUC4 and YUC6 proteins are capable of converting IPA to IAA in vitro.Citation44,45) The oxidative decarboxylation of IPA as a catalytic mechanism has been elucidated using FAD-containing YUC6 proteins.Citation45) This genetic and biochemical evidence has demonstrated that TAA and YUC produce IAA from Trp in the same IPA pathway (Fig. ).Citation23–25,27) IAAld has been proposed as a biosynthesis intermediate in the IPA pathway, as some auxin-producing bacteria have been shown to produce IAA from IPA via IAAld.Citation35,46) However, YUC proteins directly convert IPA to IAA.Citation27,45) Moreover, no significant change in IAAld levels has been observed, despite reduced levels of IPA in wei8-1 tar2-2 double mutants.Citation27) Hence, IAAld is very unlikely to participate in the IPA pathway (Fig. ).Citation23,27) Multiple knockout mutants of YUC genes show severe auxin-deficient phenotypes in various growth and developmental stages, including embryogenesis, flower development, seedling growth, vascular patterning, lateral root formation, and shade avoidance, indicating a crucial role of the YUC family in plant development (Fig. (A)).Citation9,28,42,43) Although some of the yuc single knockout mutants may have subtle phenotypes under certain conditions, single knockout mutants of each YUC gene do not show dramatic reduction in auxin phenotypes,Citation47,48) suggesting functional redundancy in the YUC family.Citation42) Similar to the TAA1/TAR genes, orthologs of YUC genes are widely distributed in vascular and nonvascular plants such as rice,Citation49,50) maize,Citation51,52) petunia,Citation53) and the liverwort Marchantia polymorpha,Citation30) indicating that the YUC gene family is evolutionarily conserved in land plants (Table ).
Multiple knockout mutants of the TAA and YUC gene families showed remarkable reduction of IAA levels and severe auxin-deficient phenotypes in various plant species,Citation29,30,37,40,51,53) indicating an important role of the IPA pathway in IAA biosynthesis. Recent chemical screens of auxin inhibitors uncovered l-kynurenine and l-amino-oxyphenylpropionic acid (l-AOPP) as TAA inhibitors and yucasin as a YUC inhibitor.Citation54–56) Application of l-kynurenine reduces endogenous IAA in Arabidopsis and causes auxin-deficient phenotypes similar to wei8-1- and wei8 tar2-deficient mutants.Citation55) Arabidopsis seedlings treated with l-AOPP also show reduced IAA levels and defects in root elongation, root hair formation, and root gravitropism.Citation54) Yucasin significantly reduces IAA biosynthesis and inhibits gravitropic response in maize coleoptiles.Citation56) These chemical biology-based studies also indicate that the IPA pathway is the main IAA biosynthesis pathway in plants.
The expression patterns of TAA and YUC genes have been extensively studied in plants. In Arabidopsis, the wild-type phenotype of tir2-1 seedlings is restored by expressing a translational fusion of TAA1/TIR2 cDNA with β-glucuronidase (GUS) under the control of the TAA1/TIR2 promoter (pTIR2); GUS expression is detected in cotyledons, young leaves, proximal meristem as well as vascular tissue in shoots, hypocotyls, and roots, suggesting that TAA1 functions broadly in both shoots and roots (Fig. ).Citation36) Consistent with a fundamental role of the TAA gene family in embryogenesis, TAA1 expression is observed in embryos of Arabidopsis, expressing TAA1 cDNA with green fluorescent protein (GFP) under the control of the TAA1 promoter.Citation8) TAA1 protein may also play a role in root gravitropism because the expression of pTIR2:TIR2-GUS is increased in the lower side of the root meristem under gravitropic stimulation.Citation36) Similar to the TAA family in Arabidopsis, FIB genes are broadly expressed in various rice organs, including the vegetative shoot apex, inflorescence apex, leaf blade, leaf sheath, rachis, young flowers, roots, and embryos.Citation40) The expression patterns of YUC genes are distinct and more strictly regulated compared with TAA genes in Arabidopsis. A promoter–GUS expression analysis of YUC genes indicates that YUC1, YUC2, and YUC4 are expressed at relatively high levels in young leaves and vascular tissues and that YUC3, YUC5, YUC7, YUC8, and YUC9 are expressed in root tissues including the proximal meristem and root caps in Arabidopsis seedlings (Fig. ).Citation42,43) YUC1, YUC2, YUC4, and YUC6 expression is also observed in flower organs.Citation42) Consistent with important roles of YUC genes in embryogenesis, YUC1, YUC4, YUC10, and YUC11 are expressed in embryos.Citation9) As YUC proteins catalyze a rate-limiting step in the IPA pathway, the specific expression patterns of YUC gene families demonstrate the importance of this pathway in spatio-temporal IAA biosynthesis in many aspects of plant growth and development.
Fig. 4. TAA1 and YUC expression patterns in pTIR2:GUS and pYUCs:GUS lines.
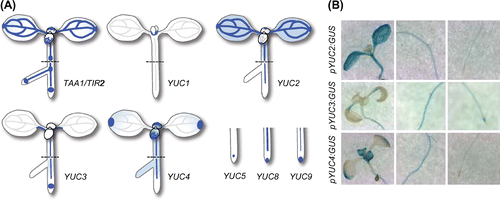
Recent genetic studies have identified various transcription factors that can positively or negatively regulate TAA and YUC genes for spatio-temporal IAA biosynthesis in plants. LEAFY COTYLEDON2 (LEC2), a B3 domain transcription factor, has been reported as a central regulator of embryogenesis.Citation57) Studies have shown that the induction of LEC2 rapidly activates the expression of YUC2 and YUC4 genes in Arabidopsis and that LEC2 can directly bind to the promoter of YUC4.Citation57) In addition to YUC2 and YUC4, LEC2 may also positively regulate YUC1 and YUC10 genes in Arabidopsis,Citation58) although it is still unknown whether LEC2 directly binds to the promoters of YUC1, YUC2, and YUC10. SHORT INTERNODES/STYLISH (SHI/STY) family members function as transcription factors and regulate the development of diverse plant organs, including flowers and leaves.Citation59,60) Induction of the STY1 gene also activates the expression of YUC4 and results in an increase in IAA levels in Arabidopsis seedlings.Citation59,60) STY1 protein can bind directly to the promoter of YUC4.Citation60) STY1 may also positively regulate YUC8.Citation60) INDETERMINATE DOMAIN (IDD) transcription factors IDD14, IDD15, and IDD16 have been reported to function in organ morphogenesis and gravitropic responses.Citation61) Moreover, TAA1 and YUC5 genes have been identified as direct targets of IDD14 and IDD16. Overexpression of IDD14 increases TAA1 and YUC5 expression and increases endogenous IAA accumulation in Arabidopsis seedlings. PHYTOCHROME-INTERACTING FACTOR (PIF) and basic helix-loop-helix transcription factors play a fundamental role in light- and high-temperature-mediated developmental changes. PIF4 and PIF5 bind to the promoter of YUC8, and PIF7 binds to the promoters of YUC8 and YUC9 to increase IAA biosynthesis and promote shade avoidance responses including hypocotyl elongation. PIF4-mediated activation of TAA1 and YUC4 also functions in high-temperature-induced IAA biosynthesis and hypocotyl elongation in Arabidopsis.Citation62) In addition, REVEILLE1 and PLETHORA transcriptional activators have been reported to positively regulate YUC genes in Arabidopsis.Citation63,64) In contrast, the SPOROCYTELESS/NOZZLE (SPL/NZZ) transcription factor, a regulator of sporogenesis, has been shown to negatively regulate YUC2 and YUC6 genes.Citation65) Although the precise mechanism of YUC suppression is still unknown, SPL/NZZ probably functions in the spatio-temporal synthesis of IAA during sporogenesis. These findings indicate that the TAA and YUC gene families are crucial targets for the regulation of IAA levels in plants under developmental and environmental changes.
A recent study revealed that a pyridoxal phosphate-dependent aminotransferase, VAS1, regulates IAA levels by converting IPA back to Trp in Arabidopsis.Citation66) vas1 knockout mutants show increased levels of IAA and the ethylene precursor 1-aminocyclopropane-1-carboxylate coupled with high-auxin phenotypes. VAS1 presumably uses the ethylene precursor methionine as an amino donor and IPA as an amino acceptor to produce Trp and 2-oxo-4-methylthiobutyric acid. The discovery of VAS1 provides novel insight into the coordinated regulation of two-plant hormone biosynthesis.
Biosynthesis of IAA from the IAOx pathway in Arabidopsis
Many genetic and biochemical studies support an auxiliary role of the IAOx pathway in IAA biosynthesis in Arabidopsis.Citation31,32,67) CYP79B2 and CYP79B3 catalyze the conversion of Trp to IAOx in the initial step of the IAOx pathway (Fig. ).Citation32,68,69) In Arabidopsis, IAOx is a common precursor of IAA and secondary metabolites, including glucobrassicin (GB) and camalexin (Fig. ).Citation31,32,70) The IAOx pathway may be a species-specific pathway in Brassicaceae plants, as CYP79B genes are largely limited in this family (Table ).Citation31,71) IG biosynthesis mutants, superroot 1 (sur1), superroot 2 (sur2)/cyp83b1, and ugt74b1, show elevated IAA and IAOx levelsCitation22,31) and high-auxin phenotypesCitation72–74) due to increased metabolic flux from IG to IAA (Fig. (B)), suggesting a metabolic link between IAA and IG biosynthesis. CYP79B2 and CYP79B3 were discovered by a screen of Arabidopsis cDNA-encoding enzymes capable of metabolizing a toxic analog of Trp in budding yeastCitation68) or by characterization of Arabidopsis CYP79 homologs.Citation69) Both CYP79B2 and CYP79B3 can catalyze the conversion of Trp to IAOx in vitro.Citation68) These cytochrome P450 monooxygenases are responsible for the production of IAOx in Arabidopsis since endogenous IAOx was not detected in cyp79b2 cyp79b3 double knockout mutants.Citation31) Overexpression of CYP79B2 increases endogenous IAA levels and results in high-auxin phenotypes such as elongated hypocotyls,Citation32) indicating that CYP79B2 catalyzes a rate-limiting step in the IAOx pathway.
Analysis of CYP79B2 promoter–GUS reporter gene fusions suggests that the expression of this gene is relatively high in young roots, cotyledons, and vascular tissues.Citation69) The phenotypes of cyp79b2 cyp79b3 double mutants are subtle; seedlings are slightly smaller than those of wild-type plants under normal growth conditions (Fig. (C)). However, cyp79b2 cyp79b3 double mutants show shorter hypocotyls and smaller cotyledons compared to wild-type plants under high-temperature conditions.Citation31,32) Hence, it has been suggested that the IAOx pathway plays an important role under high-temperature conditions. Consistent with this hypothesis, PIF4 activates CYP79B2 genes as well as TAA1 and YUC4 genes under elevated temperatures.Citation62) A recent study demonstrated that high temperature induces microRNA, miR10515, reduces expression levels of SUR1, and increases IAA levels by blocking IG biosynthesis.Citation67) The low-auxin phenotypes of miR10515 knockout mutants become more severe at higher temperatures, suggesting biological relevance of the IAOx pathway in Arabidopsis.
The underlying pathway from IAOx to IAA remains to be elucidated (Fig. ). IAM and indole-3-acetonitrile (IAN) have been suggested as possible precursors because these two precursors show metabolic profiles similar to IAOx.Citation31) For instance, endogenous levels of IAM and IAN (in addition to IAOx) were remarkably reduced in cyp79b2 cyp79b3 mutants. In addition, 13C was efficiently incorporated into IAM, IAN, and IAA when cyp79b2 cyp79b3 double mutants were fed with 13C-labeled IAOx. The NITRILASE (NIT) family of enzymes has been proposed to convert IAN to IAA in Arabidopsis and maizeCitation75–78) (Table ). However, recent studies suggest that IAN and NIT enzymes may play more predominant roles in IG metabolism, camalexin homeostasis, and cyanide detoxification rather than IAOx-dependent IAA biosynthesis in Arabidopsis.Citation24,70,79) Further molecular genetics investigation of the IAOx pathway is expected to understand the physiological roles of this pathway.
A previous study has demonstrated that CYP79A2, a homolog of CYP79B2 and CYP79B3 in Arabidopsis, can catalyze the conversion of Phe to phenyl acetaldoxime (PAOx) with narrow substrate specificity in vitro.Citation80) Here, overexpression of CYP79A2 significantly increased phenyl glucosinolate levels in Arabidopsis. In addition, endogenous GB levels are shown to be markedly reduced (<0.01%) in cyp79b2 cyp79b3 double mutants.Citation32) Taken together, these results suggest that IGs are largely produced by CYP79B2 and CYP79B3 in Arabidopsis. Interestingly, Irmisch et al. recently reported that maize CYP79A61 could produce both PAOx and IAOx in heterologous systems and might contribute to plant defense and auxin formation.Citation81) This finding suggests that the IAOx pathway might be more widely distributed in the plant kingdom than is currently thought. Thus, it will be crucial to further investigate IAOx-dependent IAA biosynthesis in maize and similar systems.
Other proposed IAA biosynthesis pathways from Trp
Many auxin-producing bacteria possess iaaM and iaaH genes, encoding TRYPTOPHAN 2-MONOOXYGENASE (iaaM) and INDOLE-3-ACETAMIDE HYDROLASE (iaaH), respectively, to produce IAA from Trp via IAM in two-step reactions.Citation46) The enzyme iaaM catalyzes the conversion of Trp to IAM and iaaH converts IAM to IAA. It has been proposed that plants also produce IAA from the IAM pathway similar to auxin-producing bacteria (Fig. ).Citation33,82) IAM has been detected in higher plants including maize and rice, which do not produce IAOx (Table ).Citation25,33) In addition, overexpression of iaaM gene increases IAA levels and results in the high-auxin phenotypes in plants,Citation83) suggesting that plants are capable of converting IAM to IAA in vivo. Previous studies have demonstrated that Arabidopsis AMIDASE 1 (AtAMI1) and its ortholog in tobacco (Nicotiana sp.) are capable of converting IAM to IAA in vitro.Citation33,84) However, no direct evidence has been found for the biological significance of AMI1 in IAA biosynthesis using ami1-deficient mutants or AtAMI1 overexpression plants. In addition, enzymes that catalyze the initial step of the IAM pathway are yet to be identified in plants. Although bioinformatic studies suggest the wide distribution of AMI1 family members in higher plants,Citation33) the present evidence is insufficient to propose the IAM pathway as a major route to IAA biosynthesis in plants.
TAM and IAAld have been suggested as possible precursors of IAA in plants (Fig. ).Citation6) These metabolites have been detected in various plant species.Citation25) In addition, a significant level of deuterium incorporation into TAM and IAAld was observed in pea (Pisum sp.) when treated with deuterium-labeled Trp.Citation85) Based on this evidence, it has been proposed that TAM and IAAld contribute to IAA biosynthesis from Trp in the roots of pea.Citation85) TRYPTOPHAN DECARBOXYLASE (TDC) family enzymes,Citation6,33) which can convert Trp to TAM, have been studied as possible enzymes in this pathway. However, TDC members likely do not contribute to IAA biosynthesis, as overexpression of Catharanthus TDC has been shown to cause increased TAM levels, but not IAA levels, in tobacco.Citation86) ALDEHYDE OXIDASE (AAO) family, which catalyzes the conversion of IAAld to IAA, has also been suggested as an IAA biosynthesis enzyme (Table ).Citation87,88) However, a recent study demonstrated that the loss of AAO activity did not cause auxin-deficient phenotypes in Arabidopsis.Citation27) Thus, it is unlikely that TDC and AAO members contribute directly to IAA biosynthesis in plants.
Trp-independent pathway
For many years, Trp has been proposed as a main precursor for IAA biosynthesis based on biochemical evidence, e.g. feeding of isotope-labeled Trp to plants resulted in the formation of isotope-labeled IAA.Citation6,31) TAA and CYP79B families that metabolize Trp in IAA biosynthesis were then identified in later studies. By contrast, the Trp-independent pathway was proposed as a major route of IAA biosynthesis in maize and Arabidopsis based on metabolite quantification and feeding experiments in Trp biosynthesis mutants.Citation89,90) It has been shown that endogenous amounts of IAA were remarkably higher in Trp biosynthesis mutants than in wild-type plants, when IAA and its metabolites (e.g. IAA-amino acid conjugates and IAA-glucoside) were quantified altogether as IAA using strong base treatment (alkaline hydrolysis of IAA metabolites). In addition, when 15N-labeled anthranilate (ANT) was fed to an Arabidopsis Trp biosynthesis mutant, trp2-1, levels of 15N incorporation were higher for IAA rather than for Trp.Citation90) Taken together, these biochemical findings have led researchers to posit that IAA is mainly produced from Trp precursors, e.g. indole (IND) and indole-3-glycerol phosphate (IGP), but not from Trp (Fig. ). Furthermore, IGP was later proposed as a branch point intermediate in the Trp-independent pathway in Arabidopsis because IAA levels were significantly reduced in antisense IGP synthase (IGS) transgenic plants; yet, levels of IAA were remarkably increased in Trp biosynthesis mutants trp3-1 and trp2-1 when quantified using the alkaline hydrolysis-based quantification method.Citation91) Conversely, Muller et al. reported that the Trp-independent pathway was an artifact by demonstrating that IGP and GB levels were dramatically increased in trp3-1 mutants and that a remarkable increase in IAA levels was formed by degradation of IGP and GB during alkaline hydrolysis-based quantification.Citation92) Since then, it has not been decidedly established whether or not the Trp-independent pathway occurs in plants.Citation6)
In 2015, Yu et al. reported that a significant portion of IAA present after base treatment could be attributed to chemical conversions other than IAA-conjugate hydrolysis.Citation93) Specifically, GB conversion was previously thought to occur at insignificant levels, but may actually account for the majority of IAA released. Moreover, a significant level of IAA was attributed to proteinaceous Trp degradation in this study, further demonstrating that IAA and its metabolite levels in previous reports on the Trp-independent pathway were most likely increased by IAA from chemical conversion of GB and proteinaceous Trp.Citation93) These results weaken the biochemical evidence of a Trp-independent pathway, and instead suggest that IAA is mainly produced from Trp in plants. However, Wang et al. recently reported that cytosol-localized indole synthase (INS), a close paralog of plastid-localized Trp synthase α-subunit domain (TSA)/TRP3, functions in the Trp-independent pathway in Arabidopsis.Citation34) This study demonstrated that INS produces IND as an initial precursor of IAA in the cytosol, although the underlying pathway from IND to IAA has not been solved. Endogenous IAA levels slightly decrease in seedlings of ins mutants, although levels of IAA increase in seedlings of trp3-1 and trp2-1 mutants. Interestingly, ins mutants are not 100% penetrant and adult ins mutants do not display dramatic developmental defects. INS is proposed to function in a parallel pathway with TAA1 to regulate hypocotyl length at high temperature, impairing hypocotyl elongation induced by high temperature in ins-1 mutants, and contributing to an additive effect in wei8-1 mutants. More importantly, INS plays an important role in the establishment of the apical–basal pattern during early embryogenesis, demonstrating that Trp-dependent and Trp-independent IAA biosynthetic pathways coordinate to regulate embryogenesis of higher plants. Further genetic and biochemical investigations of the Trp-independent pathway are expected to understand the role of this pathway in IAA biosynthesis.
Conclusion
In the last two decades, sophisticated genetic studies using Arabidopsis as a model plant system have successfully identified key genes in IAA biosynthesis pathways. In addition, the advancement of LC–MS/MS analysis technologies has greatly improved our ability to quantify extremely low amounts of labile IAA intermediates in plants. IPA has long been proposed as a biosynthesis intermediate of the main auxin biosynthesis pathway in plants, and this idea has been supported by recent genetic and biochemical studies. The occurrence of the IAOx pathway in Arabidopsis suggests the possibility that other unknown species-specific IAA biosynthesis pathways may also exist in the plant kingdom. Wang et al. further illustrated the Trp-independent pathway in auxin biosynthesis. In the future, it will be crucial to further investigate genes, enzymes, and biosynthesis intermediates in the Trp-independent pathway and their physiological roles and distribution in plant kingdom. Elucidating the molecular mechanisms and biochemical processes undergirding auxin biosynthesis, inactivation, and transport will broaden our understanding of how hormones function to regulate plant growth and development.
Funding
This work was supported by the Japan Society for the Promotion of Science (JSPS) KAKENHI [grant number 24370027]; JST PRESTO.
Acknowledgments
I thank Prof. Yunde Zhao and Prof. Ken-ichiro Hayashi for their critical reading of this review.
Disclosure statement
No potential conflict of interest was reported by the author.
References
- Darwin C. The power of movement in plants. London: John Murray; 1880.10.5962/bhl.title.102319
- Enders TA, Strader LC. Auxin activity: past, present, and future. Am. J. Bot. 2015;102:180–196.10.3732/ajb.1400285
- Davies PJ. The plant hormone: their nature, occurrence, and functions. In: Davies PJ, Editor. Plant hormones: biosynthesis, signal transduction, action!. The Netherlands: Kluwer Academic Publishers; 2004;p. 1–15.
- Haagen-Smit AJ, Leech WD, Bergen WR. Estimation, isolation and identification of auxins in plant material. Science. 1941;93:624–625.10.1126/science.93.2426.624
- Perrot-Rechenmann C. Cellular responses to auxin: division versus expansion. Cold Spring Harb. Perspect. Biol. 2010;2:a001446.
- Woodward AW, Bartel B. Auxin: regulation, action, and interaction. Ann. Bot. 2005;95:707–735.10.1093/aob/mci083
- Zhao Y. Auxin biosynthesis and its role in plant development. Annu. Rev. Plant Biol. 2010;61:49–64.10.1146/annurev-arplant-042809-112308
- Stepanova AN, Robertson-Hoyt J, Yun J, et al. TAA1-mediated auxin biosynthesis is essential for hormone crosstalk and plant development. Cell. 2008;133:177–191.10.1016/j.cell.2008.01.047
- Cheng Y, Dai X, Zhao Y. Auxin synthesized by the YUCCA flavin monooxygenases is essential for embryogenesis and leaf formation in Arabidopsis. Plant Cell. 2007;19:2430–2439.10.1105/tpc.107.053009
- Hayashi K. The interaction and integration of auxin signaling components. Plant Cell Physiol. 2012. 53:965–975.10.1093/pcp/pcs035
- Friml J. Subcellular trafficking of PIN auxin efflux carriers in auxin transport. Eur. J. Cell Biol. 2010;89:231–235.10.1016/j.ejcb.2009.11.003
- Dharmasiri N, Dharmasiri S, Estelle M. The F-box protein TIR1 is an auxin receptor. Nature. 2005;435:441–445.10.1038/nature03543
- Kepinski S, Leyser O. The Arabidopsis F-box protein TIR1 is an auxin receptor. Nature. 2005;435:446–451.10.1038/nature03542
- Gao Y, Zhang Y, Zhang D, et al. Auxin binding protein 1 (ABP1) is not required for either auxin signaling or Arabidopsis development. Proc. Nat. Acad. Sci. USA. 2015;112:2275–2280.10.1073/pnas.1500365112
- Zhao Z, Zhang Y, Liu X, et al. A role for a dioxygenase in auxin metabolism and reproductive development in rice. Dev. Cell. 2013;27:113–122.10.1016/j.devcel.2013.09.005
- Staswick PE, Serban B, Rowe M, et al. Characterization of an Arabidopsis enzyme family that conjugates amino acids to indole-3-acetic acid. Plant Cell. 2005;17:616–627.10.1105/tpc.104.026690
- Jackson RG, Kowalczyk M, Li Y, et al. Over-expression of an Arabidopsis gene encoding a glucosyltransferase of indole-3-acetic acid: phenotypic characterisation of transgenic lines. Plant J. 2002;32:573–583.10.1046/j.1365-313X.2002.01445.x
- Tanaka K, Hayashi K, Natsume M, et al. UGT74D1 catalyzes the glucosylation of 2-oxindole-3-acetic acid in the auxin metabolic pathway in Arabidopsis. Plant Cell Physiol. 2014;55:218–228.10.1093/pcp/pct173
- Tognetti VB, Van Aken O, Morreel K, et al. Perturbation of indole-3-butyric acid homeostasis by the UDP-glucosyltransferase UGT74E2 modulates Arabidopsis architecture and water stress tolerance. Plant Cell. 2010;22:2660–2679.10.1105/tpc.109.071316
- Qin G, Gu H, Zhao Y, et al. An indole-3-acetic acid carboxyl methyltransferase regulates Arabidopsis leaf development. Plant Cell. 2005;17:2693–2704.10.1105/tpc.105.034959
- Pencik A, Simonovik B, Petersson SV, et al. Regulation of auxin homeostasis and gradients in Arabidopsis roots through the formation of the indole-3-acetic acid catabolite 2-oxindole-3-acetic acid. Plant Cell. 2013;25:3858–3870.10.1105/tpc.113.114421
- Novák O, Hényková E, Sairanen I, et al. Tissue-specific profiling of the Arabidopsis thaliana auxin metabolome. Plant J. 2012;72:523–536.10.1111/tpj.2012.72.issue-3
- Zhao Y. Auxin biosynthesis. Arabidopsis Book. 2014;12:e0173.10.1199/tab.0173
- Brumos J, Alonso JM, Stepanova AN. Genetic aspects of auxin biosynthesis and its regulation. Physiol. Plant. 2014;151:3–12.10.1111/ppl.12098
- Korasick DA, Enders TA, Strader LC. Auxin biosynthesis and storage forms. J. Exp. Bot. 2013;64:2541–2555.10.1093/jxb/ert080
- Ljung K. Auxin metabolism and homeostasis during plant development. Development. 2013;140:943–950.10.1242/dev.086363
- Mashiguchi K, Tanaka K, Sakai T, et al. The main auxin biosynthesis pathway in Arabidopsis. Proc. Nat. Acad. Sci. USA. 2011;108:18512–18517.10.1073/pnas.1108434108
- Won C, Shen X, Mashiguchi K, et al. Conversion of tryptophan to indole-3-acetic acid by TRYPTOPHAN AMINOTRANSFERASES OF ARABIDOPSIS and YUCCAs in Arabidopsis. Proc. Nat. Acad. Sci. USA. 2011;108:18518–18523.10.1073/pnas.1108436108
- Stepanova AN, Yun J, Robles LM, et al. The Arabidopsis YUCCA1 flavin monooxygenase functions in the indole-3-pyruvic acid branch of auxin biosynthesis. Plant Cell. 2011;23:3961–3973.10.1105/tpc.111.088047
- Eklund DM, Ishizaki K, Flores-Sandoval E, et al. Auxin produced by the indole-3-pyruvic acid pathway regulates development and Gemmae Dormancy in the liverwort Marchantia polymorpha. Plant Cell. 2015;27:1650–1669. doi:10.1105/tpc.15.00065.
- Sugawara S, Hishiyama S, Jikumaru Y, et al. Biochemical analyses of indole-3-acetaldoxime-dependent auxin biosynthesis in Arabidopsis. Proc. Nat. Acad. Sci. USA. 2009;106:5430–5435.10.1073/pnas.0811226106
- Zhao Y, Hull AK, Gupta NR, et al. Trp-dependent auxin biosynthesis in Arabidopsis: involvement of cytochrome P450s CYP79B2 and CYP79B3. Genes Dev. 2002;16:3100–3112.10.1101/gad.1035402
- Mano Y, Nemoto K. The pathway of auxin biosynthesis in plants. J. Exp. Bot. 2012;63:2853–2872.10.1093/jxb/ers091
- Wang B, Chu J, Yu T, et al. Tryptophan-independent auxin biosynthesis contributes to early embryogenesis in Arabidopsis. Proc. Nat. Acad. Sci. USA. 2015;112:4821–4826.10.1073/pnas.1503998112
- Tao Y, Ferrer JL, Ljung K, et al. Rapid synthesis of auxin via a new tryptophan-dependent pathway is required for shade avoidance in plants. Cell. 2008;133:164–176.10.1016/j.cell.2008.01.049
- Yamada M, Greenham K, Prigge MJ, et al. The TRANSPORT INHIBITOR RESPONSE2 gene is required for auxin synthesis and diverse aspects of plant development. Plant Physiol. 2009;151:168–179.10.1104/pp.109.138859
- Phillips KA, Skirpan AL, Liu X, et al. Vanishing tassel2 encodes a grass-specific tryptophan aminotransferase required for vegetative and reproductive development in maize. Plant Cell. 2011;23:550–566.10.1105/tpc.110.075267
- Pacheco-Villalobos D, Sankar M, Ljung K, et al. Disturbed local auxin homeostasis enhances cellular anisotropy and reveals alternative wiring of auxin-ethylene crosstalk in Brachypodium distachyon seminal roots. PLoS Genet. 2013;9:e1003564.10.1371/journal.pgen.1003564
- Tivendale ND, Davidson SE, Davies NW, et al. Biosynthesis of the halogenated auxin, 4-chloroindole-3-acetic acid. Plant Physiol. 2012;159:1055–1063.10.1104/pp.112.198457
- Yoshikawa T, Ito M, Sumikura T, et al. The rice FISH BONE gene encodes a tryptophan aminotransferase, which affects pleiotropic auxin-related processes. Plant J. 2014;78:927–936.10.1111/tpj.12517
- Zhao Y, Christensen SK, Fankhauser C, et al. A role for flavin monooxygenase-like enzymes in auxin biosynthesis. Science. 2001;291:306–309.10.1126/science.291.5502.306
- Cheng Y, Dai X, Zhao Y. Auxin biosynthesis by the YUCCA flavin monooxygenases controls the formation of floral organs and vascular tissues in Arabidopsis. Genes Dev. 2006;20:1790–1799.10.1101/gad.1415106
- Chen Q, Dai X, De-Paoli H, et al. Auxin overproduction in shoots cannot rescue auxin deficiencies in Arabidopsis roots. Plant Cell Physiol. 2014;55:1072–1079.10.1093/pcp/pcu039
- Kriechbaumer V, Wang P, Hawes C, et al. Alternative splicing of the auxin biosynthesis gene YUCCA4 determines its subcellular compartmentation. Plant J. 2012;70:292–302.10.1111/tpj.2012.70.issue-2
- Dai X, Mashiguchi K, Chen Q, et al. The biochemical mechanism of auxin biosynthesis by an Arabidopsis YUCCA flavin-containing monooxygenase. J. Biol. Chem. 2013;288:1448–1457.10.1074/jbc.M112.424077
- Patten CL, Glick BR. Bacterial biosynthesis of indole-3-acetic acid. Can. J. Microbiol. 1996;42:207–220.10.1139/m96-032
- Hentrich M, Böttcher C, Düchting P, et al. The jasmonic acid signaling pathway is linked to auxin homeostasis through the modulation of YUCCA8 and YUCCA9 gene expression. Plant J. 2013;74:626–637.10.1111/tpj.2013.74.issue-4
- Sun J, Qi L, Li Y, et al. PIF4-mediated activation of YUCCA8 expression integrates temperature into the auxin pathway in regulating Arabidopsis hypocotyl growth. PLoS Genet. 2012;8:e1002594.10.1371/journal.pgen.1002594
- Yamamoto Y, Kamiya N, Morinaka Y, et al. Auxin biosynthesis by the YUCCA genes in rice. Plant Physiol. 2007;143:1362–1371.10.1104/pp.106.091561
- Fujino K, Matsuda Y, Ozawa K, et al. NARROW LEAF 7 controls leaf shape mediated by auxin in rice. Mol. Genet. Genomics. 2008;279:499–507.10.1007/s00438-008-0328-3
- Gallavotti A, Barazesh S, Malcomber S, et al. Sparse inflorescence1 encodes a monocot-specific YUCCA-like gene required for vegetative and reproductive development in maize. Proc. Nat. Acad. Sci. USA. 2008;105:15196–15201.10.1073/pnas.0805596105
- Bernardi J, Lanubile A, Li QB, et al. Impaired auxin biosynthesis in the defective endosperm18 mutant is due to mutational loss of expression in the ZmYuc1 gene encoding endosperm-specific YUCCA1 protein in maize. Plant Physiol. 2012;160:1318–1328.10.1104/pp.112.204743
- Tobena-Santamaria R, Bliek M, Ljung K, et al. FLOOZY of petunia is a flavin mono-oxygenase-like protein required for the specification of leaf and flower architecture. Genes Dev. 2002;16:753–763.10.1101/gad.219502
- Soeno K, Goda H, Ishii T, et al. Auxin biosynthesis inhibitors, identified by a genomics-based approach, provide insights into auxin biosynthesis. Plant Cell Physiol. 2010;51:524–536.10.1093/pcp/pcq032
- He W, Brumos J, Li H, et al. A small-molecule screen identifies l-kynurenine as a competitive inhibitor of TAA1/TAR activity in ethylene-directed auxin biosynthesis and root growth in Arabidopsis. Plant Cell. 2011;23:3944–3960.10.1105/tpc.111.089029
- Nishimura T, Hayashi K, Suzuki H, et al. Yucasin is a potent inhibitor of YUCCA, a key enzyme in auxin biosynthesis. Plant J. 2014;77:352–366.10.1111/tpj.2014.77.issue-3
- Stone SL, Braybrook SA, Paula SL, et al. Arabidopsis LEAFY COTYLEDON2 induces maturation traits and auxin activity: implications for somatic embryogenesis. Proc. Nat. Acad. Sci. USA. 2008;105:3151–3156.10.1073/pnas.0712364105
- Wójcikowska B, Jaskóła K, Gąsiorek P, et al. LEAFY COTYLEDON2 (LEC2) promotes embryogenic induction in somatic tissues of Arabidopsis, via YUCCA-mediated auxin biosynthesis. Planta. 2013;238:425–440.10.1007/s00425-013-1892-2
- Sohlberg JJ, Myrenås M, Kuusk S, et al. STY1 regulates auxin homeostasis and affects apical-basal patterning of the Arabidopsis gynoecium. Plant J. 2006;47:112–123.10.1111/tpj.2006.47.issue-1
- Eklund DM, Staldal V, Valsecchi I, et al. The Arabidopsis thaliana STYLISH1 protein acts as a transcriptional activator regulating auxin biosynthesis. Plant Cell. 2010;22:349–363.10.1105/tpc.108.064816
- Cui D, Zhao J, Jing Y, et al. The Arabidopsis IDD14, IDD15, and IDD16 cooperatively regulate lateral organ morphogenesis and gravitropism by promoting auxin biosynthesis and transport. PLoS Genet. 2013;9:e1003759.10.1371/journal.pgen.1003759
- Franklin KA, Lee SH, Patel D, et al. Phytochrome-interacting factor 4 (PIF4) regulates auxin biosynthesis at high temperature. Proc. Nat. Acad. Sci. USA. 2011;108:20231–20235.10.1073/pnas.1110682108
- Rawat R, Schwartz J, Jones MA, et al. REVEILLE1, a Myb-like transcription factor, integrates the circadian clock and auxin pathways. Proc. Nat. Acad. Sci. USA. 2009;106:16883–16888.10.1073/pnas.0813035106
- Pinon V, Prasad K, Grigg SP, et al. Local auxin biosynthesis regulation by PLETHORA transcription factors controls phyllotaxis in Arabidopsis. Proc. Nat. Acad. Sci. USA. 2013;110:1107–1112.10.1073/pnas.1213497110
- Li LC, Qin GJ, Tsuge T, et al. SPOROCYTELESS modulates YUCCA expression to regulate the development of lateral organs in Arabidopsis. New Phytol. 2008;179:751–764.10.1111/nph.2008.179.issue-3
- Zheng Z, Guo Y, Novák O, et al. Coordination of auxin and ethylene biosynthesis by the aminotransferase VAS1. Nat. Chem. Biol. 2013;9:244–246.10.1038/nchembio.1178
- Kong W, Li Y, Zhang M, et al. A novel Arabidopsis microRNA promotes IAA biosynthesis via the indole-3-acetaldoxime pathway by suppressing SUPERROOT1. Plant Cell Physiol. 2015;56:715–726.10.1093/pcp/pcu216
- Hull AK, Vij R, Celenza JL. Arabidopsis cytochrome P450s that catalyze the first step of tryptophan-dependent indole-3-acetic acid biosynthesis. Proc. Nat. Acad. Sci. USA. 2000;97:2379–2384.10.1073/pnas.040569997
- Mikkelsen MD, Hansen CH, Wittstock U, et al. Cytochrome P450 CYP79B2 from Arabidopsis catalyzes the conversion of tryptophan to indole-3-acetaldoxime, a precursor of indole glucosinolates and indole-3-acetic acid. J. Biol. Chem. 2000;275:33712–33717.10.1074/jbc.M001667200
- Nafisi M, Goregaoker S, Botanga CJ, et al. Arabidopsis cytochrome P450 monooxygenase 71A13 catalyzes the conversion of indole-3-acetaldoxime in camalexin synthesis. Plant Cell. 2007;19:2039–2052.10.1105/tpc.107.051383
- Bender J, Celenza JL. Indolic glucosinolates at the crossroads of tryptophan metabolism. Phytochem. Rev. 2009;25–37.10.1007/s11101-008-9111-7
- Bak S, Tax FE, Feldmann KA, et al. CYP83B1, a cytochrome P450 at the metabolic branch point in auxin and indole glucosinolate biosynthesis in Arabidopsis. Plant Cell. 2001;13:101–111.10.1105/tpc.13.1.101
- Grubb CD, Zipp BJ, Ludwig-Müller J, et al. Arabidopsis glucosyltransferase UGT74B1 functions in glucosinolate biosynthesis and auxin homeostasis. Plant J. 2004;40:893–908.10.1111/tpj.2004.40.issue-6
- Mikkelsen MD, Naur P, Halkier BA. Arabidopsis mutants in the C-S lyase of glucosinolate biosynthesis establish a critical role for indole-3-acetaldoxime in auxin homeostasis. Plant J. 2004;37:770–777.10.1111/tpj.2004.37.issue-5
- Bartel B, Fink GR. Differential regulation of an auxin-producing nitrilase gene family in Arabidopsis thaliana. Proc. Nat. Acad. Sci. USA. 1994;91:6649–6653.10.1073/pnas.91.14.6649
- Vorwerk S, Biernacki S, Hillebrand H, et al. Enzymatic characterization of the recombinant Arabidopsis thaliana nitrilase subfamily encoded by the NIT2/NIT1/NIT3-gene cluster. Planta. 2001;212:508–516.10.1007/s004250000420
- Park WJ, Kriechbaumer V, Moller A, et al. The nitrilase ZmNIT2 converts indole-3-acetonitrile to indole-3-acetic acid. Plant Physiol. 2003;133:794–802.10.1104/pp.103.026609
- Kriechbaumer V, Park WJ, Piotrowski M, et al. Maize nitrilases have a dual role in auxin homeostasis and beta-cyanoalanine hydrolysis. J. Exp. Bot. 2007;58:4225–4233.10.1093/jxb/erm279
- Su T, Xu J, Li Y, et al. Glutathione-indole-3-acetonitrile is required for camalexin biosynthesis in Arabidopsis thaliana. Plant Cell. 2011;23:364–380.10.1105/tpc.110.079145
- Wittstock U, Halkier BA. Cytochrome P450 CYP79A2 from Arabidopsis thaliana L. catalyzes the conversion of L-phenylalanine to phenylacetaldoxime in the biosynthesis of benzylglucosinolate. J. Biol. Chem. 2000;275:14659–14666.10.1074/jbc.275.19.14659
- Irmisch S, Zeltner P, Handrick V, et al. The maize cytochrome P450 CYP79A61 produces phenylacetaldoxime and indole-3-acetaldoxime in heterologous systems and might contribute to plant defense and auxin formation. BMC Plant Biol. 2015;15:128.10.1186/s12870-015-0526-1
- Pollmann S, Neu D, Weiler EW. Molecular cloning and characterization of an amidase from Arabidopsis thaliana capable of converting indole-3-acetamide into the plant growth hormone, indole-3-acetic acid. Phytochemistry. 2003;62:293–300.10.1016/S0031-9422(02)00563-0
- Romano CP, Robson PR, Smith H, et al. Transgene-mediated auxin overproduction in Arabidopsis: hypocotyl elongation phenotype and interactions with the hy6-1 hypocotyl elongation and axr1 auxin-resistant mutants. Plant Mol. Biol. 1995;27:1071–1083.10.1007/BF00020881
- Nemoto K, Hara M, Suzuki M, et al. The NtAMI1 gene functions in cell division of tobacco BY-2 cells in the presence of indole-3-acetamide. FEBS Lett. 2009;583:487–492.10.1016/j.febslet.2008.12.049
- Quittenden LJ, Davies NW, Smith JA, et al. Auxin biosynthesis in pea: characterization of the tryptamine pathway. Plant Physiol. 2009;151:1130–1138.10.1104/pp.109.141507
- Songstad DD, De Luca V, Brisson N, et al. High levels of tryptamine accumulation in transgenic tobacco expressing tryptophan decarboxylase. Plant Physiol. 1990;94:1410–1413.10.1104/pp.94.3.1410
- Seo M, Akaba S, Oritani T, et al. Higher activity of an aldehyde oxidase in the auxin-overproducing superroot1 mutant of Arabidopsis thaliana. Plant Physiol. 1998;116:687–693.10.1104/pp.116.2.687
- Sekimoto H, Seo M, Dohmae N, et al. Cloning and molecular characterization of plant aldehyde oxidase. J. Biol. Chem. 1997;272:15280–15285.10.1074/jbc.272.24.15280
- Wright AD, Sampson MB, Neuffer MG, et al. Indole-3-acetic acid biosynthesis in the mutant maize orange pericarp, a tryptophan auxotroph. Science. 1991;254:998–1000.10.1126/science.254.5034.998
- Normanly J, Cohen JD, Fink GR. Arabidopsis thaliana auxotrophs reveal a tryptophan-independent biosynthetic pathway for indole-3-acetic acid. Proc. Nat. Acad. Sci. USA. 1993;90:10355–10359.10.1073/pnas.90.21.10355
- Ouyang J, Shao X, Li J. Indole-3-glycerol phosphate, a branchpoint of indole-3-acetic acid biosynthesis from the tryptophan biosynthetic pathway in Arabidopsis thaliana. Plant J. 2000;24:327–333.10.1046/j.1365-313x.2000.00883.x
- Muller A, Weiler EW. Indolic constituents and indole-3-acetic acid biosynthesis in the wild-type and a tryptophan auxotroph mutant of Arabidopsis thaliana. Planta. 2000;211:855–863.
- Yu P, Lor P, Ludwig-Müller J, et al. Quantitative evaluation of IAA conjugate pools in Arabidopsis thaliana. Planta. 2015;241:539–548.10.1007/s00425-014-2206-z