Abstract
As molybdenum (Mo) is an indispensable metal for plant nitrogen metabolisms, accumulation of dissolved Mo into bacterial cells may connect to the development of bacterial fertilizers that promote plant growth. In order to enhance Mo bioaccumulation, nitrogen removal and light illumination were examined in anoxygenic photosynthetic bacteria (APB) because APB possess Mo nitrogenase whose synthesis is strictly regulated by ammonium ion concentration. In addition, an APB, Rhodopseudomonas palustris, transformed with a gene encoding Mo-responsive transcriptional regulator ModE was constructed. Mo content was most markedly enhanced by the removal of ammonium ion from medium and light illumination while their effects on other metal contents were limited. Increases in contents of trace metals including Mo by the genetic modification were observed. Thus, these results demonstrated an effective way to enrich Mo in the bacterial cells by the culture conditions and genetic modification.
Graphical abstract
Mo content was specifically enhanced in N-deprived photosynthetic bacterium harboring a gene coding Mo-binding protein (FL, full-length; CT, Mo-binding domain; EV, vector).
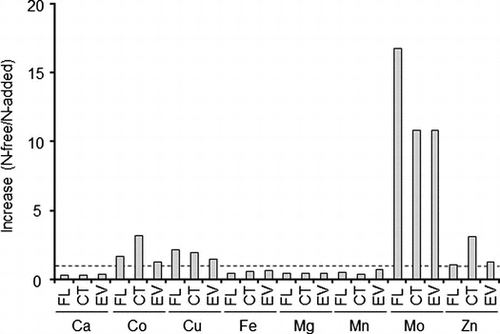
Recovery and reuse of agriculturally important limited resources are essential for sustainable food supply in order to keep up with world population growth. Among such resources, a trace element molybdenum (Mo) is contained in nearly all organisms, and it forms the catalytic center of a large variety of enzymes (molybdoenzymes). Loss of Mo-dependent enzyme activities through low internal Mo levels negatively impacts upon plant development, in particular, plant processes involving nitrogen metabolism.Citation1) Most prokaryotes also utilize Mo in molybdoenzymes such as dimethylsulfoxide reductase, sulfite oxidase,Citation2) nitrogenase, and nitrate reductase.Citation3,4)
Mo is bound in proteins to a pterin to form the molybdenum cofactor at the catalytic sites of many molybdoenzymes,Citation2) while Mo is contained as the Fe–Mo cofactor at the catalytic center in a mature form of Mo nitrogenase.Citation5) Escherichia coli periplasmic Mo-binding protein ModA encoded by modA and Mo-responsive transcriptional regulator ModE encoded by modE have binding capability to molybdate.Citation6) Mo is bound to ModA at the interdomain space of ModACitation7) and the C-terminal domain of ModECitation8) directly through hydrogen bonds between the oxyanion and specific amino acid residues. Therefore, when prokaryotes are cultured in Mo-added medium and synthesis of molybdoenzymes or Mo-binding proteins in the prokaryotes is induced under specific growth conditions, amount of Mo incorporated from the medium into cells and then captured by the proteins will be enhanced. Thus, a higher amount of Mo could be recovered from the medium by collecting the cultured prokaryotic cells. Dimethylsufoxide reductase family is the most widespread molybdoenzymes in prokaryotes.Citation2) However, considering an advantage that protein synthesis is derepressed not by any inducers but by specific culture conditions, Mo nitrogenase becomes a good candidate as a Mo-capturing protein synthesized in prokaryotic cells.
Nitrogenases catalyze the nitrogen fixation, which is a conversion process from nitrogen gas to ammonia. Nitrogen fixation has been found in many bacteria such as root nodule bacteria,Citation9) cyanobacteria, and anoxygenic photosynthetic bacteria (APB).Citation10) Therefore, one possibility is that such nitrogen-fixing bacteria possess higher accumulating capacity of Mo dissolved in aquatic environments than non-nitrogen-fixing species. Mo-enriched bacterial cells may have potential to be used as a biofertilizer because Mo could promote plant growth and primary production.Citation11)
APB are superior to other nitrogen-fixing bacteria in their versatility of growth modes, and can utilize light energy under phototrophic conditions.Citation12) Accumulation of Mo from aquatic environments into living cells driven by sunlight could be achieved by culturing APB. In addition, large-scale cultivation techniques of APB have been established.Citation13–15) In general, nitrogenase synthesis is repressed by the presence of ammonium ion and oxygen gas. Therefore, in case of APB cultures, de novo synthesis of the nitrogenase structural proteins can be induced by exhaustion or removal of ammonium ion and oxygen gas from environments.Citation16,17)
In this study, methods that enhance accumulation of Mo in APB were evaluated by comparing metal contents of cells cultured with and without ammonium ion, oxygen, and light illumination. Effect of introduction of modE into APB on metal contents was also examined. Analyses of gene expression and metal composition firstly demonstrated that cultivation of the genetically modified APB in N-free medium under phototrophic conditions effectively enhanced the cellular Mo content through expression of nitrogenase and ModE genes.
Materials and methods
Bacterial strains and culture media
APB including Rhodopseudomonas palustris CGA009,Citation18) its genetically modified strains, and Rhodovulum sulfidophilum W-1SCitation19) were pre-cultivated at 32 °C under photoheterotrophic conditions using incandescent lamp as a light source in 1.3-L rectangular glass bottle containing 300 mL of modified Okamoto medium (MOM),Citation20) in which yeast extract was removed. When Rvu. sulfidophilum was cultured, 30 g L−1 NaCl was added, instead of 0.2 g L−1. The pre-culture was divided into two portions and exponentially growing cells were collected by centrifugation at 4 °C. The cells were re-suspended into MOM not containing yeast extract but containing ammonium chloride (N-added) or MOM not containing both yeast extract and ammonium chloride (N-free) so that OD600 of cell suspension became around 0.6. The cell suspension was transferred to 170-mL N-added or N-free medium in 175-mL rectangular glass bottle. When the cell suspension was transferred to N-free medium, the bottle was sealed with a rubber cap with double-seal stopper to cultivate cells under anoxic conditions. In case of culturing in N-added medium, a silicon cap was used. Mo concentration in the N-added and N-free media was 100-fold increased (10 μM) and 4 g L−1 disodium succinate was added as a sole carbon source. The cells were cultured for 72 h at 32 °C under photoheterotrophic conditions, collected by centrifugation at 4 °C, washed with ultrapurified water, and stored at −25 °C after removal of supernatant.
Construction of genetically modified strains of APB
PCR-amplified fragments and plasmids were purified with MinElute PCR purification kit and Plasmid mini kit, respectively (both from Qiagen, Tokyo, Japan). Genes encoding full-length ModE (FLmodE) or its Mo-binding domain at the C-terminus (CTmodE)Citation21) were amplified with polymerase chain reaction (PCR) using total DNA of E. coli K12 as a template DNA. Forward primers were AATCTAGAAGGAGGTAGGTACCCATGCAGGCCGAAATCCTTCT for FLmodE and AATCTAGAAGGAGGTAGGTACCCATGCCGCTGAACAGCCTGCTGGC for CTmodE, and a reverse primer for FLmodE and CTmodE was AATCTAGATTAGCACAGCGTGGCGATAA, where XbaI sites were italicized. Plasmids were constructed in E. coli DH5α, based on a vector pMG103ΩParsarsRcrtIcat,Citation20) which is a derivative of Rps. palustris pMG103.Citation22) A vector fragment containing a phytoene desaturase gene (crtI) and a chloramphenicol acetyltransferase gene (cat) was amplified with the inverse PCR using a primer set of 5′-CATCTAGATTAAGGAGGTACCCATGCTCG and 5′-AATCTAGAGTCGACCTGCAGAAAAAAAGCG, where XbaI sites were italicized, and pMG103ΩParsarsRcrtIcat as a template DNA to form an empty vector (EV) pMG103ΩcrtIcat. FLmodE, CTmodE, and pMG103ΩcrtIcat were digested with XbaI, and FLmodE and CTmodE were separately ligated with an XbaI site of pMG103ΩcrtIcat to form pMG103ΩFLmodEcrtIcat and pMG103ΩCTmodEcrtIcat, respectively. Direction of FLmodE or CTmodE in the vector was checked by PCR and clones with the appropriate direction were selected. DNA sequences of FLmodE and CTmodE in pMG103ΩFLmodEcrtIcat and pMG103ΩCTmodEcrtIcat were confirmed. pMG103ΩFLmodEcrtIcat, pMG103ΩCTmodEcrtIcat, and pMG103ΩcrtIcat were separately introduced into Rps. palustris No. 711Citation23) by electroporation, as described previouslyCitation20) to obtain genetically modified strains, FL, CT, and EV. Reddish colonies obtained as the result of complementation of the crtI deletion in No. 711 with a crtI gene of pMG103ΩcrtIcat were inoculated to liquid media as transformants.
Analysis of gene expression
For the extraction of RNA from recombinant Rps. palustris, 0.5 mL of culture at late log phase was mixed with 1.0 mL of RNAprotect bacteria reagent (Qiagen, Tokyo). Cells were collected by centrifugation at 4 °C for 10 min and immediately stored at −80 °C. Extraction of RNA was started by resuspending the frozen cells with 100-μL TE buffer (10 mM Tris–HCl, 1.0 mM ethylene diamine tetraacetic acid (EDTA) sodium salt, pH 8.0) containing 1.0 mg mL−1 lysozyme. The cell suspension was incubated at room temperature for 5 min. Then, 350-μL buffer RLT containing 2-mercaptoethanol was added to the cell suspension, followed by the standard protocol of RNeasy Mini Kit (Qiagen, Tokyo). Expression of nitrogenase- and ModE-coding genes was quantified by the real-time reverse transcription PCR (RT-PCR) assay using ReverTra Ace qPCR RT kit and KOD SYBR qPCR Mix (both from Toyobo, Osaka, Japan). cDNA corresponding to FLmodE and CTmodE was quantified using a primer set comprising 5′-GCTGGATGAAGGCAAAGAAG and 5′-TCGGGTAGCGCCATTAATAC, which targets to a genetic region coding an amino acid sequence within the Mo-binding domain. cDNA corresponding to a nitrogenase gene was quantified using a primer set comprising 5′-TATGGGAAAGGCGGGATCGG and 5′-TCGAGTCCGCCTTGGGATCG, which targets a genetic region coding a part of nifH, a structural gene of nitrogenase reductase. Expression intensity was normalized by molar ratio to 16S rRNA gene quantified by the RT-PCR targeted by a primer set comprising 5′-CCTACGGGAGGCAGCAG and 5′-GTATTACCGCGGCTGCTG. Threshold PCR cycle number (Ct) was determined with ABI 7500 real-time PCR system (Applied Biosystems, Foster City, CA, USA) and cDNA was relatively quantified using an equation of 1/(2Ct).
Analysis of metal composition
Cells collected after cultivation and stored at −25 °C were lyophilized overnight, ground in a mortar with pestle, weighted, and transferred to crucible. The crucibles containing powdered cells were heated in an electric muffle furnace (FUW 220PA, Advantec, Tokyo, Japan) using a temperature program of a gradient of room temperature to 150 °C per 30 min, 150 °C for 1 h, a gradient of 150–550 °C per 3 h, and 550 °C for 5 h in order to reduce cell mass. After cooling down to room temperature, 2 mL of nitric acid (65% wt/v) was added to the cell debris and evaporated by heating at 250 °C in a draft chamber. Crucibles containing the cell debris were again heated in a muffle furnace with the same heating program. Then, 2 mL of nitric acid (65% wt/v) was again added to cell debris and the mixture was heated at 250 °C in a draft chamber until the nitric acid was removed from the crucibles. Ashes in the crucibles were dissolved with 2 mL of 6 N HCl, and the metal solution was heated at 150 °C. When whitish HCl vapor was emerged, heating was stopped. After cooling down to room temperature, 25 mL of ultrapure water was added to the HCl in crucible, followed by filtration through an Advantec 5C filter paper. The concentrations of Ca, Fe, Mg, Mn, and Zn extracted from the lyophilized cells were measured by inductively coupled plasma atomic emission spectrometry (ICP-AES) (ICPS-7500, Shimadzu, Kyoto, Japan). Co and Cu, whose concentrations in the extracts were less than detection limits of ICP-AES, were quantified by electrothermal atomic absorption spectrometry (ET-AAS) (Z-5010, Hitachi high-technologies, Tokyo, Japan). Mo was measured by either ICP-AES or ET-AAS.
Evaluation of growth rate
Cell growth was monitored after inoculation of 2 mL pre-cultures to 100 mL of N-added medium in 170-mL rectangular glass bottle under same conditions as applied to the pre-culture for Mo bioaccumulation. Optical density (OD) was measured every 10 min with OD-Monitor (Taitec, Koshigaya, Saitama, Japan). An incandescent lamp was cut off for 1 min during the measurement of OD. The specific growth rate (μ) was calculated by Equation (Equation1(1) ).
(1)
Results
Metal contents in wild-type strains cultivated in N-free and N-added media
The wild-type APB were cultivated in N-free and N-added media, and Ca, Fe, Mg, and Mo contents in the cells after cultivation were determined. Ca, Mg, and Fe were abundantly contained in a culture medium as divalent cations. Light-driven hydrogen gas production was observed during cultivation in N-free medium but not in N-added medium (data not shown), suggesting that the cells cultivated in the N-free medium had nitrogenase activity. Cellular contents of Ca and Mg were higher in N-added medium than in N-free medium in both Rps. palustris and Rvu. sulfidophilum (Table ). This suggested that the cells growing with sufficient N supply accumulated the divalent cations with higher efficiency, compared to the growth-restricted cells by the removal of N source. On the other hand, cellular contents of Mo were remarkably increased in N-free medium in both bacterial species. The differences in Fe content were less significant than in Ca, Mg, and Mo contents although Fe is contained in the catalytic center of Mo nitrogenase. The result indicates that cultivation of the APB in N-free medium under phototrophic conditions specifically increases the cellular content of Mo.
Table 1. Metal contents of wild-type cells cultivated in N-free and N-added media.
Gene expression and growth rates of recombinant Rps. palustris strains
Rps. palustris cells transformed with either FLmodE or CTmodE were cultured with light illumination. In order to investigate whether the introduced genes were expressed or not, their transcriptional levels were quantified with RT-PCR (Fig. ). Transcriptional level for a nitrogenase gene (nifH) was also determined with RT-PCR. In all the recombinant strains, nifH was transcribed only in the absence of N sources. Amounts of modE transcript in the strains FL and CT were significantly increased, compared to that in the strain EV, in which only a background level of modE transcript was detected, regardless of the culture conditions.
Fig. 1. Expression of genes encoding nitrogenase (A) and ModE (B) in the recombinant Rps. palustris strains cultured in N-added (open columns) and N-free (closed columns) media.
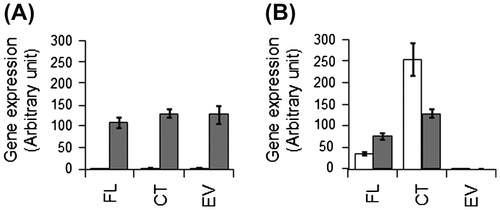
Heterologous expression of the transcriptional regulator genes may inhibit cell growth of the recombinant Rps. palustris strains and reduce the final biomasses, in which Mo is biologically concentrated from aquatic environments. Therefore, growth under photoheterotrophic conditions was evaluated in the recombinant strains (Fig. ). Growth rates at the log phase were almost same among the three strains, indicating that transformation with FLmodE and CTmodE does not repress photoheterotrophic growth of Rps. palustris. The specific growth rates calculated with Equation Equation1(1) were 0.38 h−1 for strain CT, 0.45 h−1 for stain FL, and 0.41 h−1 for strain EV.
Metal contents in the recombinant strains cultivated in N-free and N-added media
Effect of the genetic modification on Ca, Mg, Fe, and Mo contents was investigated in the absence and presence of N source. Marked increases by cultivation in N-free medium were observed only in Mo contents (Table ). The Mo contents also increased in the recombinant strains FL and CT when compared to the Mo contents in the strain EV. However, the increases of Mo content by the genetic modification were not so much as observed in the increases of Mo content achieved by cultivation in N-free medium. Both cultivation in N-free medium and the genetic modification resulted in decreases of Ca, Fe, and Mg contents. As observed in Ca and Mg contents of the Rps. palustris wild-type strain, contents of Ca, Fe, and Mg were abundantly found in all the recombinant strains grown in N-added medium.
Table 2. Metal contents of recombinant cells cultivated in N-free and N-added media.
Cultivation of the recombinant strains with light illumination was performed in order to determine the trace metal contents. In this comparison, Mo contents in all the strains were markedly increased by cultivation in N-free medium, compared to the increases in the other trace metal contents (Table ). Only Mn contents decreased in all the strains cultured in N-free medium. The genetic modification also resulted in increases of the trace metal contents except Mn in the cells cultured in N-free medium. The effect of genetic modification on the increases in trace metal contents was limited in the cells cultured in N-added medium.
Table 3. Trace metal contents of recombinant cells cultivated in N-free and N-added media.
Ratios of metal content in cells cultured in N-free medium to that in N-added medium were calculated (Fig. (A)). Even though up to threefold increases in Co, Cu, and Zn contents were observed, N-deprived cells of all the recombinant strains specifically accumulated Mo. The highest increase in the ratio of Mo was found in strain FL mainly because, after cultivation in N-added medium, the Mo content of FL was lower than that of CT. Ratios of metal content in FL or CT to that in EV were also calculated from the data obtained with N-free (Fig. (B)) and N-added (Fig. (C)) media. Introduction of FLmodE or CTmodE enhanced the contents of Cu and Mo, regardless of differences in the genes introduced and the media used. In case of Co, Mn, and Zn, whether or not the ratio increases is dependent on the genes introduced and the media used. The result indicates that the effect of introduction of FLmodE or CTmodE on the increase in metal content is specific to the trace metals, whereas the increase in the ratio by cultivation in N-free medium is markedly observed in Mo content.
Fig. 3. Ratios of metal content of cells cultured in N-free medium to that in N-added medium (A) and ratios of metal content of strain FL or CT to that of strain EV cultured in N-free medium (B) and N-added medium (C).
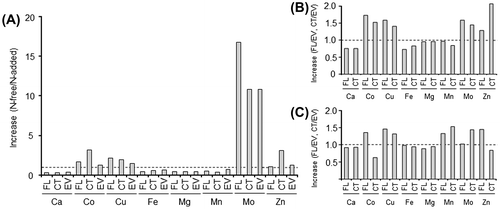
Metal contents in a recombinant strain cultivated with and without light illumination
The recombinant strain CT exhibited higher Mo content than that in the strain EV both in N-free and N-added media and higher expression level of CTmodE than those in the strains FL and EV. Therefore, the strain CT was cultured under N-free and anoxic conditions with light illumination or dark in order to investigate whether Mo bioaccumulation is enhanced by light or not (Table ). Cells cultured under light illumination accumulated about 1.5–2 times higher contents of Fe, Mg, Mn, and Mo compared to those of cells cultured under dark.
Table 4. Metal contents of recombinant strain CT cultivated under N-free and anoxic conditions.
Discussion
This study firstly demonstrated that Mo bioaccumulation in an APB Rps. palustris is increased by specific culture conditions as well as by introduction of a gene encoding Mo-binding protein. The Mo contents of the strains FL and CT grown in N-added medium were much lower than the Mo content of the strain EV cultivated in N-free medium. The result indicates that removal or exhaustion of ammonium ion caused more significant increase than the genetic modification did. Removal of ammonium ion from the bacterial cultures might cause de novo synthesis of intracellular Mo-binding proteins such as Mo nitrogenase, resulting in the marked increase of cellular capability of Mo retention. This physiological change was supported by the results that nifH was derepressed and hydrogen gas was produced only in N-free medium with light illumination. In addition to the de novo synthesis of molybdoenzymes, the marked and specific increase in the N-deprived cells may be explained by capacity change in Mo transportation through cellular membrane and difference in the manner of Mo binding to protein between molybdoenzymes and ModE (interaction with protein through incorporation into cofactor vs. direct interaction with protein through hydrogen bonds). The increase in Mo contents in N-free medium under phototrophic conditions was also seen in another species of APB Rvu. sulfidophilum.
As shown in the experiments using the recombinant Rps. palustris strains, although cultivation under the N-free and anaerobic conditions with light illumination was effective in order to increase Mo content, the N-free and anaerobic conditions more strongly affected it than light illumination did. Several papers have reported the metabolisms of APB, in which molybdoenzymes might participate. Rhodopseudomonas sp. isolated from activated sludge exhibited nitrite oxidation under phototrophic conditions.Citation24) However, only weak activity of nitrite oxidation could be found. Dimethyl sulfoxide reductase in Rhodobacter sphaeroides has been shown to take part in anaerobic respiration using dimethyl sulfoxide in the absence of light.Citation25) Dimethyl sulfide dehydrogenase in Rvu. sulfidophilum is a molybdoenzyme needed for the anaerobic respiration using dimethyl sulfide during photolithotrophic growth,Citation26) but the gene encoding the enzyme is induced by dimethyl sulfide.Citation27) Xanthine dehydrogenase gene in Rhodobacter capslatus is not regulated under the N regulatory network but induced by xanthine.Citation28) On the other hand, it has been suggested in Rps. palustris that the 32 genes in the nif gene cluster were induced in Mo nitrogenase-expressing wild-type strains phototrophically grown under nitrogen-fixing conditions.Citation29) Thus, the results in this study and the features of molybdoenzymes reported in bibliography suggest main contribution of Mo nitrogenase synthesis to the specific increase of Mo content in the APB cultured under N-free and anoxic conditions with light illumination.
Though APB are versatile in growth mode, their ATP synthesis under anoxic conditions is dependent partly on absorption and utilization of light energy. The result showing enhancement of Fe, Mg, Mn, and Mo bioaccumulation with light illumination suggests that processes needed for bioaccumulation of these metals might require ATP whose synthesis is highly dependent on light under anoxic conditions. Another possibility is that physiological roles of these specific metals in light-dependent processes may cause the increased cellular contents under light illumination.
Obvious advantage in Mo accumulation could not be seen between introduction of FLmodE and CTmodE in N-free medium, though strain CT repeatedly resulted in the increase of Mo content in N-added medium compared to that in strain EV. The genetic modification affected not only Mo content but also the other trace metal contents. Considering that ModE has the metal specificity to molybdate and tungstate,Citation30 secondary effects of ModE or the Mo-binding domain of ModE as a chelator of the metallic oxyanions or a transcriptional regulator may change the trace metal contents, which are two to four orders of magnitude lower than Ca, Fe, and Mg contents and, therefore, must be easily affected by production of the recombinant metalloprotein.
As bacterial growth is severely restricted by N depletion, it is important for Mo bioaccumulation to obtain a sufficient number of cells before suspending the cells with N-free medium containing dissolved Mo. The genetic transformation of Rps. palustris with FLmodE or CTmodE did not impair the specific growth rate in N-added medium, indicating that a two-stage cultivation composed of the stages for growth of the recombinant bacteria in N-added medium and Mo accumulation in N-free medium would maximize the efficiency of Mo accumulation under phototrophic conditions. The application of APB as biofertilizers has been studied in various crops, which include rice, leguminous plants, tomato, citrus, oranges, and persimmon.Citation31) Rps. palustris is also known as a producer of 5-aminolevulinic acid,Citation32) which has been reported to promote growth of rice seedling, growth of horseradish shoot primordia, and CO2 fixation rate of pothos.Citation33) A previous study revealed that foliar application of Mo increases the nitrogenase and nitrate reductase activities with a consequent increase in total shoot N in common bean.Citation34) Therefore, enrichment of Mo in APB by the bioaccumulation strategy performed in this study may improve a value of the bacterial fertilizer to promote N assimilation in leguminous plants.
Conclusions
In this study, enhancement of light-driven Mo bioaccumulation in genetically modified Rps. palustris under specific culture conditions was demonstrated. The enhancement under the specific culture conditions was highly specific to Mo content whereas the genetic modification to introduce a gene encoding Mo-binding protein affected contents of trace metals including Mo. The genetic modification did not inhibit cell growth. Therefore, it was suggested that the two-stage culture of recombinant Rps. palustris, composed of a growth stage in N-added medium and a subsequent Mo bioaccumulation stage in N-free medium, might be an effective way to enrich Mo in the biomass.
Author contributions
Taki Naito, Sachuronggui, and Masayoshi Ueki performed all sample preparation, data acquisition, and data analyses. Isamu Maeda contributed to conception of the study, experimental design, manuscript writing, and revision of manuscript.
Disclosure statement
No potential conflict of interest was reported by the authors.
Funding
This work was supported by a Grant-in-Aid for Scientific Research (B) from the Japan Society for the Promotion of Science [grant number JSPS 23360362] to Isamu Maeda.
References
- Kaiser BN, Gridley KL, Ngaire Brady J, et al. The role of molybdenum in agricultural plant production. Ann. Bot. 2005;96:745–754.10.1093/aob/mci226
- Zhang Y, Gladyshev VN. Molybdoproteomes and evolution of molybdenum utilization. J. Mol. Biol. 2008;379:881–899.10.1016/j.jmb.2008.03.051
- Beevers L, Hageman RH. Nitrate reduction in higher plants. Annu. Rev. Plant Physiol. 1969;20:495–522.10.1146/annurev.pp.20.060169.002431
- Schwarz G, Mendel RR, Ribbe MW. Molybdenum cofactors, enzymes and pathways. Nature. 2009;460:839–847.10.1038/nature08302
- Shah VK, Brill WJ. Isolation of an iron-molybdenum cofactor from nitrogenase. Proc. Natl. Acad. Sci. USA. 1977;74:3249–3253.10.1073/pnas.74.8.3249
- Grunden AM, Ray RM, Rosentel JK, et al. Repression of the Escherichia coli modABCD (molybdate transport) operon by ModE. J. Bacteriol. 1996;178:735–744.
- Aryal BP, Brugarolas P, He C. Binding of ReO4(-) with an engineered MoO4(2-)-binding protein: towards a new approach in radiopharmaceutical applications. J. Biol. Inorg. Chem. 2012;17:97–106.10.1007/s00775-011-0833-4
- Hall DR, Gourley DG, Leonard GA, et al. The high-resolution crystal structure of the molybdate-dependent transcriptional regulator (ModE) from Escherichia coli: a novel combination of domain folds. EMBO J. 1999;18:1435–1446.10.1093/emboj/18.6.1435
- Hennecke H. Nitrogen fixation genes involved in the Bradyrhizobium japonicum-soybean symbiosis. FEBS Lett. 1990;268:422–426.10.1016/0014-5793(90)81297-2
- Pinckney JL, Paerl HW. Anoxygenic photosynthesis and nitrogen fixation by a microbial mat community in a bahamian hypersaline lagoon. Appl. Environ. Microbiol. 1997;63:420–426.
- Barron AR, Wurzburger N, Bellenger JP, et al. Molybdenum limitation of asymbiotic nitrogen fixation in tropical forest soils. Nat. Geosci. 2009;2:42–45.10.1038/ngeo366
- Carius L, Carius AB, McIntosh M, et al. Quorum sensing influences growth and photosynthetic membrane production in high-cell-density cultivations of Rhodospirillum rubrum. BMC Microbiol. 2013;13:189.10.1186/1471-2180-13-189
- Adessi A, Torzillo G, Baccetti E, et al. Sustained outdoor H2 production with Rhodopseudomonas palustris cultures in a 50 L tubular photobioreactor. Int. J. Hydrogen Energy. 2012;37:8840–8849.10.1016/j.ijhydene.2012.01.081
- Carlozzi P, Sacchi A. Biomass production and studies on Rhodopseudomonas palustris grown in an outdoor, temperature controlled, underwater tubular photobioreactor. J. Biotechnol. 2001;88:239–249.10.1016/S0168-1656(01)00280-2
- Maeda I, Mizoguchi T, Miura Y, et al. Influence of sulfate-reducing bacteria on outdoor hydrogen production by photosynthetic bacterium with seawater. Curr. Microbiol. 2000;40:210–213.10.1007/s002849910042
- Basak N, Das D. The prospect of purple non-sulfur (PNS) photosynthetic bacteria for hydrogen production: the present state of the art. World J. Microbiol. Biotechnol. 2007;23:31–42.10.1007/s11274-006-9190-9
- Lehman LJ, Roberts GP. Identification of an alternative nitrogenase system in Rhodospirillum rubrum. J. Bacteriol. 1991;173:5705–5711.
- Larimer FW, Chain P, Hauser L, et al. Complete genome sequence of the metabolically versatile photosynthetic bacterium Rhodopseudomonas palustris. Nat. Biotechnol. 2004;22:55–61.10.1038/nbt923
- Maeda I, Miyasaka H, Umeda F, et al. Maximization of hydrogen production ability in high-density suspension of Rhodovulum sulfidophilum cells using intracellular poly(3-hydroxybutyrate) as sole substrate. Biotechnol. Bioeng. 2003;81:474–481.10.1002/(ISSN)1097-0290
- Yoshida K, Inoue K, Takahashi Y, et al. Novel carotenoid-based biosensor for simple visual detection of arsenite: characterization and preliminary evaluation for environmental application. Appl. Environ. Microbiol. 2008;74:6730–6738.10.1128/AEM.00498-08
- Nishitani T, Shimada M, Kuroda K, et al. Molecular design of yeast cell surface for adsorption and recovery of molybdenum, one of rare metals. Appl. Microbiol. Biotechnol. 2010;86:641–648.10.1007/s00253-009-2304-1
- Inui M, Roh JH, Zahn K, et al. Sequence analysis of the cryptic plasmid pMG101 from Rhodopseudomonas palustris and construction of stable cloning vectors. Appl. Environ. Microbiol. 2000;66:54–63.10.1128/AEM.66.1.54-63.2000
- Yoshida K, Yoshioka D, Inoue K, et al. Evaluation of colors in green mutants isolated from purple bacteria as a host for colorimetric whole-cell biosensors. Appl. Microbiol. Biotechnol. 2007;76:1043–1050.10.1007/s00253-007-1079-5
- Schott J, Griffin BM, Schink B. Anaerobic phototrophic nitrite oxidation by Thiocapsa sp. strain KS1 and Rhodopseudomonas sp. strain LQ17. Microbiology. 2010;156:2428–2437.10.1099/mic.0.036004-0
- Mouncey NJ, Choudhary M, Kaplan S. Characterization of genes encoding dimethyl sulfoxide reductase of Rhodobacter sphaeroides 2.4.1T: an essential metabolic gene function encoded on chromosome II. J. Bacteriol. 1997;179:7617–7624.
- McDevitt CA, Hugenholtz P, Hanson GR, et al. Molecular analysis of dimethyl sulphide dehydrogenase from Rhodovulum sulfidophilum: its place in the dimethyl sulphoxide reductase family of microbial molybdopterin-containing enzymes. Mol. Microbiol. 2002;44:1575–1587.10.1046/j.1365-2958.2002.02978.x
- Maeda I, Yamashiro H, Yoshioka D, et al. Colorimetric dimethyl sulfide sensor using Rhodovulum sulfidophilum cells based on intrinsic pigment conversion by CrtA. Appl. Microbiol. Biotechnol. 2006;70:397–402.10.1007/s00253-005-0117-4
- Leimkühler S, Kern M, Solomon PS, et al. Xanthine dehydrogenase from the phototrophic purple bacterium Rhodobacter capsulatus is more similar to its eukaryotic counterparts than to prokaryotic molybdenum enzymes. Mol. Microbiol. 1998;27:853–869.10.1046/j.1365-2958.1998.00733.x
- Oda Y, Samanta SK, Rey FE, et al. Functional genomic analysis of three nitrogenase isozymes in the photosynthetic bacterium Rhodopseudomonas palustris. J. Bacteriol. 2005;187:7784–7794.10.1128/JB.187.22.7784-7794.2005
- Kuroda K, Nishitani T, Ueda M. Specific adsorption of tungstate by cell surface display of the newly designed ModE mutant. Appl. Microbiol. Biotechnol. 2012;96:153–159.10.1007/s00253-012-4069-1
- Sasikaka C, Ramana CV. Biotechnological potentials of anoxygenic phototrophic bacteria. II. Biopolyesters, biopesticide, biofuel, and biofertilizer. In: Neidleman SL, editor. Advances in applied microbiology 41. San Diego (CA): Academic Press; 1995. p. 227–271.
- Saikeur A, Choorit W, Prasertsan P, et al. Influence of precursors and inhibitor on the production of extracellular 5-aminolevulinic acid and biomass by Rhodopseudomonas palustris KG31. Biosci. Biotechnol. Biochem. 2009;73:987–992.10.1271/bbb.80682
- Hotta Y, Tanaka T, Takaoka H, et al. New physiological effects of 5-aminolevulinic acid in plants: the increase of photosynthesis, chlorophyll content, and plant growth. Biosci. Biotechnol. Biochem. 1997;61:2025–2028.10.1271/bbb.61.2025
- Vieira RF, Cardoso EJBN, Vieira C, et al. Foliar application of molybdenum in common beans. I. Nitrogenase and reductase activities in a soil of high fertility. J. Plant Nutr. 1998;21:169–180.10.1080/01904169809365391