Abstract
Mycotoxin contamination of crops is a serious problem throughout the world because of its impact on human and animal health as well as economy. Inhibitors of mycotoxin production are useful not only for developing effective methods to prevent mycotoxin contamination, but also for investigating the molecular mechanisms of secondary metabolite production by fungi. We have been searching for mycotoxin production inhibitors among natural products and investigating their modes of action. In this article, we review aflatoxin and trichothecene production inhibitors, including our works on blasticidin S, methyl syringate, cyclo(l-Ala-l-Pro), respiration inhibitors, and precocene II.
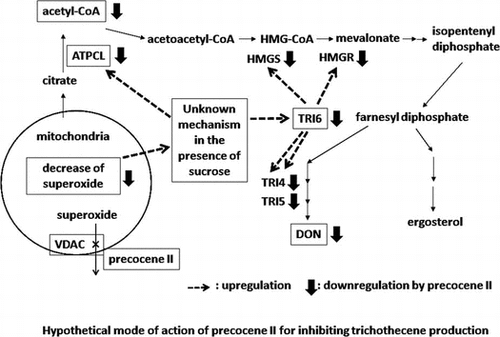
Fungi produce a variety of secondary metabolites, hundreds of which are toxic to humans and animals. Toxic fungal secondary metabolites with low-molecular weight are called mycotoxins. Mycotoxin-producing fungi infect and produce mycotoxins in crops before and/or after harvest. Mycotoxins produced in food and feed are stable (not degraded) during storage, processing, or cooking at temperatures typically used for food. Mycotoxin contamination of food and feed can lead to human and animal diseases (mycotoxicoses) and economic loss due to food waste.Citation1,2) However, despite the worldwide prevalence of mycotoxin contamination, there are few effective methods to protect crops from contamination by mycotoxins, especially aflatoxin.
Compounds that inhibit mycotoxin production without inhibiting fungal growth may be useful for mycotoxin control without incurring a rapid spread of resistant strains. Highly selective inhibitors of mycotoxin production are also useful as probes to investigate the basic regulatory mechanisms of secondary metabolites production in fungi. Understanding these regulatory mechanisms is crucial for determining the optimal target of methods to control mycotoxin contamination.
As mycotoxin-producing fungi infect plants, effects of many plant constituents on growth and mycotoxin production of mycotoxin-producing fungi have been tested. Such information of plant constituents on mycotoxin-producing fungi may be important for understanding and developing crop resistance to mycotoxin contamination. On the other hand, we have been using a bioassay-guided screening method to obtain mycotoxin production inhibitors that do not affect fungal growth and investigating their modes of action.
We have been searching inhibitors of aflatoxin and trichothecene production among natural products, such as microbial metabolites, essential oils, and chemical library. The use of natural products is desirable in agriculture as such products are easily degraded in the natural environment. In this article, we review works on aflatoxin and trichothecene production inhibitors including inhibitors obtained by our screenings.
Aflatoxin production inhibitors
Aflatoxin contamination of crops represents the most serious mycotoxin problem worldwide.Citation3,4) Natural aflatoxins (aflatoxins B1 (1), B2 (2), G1 (3), G2 (4), M1 (5), and M2 (6)) are produced by Aspergillus species, mainly Aspergillus flavus that produces aflatoxins B1 and B2 as main components and Aspergillus parasiticus that produces aflatoxins B1, B2, G1, and G2 as main components. Aflatoxins M1 and M2 can be biosynthesized by both A. flavus and A. parasiticus as minor components. Aflatoxins M1 and M2 are also produced as hydroxylated metabolites of aflatoxins B1 and B2, respectively, in animals. Contamination of cow’s milk by aflatoxins M1 and M2 results as a consequence of feeding cows with aflatoxin-contaminated feeds. Aflatoxin B1 has the strongest carcinogenicity among not only aflatoxins, but also all known natural products (see Fig. ).
Fig. 1. Structures of aflatoxins and aflatoxin production inhibitors from plant constituents.
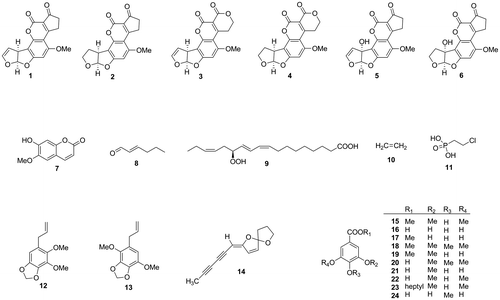
Aflatoxin contamination of crops, such as maize, peanut, pistachio nut, and rice, is most common in tropical and subtropical areas, resulting in very severe economic loss.Citation4) It is estimated that five billion people worldwide are exposed to uncontrolled aflatoxin in the dietCitation3) and many liver cancer cases are caused by aflatoxin intake.Citation5) Thus, it is essential to prevent and reduce aflatoxin contamination of crops. Environmental and storage conditions as well as insect control are important factors for crop contamination by any mycotoxin. However, few practical methods are available for aflatoxin control at present. Antifugal agents, which can kill mycotoxin-producing fungi, are generally used for mycotoxin control such as the case of deoxynivalenol contamination as described later. But, there is no fungicide effective against aflatoxin producers in fields.Citation6)
One novel approach for reducing aflatoxin contamination is to use spores of non-aflatoxigenic strains of A. flavus,Citation7) which may compete with natural aflatoxigenic strains to reduce aflatoxin contamination. In this process, the effects of non-aflatoxigenic strains on nature, such as gene transfer between non-aflatoxigenic and aflatoxigenic strains by mating, should be monitored.Citation8) Many micro-organisms are effective for aflatoxin control under laboratory conditionsCitation9); however, except for the non-aflatoxigenic strains, these micro-organisms have not been used practically.
A variety of aflatoxin production inhibitors have been known. Their sources are roughly classified into two groups, natural products and synthetic compounds such as pesticides. Holmes et al. have published an excellent review article on aflatoxin production inhibitors in 2008.Citation10) We summarize recent works on aflatoxin production inhibitors including our works in the following.
Aflatoxin production inhibitors from natural products
Plant constituents
In the review by Holmes et al.Citation10), aflatoxin production inhibitors from plant constituents were classified into a variety of groups such as alkaloids (caffeine, piperine, piperlongumine, pipernonaline, and piperoctadecalidine); phenylpropanoids [coumarins and furanocumarins (bergapten, p-coumaric acid, 5,7-dihydroxychromone, khellin, psoralene, visnagin, and xanthotoxin), flavonoids (amentoflavone, 6,6′′-bigenkwanin, 7,7′′-dimethoxyagathisflavone, tetradimethoxy-6,6′′-bigenkwanin, cyanidin, cyanidin 3-galactoside, cyanidin 3-glucoside, delphinidin, eriodictyol, glyceollin, kaempferol, luteolin, malvidin, pelargonidin, pelargonidin 3-glucoside, and peonidin), and phenolics (eugenol, ferulic acid, vanillic acid, vanillylacetone, diferuloylputrescine, and p-coumaroylferuloylputrescine)]; hydroxamic acids (4-acetyl-2-benzoxazolinone and 6-methoxy-2-benzoxazolinone); terpenoides (camphene, canthaxanthin, α-carotene, β-carotene, β-cryptoxanthin, α-ionone, β-ionone, limonene, lutein, lycopene, and zeaxanthin); a tannin component (gallic acid); an inositol derivative (phytic acid), peroxidation products of unsaturated fatty acids, alkyl aldehydes (nonyl aldehyde); and signal molecules (ethylene and methyl jasmonate). Holmes et al. summarized aflatoxin production inhibitory activities of these inhibitors in detail and pointed out that many plant-derived inhibitors have antioxidant activity, but their modes of action for inhibiting aflatoxin production are not clear.
Scopoletin (7)Citation11) and (E)-2-hexenal (8)Citation12) were recently reported as the key compounds for obtaining resistance of cassava and soybean seed to aflatoxin contamination, respectively. As scopoletin, a phytoalexin of cassava, showed strong aflatoxin production inhibitory activity against A. flavus at the concentration of 2 mM, and content level of scopoletin in cassava was suggested to correlate with resistant level of cassava to aflatoxin contamination. (E)-2-Hexenal was identified as a volatile component produced by the exposure of soybean homogenates to lipase and shown to have strong inhibitory activity against aflatoxin production and growth of A. flavus after its 72 h continuous exposure. It was further shown that (E)-2-hexenal inhibits growth and aflatoxin production of the fungus in a model corn storage experiment.Citation13)
Peroxidation products of unsaturated fatty acids produced by the action of plant lipoxygenase (oxylipins), such as (13S)-hydroperoxy-9(Z), 11(E), 15(Z)-octadecatrienoic acid (9), have been known to inhibit aflatoxin production by affecting the G-protein-coupled receptor which regulates aflatoxin production,Citation10) but the activity of linolenic acid (C18:3) to aflatoxin production has been controversial. It was shown that pure linolenic acid has no aflatoxin production inhibitory activity. However, when linolenic acid was exposed to air, its peroxidation products were produced by autoxidation and the products inhibited aflatoxin production.Citation14)
Ethylene (10) and (2-chloroethyl) phosphonic acid (11), an ethylene-generating compound, inhibited aflatoxin production.Citation15) It was shown that (2-chloroethyl) phosphoric acid inhibits aflatoxin production due to oxidative stress alleviation.Citation16) It has been strongly suggested that oxidative stress has an important positive role in the onset of aflatoxin production in aflatoxigenic fungi.Citation17,18) However, it is not clear how oxidative stress leads to aflatoxin production or which reactive oxygen species is important for aflatoxin production.
There are many reports describing the effects of essential oils on the growth and aflatoxin production of aflatoxigenic fungi.Citation19) Essential oils are attractive for use in agriculture. Preparation of essential oils is relatively easy and it is possible to use the obtained crude oils in fields without pesticide registration. Therefore, aflatoxin production inhibitory activities of crude essential oils were reported in most papers and there are few cases that the active component in the essential oil showing aflatoxin production inhibitory activity was identified through a bioassay-guided fractionation.Citation20)
Constituents of essential oils of known plants have been exhaustively studied. Therefore, it is very difficult to find novel compounds by screening against essential oils. However, constituents of essential oils do not have complicated structures and their biosynthetic pathways may not be complicated. Therefore, they are suitable as lead compounds for developing effective inhibitors and genes responsible for their biosynthesis may be used to create transgenic plants that resist aflatoxin contamination by in situ production of an active compound.
To obtain aflatoxin production inhibitors with simple structures, that do not affect fungal growth, from essential oils, we performed screening among commercially available essential oils. Dillapiol (12), apiol (13), and spiroether (14) were found as specific inhibitors of the production of aflatoxins G1 and G2.Citation21,22) Our screening did not reveal any essential oils that can inhibit aflatoxin production by A. parasiticus without affecting fungal growth. The essential oil of Betula alba showed antifungal activity against A. parasiticus. While purifying antifungal compounds involved in this oil, we identified a fraction that showed inhibitory activity against aflatoxin production without affecting fungal growth. Methyl syringate (15) was isolated from the fraction as a selective aflatoxin production inhibitor.Citation23)
Methyl syringate is a derivative of gallic acid (16), which is known as an inhibitor of aflatoxin production by A. flavus. Among the gallic acid derivatives, methyl gallate (17), methyl 3,4,5-trimethoxybenzoate (18), and methyl 3-O-methylgallate (19) inhibited both aflatoxin production and fungal growth of A. parasiticus and A. flavus. Their acids (16, 20, 21) and syringic acid (22) did not inhibit aflatoxin production or growth of A. parasiticus significantly. Among the prepared syringic acid esters, heptyl syringate (23) showed the strongest inhibitory activity against aflatoxin production (IC50 values of methyl syringate and heptyl syringate for inhibition of aflatoxin production of A. parasiticus: 900 and 37 μM, respectively) (unpublished result). The aflatoxin production inhibitory activity of gallic acid is thought to be related to its antioxidant activity.Citation10) The 2,2-diphenyl-1-picrylhydrazyl (DPPH) radical-scavenging activity of methyl syringate was much weaker than that of gallic acid. These results showed that methyl syringate has a unique inhibitory activity toward aflatoxin production with a different mode of action from that of gallic acid. Recently, 4-O-methylgallic acid (24) was shown to inhibit aflatoxin production of A. flavus at the concentration of 2 mg/mL without affecting the fungal growth.Citation24)
Microbial metabolites
Many bioactive compounds produced by a variety of microbes are practically used not only in medicine but also in agriculture. Microbial metabolites generally have the advantage of being able to be prepared on a large scale by fermentation. Microbes are also useful as biocontrol agents in agriculture. Therefore, a microbe that produces an aflatoxin production inhibitor may have potential usefulness in aflatoxin control (see Fig. ).
Fig. 2. Aflatoxin production inhibitors from microbial metabolites and related compounds.
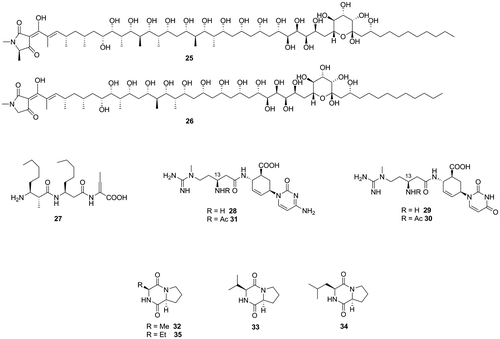
We performed a screening search for aflatoxin production inhibitors among microbial metabolites and found aflastatin A (25), a metabolite of Streptomyces sp., as the first aflatoxin production inhibitor of microbial origin.Citation25) We showed that blasticidin A (26), a Streptomyces metabolite whose structure had not been determined, has aflatoxin production inhibitory activity and structure similar to those of aflastatin A.Citation26) Dioctatin A (27), a metabolite of Streptomyces sp., was also shown to inhibit aflatoxin production.Citation27) The first author has reviewed works on these inhibitors of microbial origin.Citation28)
Blasticidin A was shown to inhibit protein synthesis. Some protein synthesis inhibitors, including blasticidin S (28), were found to show selective activity for aflatoxin production.Citation29) As A. flavus rapidly detoxifies blasticidin S by metabolizing the compound to the nontoxic deaminohydroxyblasticidin S (29) with blasticidin S deaminase, we asked whether blasticdin S inhibits aflatoxin production by mechanisms other than its protein synthesis inhibitory activity.
Analysis of metabolites in blasticidin S-treated A. flavus showed that blasticidin S was metabolized to a novel blasticidin S metabolite, N-acetyldeaminohydroxyblasticidin S (30).Citation30) In vivo and in vitro conversion experiments with blasticidin S, deaminohydroxyblasticidin S, and N-acetylblasticidin S (31) revealed that A. flavus converted blasticidin S to N-acetyldeaminohydroxyblasticidin S via deaminohydroxyblasticidin S. Interestingly, although deaminohydroxyblasticidin S lost antifungal activity, it maintained inhibitory activity against aflatoxin production (IC50 values of blasticidin S and deaminohydroxyblasticidin S for inhibition of aflatoxin production of A. flavus: 24 and 109 μM, respectively). N-Acetylblasticidin S and N-acetyldeaminohydroxyblasticidin S did not inhibit aflatoxin production. These results suggested the importance of the free amino group at C-13 of the blasticidin S skeleton for aflatoxin production inhibitory activity.
Acetyl-CoA was necessary for the acetylation of deaminohydroxyblasticidin S to N-acetyldeaminohydroxyblasticidin S while acetyl-CoA was known as the precursor for aflatoxin biosynthesis. When A. flavus was cultured with deaminohydroxyblasticidin S, the amount of acetyl-CoA consumed by acetylation of deaminohydroxyblasticidin S to N-acetyldeaminohydroxyblasticidin S was approximately equal to the amount of acetyl-CoA saved by reducing aflatoxin B1 production. This finding suggested that intracellular acetyl-CoA was consumed by the acetylation of deaminohydroxyblasticidin S, which caused a decrease in aflatoxin biosynthesis. These results indicated that a compound that can trap acetyl-CoA used for aflatoxin biosynthesis would be a good candidate for aflatoxin production inhibitors with high selectivity.
A bacterium, named strain no. 27, was found by our screening search for soil bacteria which produce aflatoxin production inhibitors. Cyclo(l-Ala-l-Pro) (32) and cyclo(l-Val-l-Pro) (33) were identified as aflatoxin production inhibitors produced by the strain.Citation9) Since cyclo(l-Leu-l-Pro) (34) had been isolated from Achromobacter xylosoxidans as an aflatoxin production inhibitor,Citation31) these diketopiperadines were thought to be good lead compounds for developing effective inhibitors. Among the four stereoisomers of cyclo(Ala-Pro), only cyclo(l-Ala-l-Pro) showed aflatoxin production inhibitory activity. Among synthetic cyclo(l-Ala-l-Pro) derivatives in which the alanine methyl side chain was replaced by longer alkyl groups, the ethyl derivative (35) inhibited aflatoxin production more strongly than cyclo(l-Ala-l-Pro) (IC50 values of cyclo(l-Ala-l-Pro), cyclo(l-Val-l-Pro), and the ethyl derivative (35) for inhibition of aflatoxin production of A. flavus: 0.68, 0.70, and 0.46, respectively), whereas derivatives with longer side chains showed weak or very weak activities (unpublished result).
Cyclo(l-Ala-l-Pro) reduced mRNA level of aflR, similarly to cyclo(l-Leu-l-Pro). AflR is a key regulatory protein for aflatoxin production. The aflR gene is involved in a gene cluster of aflatoxin biosynthesis. AflR regulates expression of genes encoding aflatoxin biosynthetic enzymes in the cluster. Therefore, expression of AflR is absolutely necessary for aflatoxin biosynthesis. The regulatory mechanism for AflR expression has not been clarified well and may involve many factors concerned with the regulation. Cyclo(l-Ala-l-Pro) may affect some parts of the regulation. Therefore, uncovering the mode of action of cyclo(l-Ala-l-Pro) may provide an important clue to understanding this regulatory mechanism.
Strain no. 27 was identified as Stenotrophomonas sp., most similar to Stenotrophomonas rhizophila. The Stenotrophomonas species are known to be promising candidates for biocontrol agents in agriculture due to their beneficial functions. S. rhizophila is especially suitable for such applications due to its non-pathogenic property. Therefore, we explored the possibility of using strain no. 27 as a biocontrol agent for aflatoxin production.Citation9) When A. flavus was co-cultured with strain no. 27 in a liquid medium, aflatoxin production was reduced without affecting fungal growth. Inhibition of aflatoxin production was dependent on the bacterial cell numbers used for co-culture. Culture broth obtained by the co-culture in which aflatoxin production was inhibited contained the two diketopiperadines at sufficient concentrations to inhibit aflatoxin production. These results indicated that strain no. 27 may be an effective aflatoxin biocontrol agent.
Aflatoxin production inhibitors from synthetic compounds
If known pesticides can effectively prevent aflatoxin contamination of crops, their new use in agriculture may be developed, although the environmental impact of using synthetic pesticides is stronger than that of natural compounds. Organophosphorous insecticides with cholinesterase inhibitory activity, such as dichlorvos (36) and inhibited aflatoxin production.Citation32) They may inhibit the esterase catalyzing the step from versiconal hemiacetal acetate to versiconal in the aflatoxin biosynthetic pathway. Some inhibitors of pentaketide-derived melanin biosynthesis in fungi, such as tricyclazole (37), showed aflatoxin production inhibitory activity.Citation33) It is suggested that they inhibit a reductase involved in the conversion step from versicolorin A to demethylsterigmatocystin in the aflatoxin biosynthetic pathway (see Fig. ).
Fig. 3. Aflatoxin production inhibitors from pesticides, respiration inhibitors, and synthetic bioactive compounds.
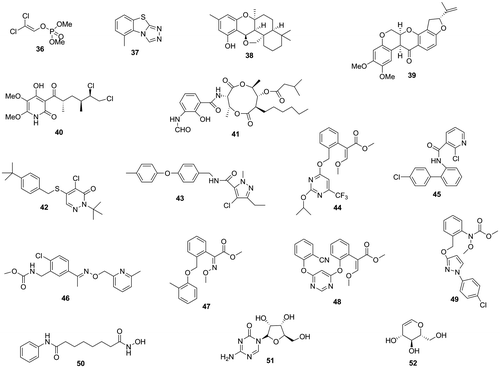
By the screening against a chemical library (RIKEN Natural Products Depositor), we found that siccanin (38), a known respiration inhibitor, inhibits aflatoxin production by A. parasiticus. As the relationship between inhibitory activities of respiration and aflatoxin production had not been reported previously, we examined whether other known respiration inhibitors including synthetic pesticides also had inhibitory activity against aflatoxin production.Citation34)
Four natural respiration inhibitors, rotenone (39) (a complex I inhibitor), siccanin and atpenin A5 (40) (complex II inhibitors), and antimycin A (41) (a complex III inhibitor), inhibited the production of aflatoxin by A. parasiticus without affecting fungal growth significantly and with similar IC50 values of around 10 μM in liquid culture. Among the synthetic miticides with respiration inhibitory activity, pyridaben (42), tolfenpyrad (43) (complex I inhibitors), and fluacrypyrim (44) (a complex III inhibitor) demonstrated strong inhibitory activities with IC50 values of less than 0.2 μM. Among the synthetic fungicides, boscalid (45) (a complex II inhibitor) showed the strongest aflatoxin production inhibitory activity with an IC50 value of less than 0.01 μM. Pyribencarb (46), kresoxim-methyl (47), azoxystrobin (48), and pyraclostrobin (49) (complex III inhibitors) inhibited aflatoxin production strongly with IC50 values of less than 0.5 μM. From the inhibitory activities of known respiration inhibitors tested, it was difficult to identify a correlation between the targets of the respiration inhibitors (complexes I, II, and III) and their IC50 values for inhibitory activity against aflatoxin production. This suggested that respiration inhibitors with a variety of targets may potentially have inhibitory activities against aflatoxin production.
The effects of boscalid and tolfenpyrad on the mRNA levels of aflR and three genes (pacC, facB, and laeA) encoding regulatory proteins necessary for aflR expression as well as genes present in the aflatoxin biosynthetic gene cluster were examined to investigate the mode of action of respiration inhibitors for the inhibition of aflatoxin production. Boscalid and tolfenpyrad did not reduce the mRNA levels of aflR or any of the other tested genes (unpublished result). This finding suggested that respiration inhibitors may alter the metabolic flow of key molecules, such as acetyl-CoA, important for aflatoxin production.
Aflatoxin production inhibitory activity of a synthetic bioactive compound with known mode of action may be useful for investigating the regulatory mechanism of aflatoxin production in aflatoxigenic fungi. A calmodulin antagonist (trifluoperazine) and calcium channel blockers (verapamil and diltiazem) inhibited aflatoxin production,Citation10) suggesting a relationship of aflatoxin production with calcium signaling. Aflatoxin production inhibitory activities of suberoylanilide hydroxamic acid (50),Citation35) a histone deacetylase inhibitor, 5-azacytidine (51),Citation36) an inactivator of DNA methyltransferase, d-glucal (52),Citation37) and a non-metabolizable glucose analog have been reported.
Trichothecene production inhibitors
Trichothecenes are produced by Fusarium and other fungal genera. They have a common trichothecene skeleton, 12,13-epoxytrichothec-9-ene, and contain troublesome mycotoxins such as nivalenol (53), deoxynivalenol (54), T-2 toxin (55), and HT-2 toxin (56). Among these trichothecene mycotoxins, deoxynivalenol is particularly troublesomeCitation38) because it contaminates important cereal crops, such as wheat. Deoxynivalenol causes vomiting and other toxicoses in exposed animals. Fusarium graminearum is a worldwide predominant pathogen that causes Fusarium head blight (FHB) of wheat and other grain cereals, and produces deoxynivalenol in infected grain. FHB epidemics reduce crop yield, and deoxynivalenol contamination limits grain utilization. At present, the use of fungicides is the most effective method for controlling FHB and deoxynivalenol contamination. However, inhibition of fungal growth sometimes leads to the rapid spread of resistant fungal strains. Deoxynivalenol production is not necessary for fungal growth or initial infection on wheat, but the spread of FHB is suppressed when trichothecene production is inhibited.Citation39) Therefore, specific inhibitors of deoxynivalenol production may be useful in protecting crops from both FHB and deoxynivalenol contamination.
Trichothecenes are biosynthesized from farnesyl pyrophosphate produced via the mevalonate pathway.Citation40) Mevalonate, the key intermediate in the pathway, is biosynthesized from three acetyl-CoA molecules. Tri genes are responsible for trichothecene biosynthesis from farnesyl pyrophosphate. Among the Tri genes, TRI6 encodes a key regulatory protein for trichothecene biosynthesis in F. graminearum.Citation41,42) TRI6 not only induces the expression of Tri genes encoding trichothecene biosynthetic enzymes, such as Tri4 and Tri5, but also upregulates expression of genes encoding enzymes involved in the mevalonate pathway, such as HMGS and HMGR (encoding hydroxymethylglutaryl-CoA synthase and hydroxymethylglutaryl-CoA reductase, respectively). Therefore, overall the biosynthetic pathway from acetyl-CoA to trichothecenes is activated by TRI6. Upstream events that lead to TRI6 expression have not yet been clarified.
There are many reports on plant constituents that affect growth and trichothecene production of trichothecene-producing fungi, similarly to the case of aflatoxin. We summarize trichothecene production inhibitors of such plant constituents and other sources such as inorganic and synthetic compounds and describe our work on a highly selective inhibitor, precocene II, obtained by our screening against essential oils.
Trichothecene production inhibitors from plant constituents, synthetic compounds, and inorganic compounds
In early studies, plant constituents, flavone (57) and some furanocoumarins (heraclenin (58), imperatorin (59), xanthotoxin (60), bergapten (61), pimpinellin (62), isopimpinellin (63), and psoralen (64)), and a synthetic compound, ancymidol (65), were shown to inhibit T-2 toxin production by Fusarium sporotrichioides at concentrations of around a few mM.Citation43,44) XanthotoxinCitation45) and ancymidol are inhibitors of cytochrome P-450 monooxygenase and accumulated trichodiene, a biosynthetic intermediate of trichothecene, in the culture broth of F. sporotrichioides. It was suggested that xanthotoxin may inhibit TRI4, which is a biosynthetic enzyme catalyzing early steps in the trichothecene biosynthesis from trichodiene to isotrichodermol. Inhibition of TRI4 by xanthotoxin, bergapten, psoralen, and flavone was shown by the assay system using transgenic Saccharomyces cerevisiae expressing Tri4.Citation46) Spiroether (14) also inhibited TRI4 and trichothecene production of F. graminearum (see Fig. ).Citation22)
Fig. 4. Trichothecenes, trichothecene production inhibitors, and precocene II-immobilized magnet bead (84).
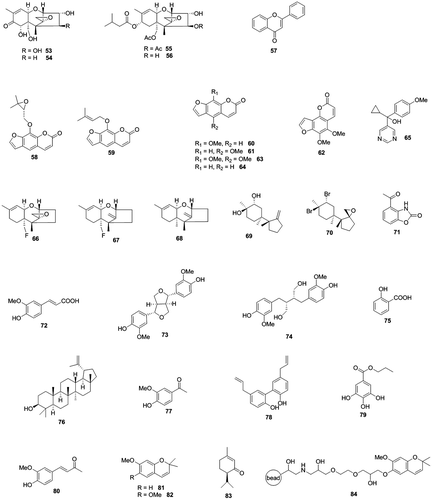
Inhibitors of trichothecene biosynthesis were designed based on structures of biosynthetic intermediates and synthesized. 15-Fluoroepoxytrichothecene (66), 15-fluorotrichotheca-9,12-diene (67), and trichothec-9,12-diene (68) were found to inhibit trichothecene production of Fusarium culmorum very effectively at the concentration of 0.25 mM.Citation47) Trichodiene analogs, diol (69) and epoxydibromide (70), reduced trichothecene production of F. culmorum very strongly.Citation48)
4-Acetyl-2-benzoxazolinone (71) was isolated from an extract of Gibberella ear rot-resistant corn by bioassay-directed fractionation.Citation49) It inhibited trichothecene production of F. culmorum in liquid culture at the IC50 value of 4 μM without affecting the fungal growth, but did not inhibit deoxynivalenol production of F. graminearum grown on the maize grain that contained this compound at the concentration of more than 100 μM.Citation50)
Ferulic acid (72), the most abundant phenolic acid in wheat bran, completely inhibited trichothecene production of F. culmorum at concentrations of higher than 1 mM in liquid medium by reducing mRNA levels of Tri genes including Tri6.Citation51) It was reported that inhibition of trichothecene production of F. graminearum by ferulic acid was not observed under a culture condition.Citation52) Pinoresinol (73) and secoisolariciresinol (74), the most prominent wheat lignans, showed strong inhibitory activity toward trichothecene production of F. graminearum in liquid culture at concentrations of around a few mg/L.Citation53) Pinoresinol repressed trichothecene production and mRNA levels of some Tri genes (Tri4, Tri5, and Tri11) more strongly than secoisolariciresinol. Ten compounds (capric acid, lauric acid, methyl jasmonate, p-coumaric acid, sinapic acid, quinic acid, ferulic acid, caffeic acid, naringenin, and quercetin), which were selected from resistance-related endogenous metabolites in barley, strongly inhibited trichothecene production of F. graminearum at their molar concentrations that inhibited 50% fungal biomass.Citation54) Salicylic acid (75), a plant signal molecule, inhibited deoxynivalenol production of F. graminearum at the concentration of 0.5 mM but its inhibitory activity required acidic growth conditions.Citation55) Bioassay-guided fractionation of extracts of Schinopsis species from Argentina revealed that lupeol (76) is a strong trichothecene production inhibitor which completely inhibits deoxynivalenol production of F. graminearum in liquid culture without affecting fungal growth at the concentration of 125 μg/mL.Citation56)
Recently, inhibitors of trichothecene production of F. culmorum were screened against many natural and natural-like phenolic compounds.Citation57) Apocynin (77), magnolol (78), propyl gallate (79), and dehydrozingerone (80) were found to inhibit trichothecene production strongly in a dose-dependent manner. Among these compounds, magnolol showed strongest trichothecene production inhibitory activity together with fungicidal activity.
Trichothecene production of Fusarium tricinctum was inhibited by sodium bicarbonate at the concentration of 0.11 M.Citation58) As production of geraniol and carotenoids by the fungi was also reduced, it was suggested that sodium bicarbonate affects the mevalonate pathway to reduce terpenoids production.Citation59) Mg2+ and Mn2+ inhibited trichothecene production of F. graminearum. Mg2+ was shown to suppress mRNA levels of Tri5, Tri6, and Tri12.Citation60) On the other hand, Fe2+, Zn2+, and Co2+ stimulated trichothecene production.Citation61,62) Co2+ strongly upregulated mRNA levels of Tri4 and Tri6.
Precocene II
Trichothecene production inhibitors from essential oils
There are a few reports on the effects of essential oils on trichothecene production.Citation63) As any active principles in the effective essential oils had not been identified, we performed screening search for inhibitors of trichothecene production of F. graminearum in liquid culture among commercially available essential oils.
Precocenes I (81) and II (82) and piperitone (83) were isolated from essential oils of Matricaria recutita and Eucalyptus dives, respectively, as trichothecene production inhibitors.Citation64) Precocene II showed stronger activity than precocene I with an IC50 value of a few micromolars in liquid culture. The activity of piperitone was very weak. Precocenes and piperitone did not affect the growth of F. graminearum significantly. Precocene II strongly inhibited deoxynivalenol production in solid culture on rice, suggesting its potential utility for field application.
Mode of action of precocene II
Because precocene II showed strong inhibitory activity against trichothecene production of F. graminearum without affecting fungal growth, we investigated its mode of action, in order to clarify the regulatory mechanism for trichothecene production by F. graminearum. Historically, precocene II was first isolated as a constituent of essential oils of Ageratum species and named ageratochromene.Citation65) After the discovery of its specific anti-juvenile hormone activity in insects,Citation66) ageratochromene was renamed as precocene II, by which it is commonly known today. Identification of the target molecule of precocene II in insects would be very important for the development of insect growth regulators, but it has not been clarified yet.
We found that precocene II reduced the mRNA levels of Tri6 and other genes (Tri4, Tri5, HMGS, and HMGR) whose expression is regulated by TRI6, suggesting that precocene II may affect the pathway leading to Tri6 expression.Citation64)
We further examined the effects of precocene II on some basic metabolic activities. Although F. graminearum can grow in liquid media containing a variety of carbon sources such as glucose, fructose, or sucrose, high-level trichothecene production occurs only in sucrose-containing media.Citation67) In the presence of sucrose, expression of Tri6 and other Tri genes is induced and that of genes in the mevalonate pathway is enhanced. Because F. graminearum has a set of genes encoding enzymes involved in the mevalonate pathway, the enhanced expression of genes in this mevalonate pathway may have a role in supplying biosynthetic precursors for trichothecene production. Precocene II did not affect the production of ergosterol, which is an essential membrane component biosynthesized through the mevalonate pathway. Therefore, it seemed that the supply of additional acetyl-CoA to the mevalonate pathway may be a key event for initiating trichothecene production.Citation68)
We quantified the amount of acetyl-CoA in fungal cells cultured with or without precocene II and/or sucrose. Precocene II reduced the amount of acetyl-CoA to the level observed in cells cultured without sucrose, in which trichothecene was not produced.Citation68) By the proteome analysis with two-dimensional differential gel electrophoresis, it was found that expression of ATP citrate lyase (ATPCL) in the presence of sucrose was significantly reduced by precocene II.Citation68) ATPCL is a key enzyme to produce acetyl-CoA in the fungal cytosol. Its deletion affects trichothecene production and its expression is not under the control of TRI6.Citation41) The mRNA level of ATPCL was upregulated in the presence of sucrose and precocene II reduced it to the level without sucrose. These results suggested that ATPCL expression is activated in the presence of sucrose, and that acetyl-CoA produced by the increased ATPCL may be used for trichothecene production in the fungus.Citation68) Precocene II may affect both pathways which lead to ATPCL and TRI6 expression in the presence of sucrose.
According to histological studies in insects and rats, precocene II affects mitochondrial function and structure,Citation69–71) suggesting that precocene II may attack a component present in mitochondria directly. We used an affinity magnetic bead method to detect the precocene II-binding protein in mitochondria. O-Alkyl derivatives of 6-O-demethylprecocene II can maintain relatively strong inhibitory activities for trichothecene production (unpublished result). Therefore, we prepared precocene II-immobilized magnetic nanobeads (84). The beads were incubated with a protein extract from the mitochondrial fraction of F. graminearum protoplasts and eluted with a precocene II-containing solution. A band around 30 kDa observed on the sodium dodecyl sulfate–polyacrylamide gel electrophoresis gel of the precocene II-eluted fraction was disappeared by a competitive experiment, suggesting involvement of a specific binding protein of precocene II in the band. LC/MS/MS analysis showed that voltage-dependent anion channel (VDAC), a mitochondrial outer membrane protein, was the most probable candidate for the precocene II-binding protein in the band. The binding of VDAC with the precocene II-immobilized beads was confirmed by experiments with recombinant His-tagged VDAC and with Saccharomyces cerevisiae mitochondria.Citation72)
VDAC, which is responsible for fluxes of ATP, NADH, and other low-molecular weight metabolites,Citation73) may be related to superoxide (O2.−) release from the mitochondrial intermembrane space into the cytosol.Citation74,75) Superoxide in mitochondria is generated as a byproduct of the mitochondrial electron transport chain reactions, and some of the generated superoxide is released into the intermembrane space. Superoxide-generating agents, paraquat and menadione, inhibited trichothecene production.Citation72) Therefore, we focused on the superoxide-releasing role of VDAC to investigate the mode of action of precocene II for inhibiting trichothecene production.
We analyzed superoxide levels in the fungal cells, which were cultured with precocene II or paraquat, using two superoxide-specific fluorescent dyes, MitoSOX red and dihydroethidium. MitoSOX and dihydroethidium are predominantly localized to the mitochondria and cytoplasm, respectively. By the observation under a fluorescent microscope, we found that precocene II increased the superoxide level only in mitochondria, whereas paraquat increased the superoxide levels in both mitochondria and the cytoplasm.Citation72) Therefore, precocene II and paraquat may have different modes of action.
We measured the time course of the mitochondrial superoxide level in fungal cells to clarify the relationship between superoxide level and trichothecene production. The mitochondrial superoxide level gradually decreased during the growth phase to become very low when trichothecene production began. Addition of precocene II at the beginning of cultivation enhanced the mitochondrial superoxide level during the growth phase. The mRNA level of Tri6 gradually increased antiparallel to the mitochondrial superoxide level. These facts suggested that a low level of mitochondrial superoxide was required for the onset of trichothecene production.Citation72)
Experiments with antioxidants supported the above hypothesis. We tested the effects of three natural antioxidants (ascorbic acid, glutathione, and α-tocopherol) on trichothecene production and precocene II activity. These antioxidants, especially α-tocopherol, enhanced trichothecene production by the fungus very strongly and compensated for the inhibitory activity of precocene II on trichothecene production. On the other hand, hydrogen peroxide, which is produced by superoxide dismutase and known to enhance trichothecene production of a Fusarium strain,Citation76) did not significantly affect the trichothecene production of the strain we used. These facts strongly suggested that superoxide, especially mitochondrial superoxide, is a key molecule for trichothecene production.Citation72)
On the basis of the results described above, we hypothesized the mode of action of precocene II for inhibiting trichothecene production by F. graminearum, as shown in Fig. . When the superoxide level in mitochondria reaches a threshold in the presence of sucrose, the expression of ATPCL is activated and that of TRI6 is induced. Acetyl-CoA molecules produced by ATPCL are used for trichothecene production through the activated mevalonate pathway. Precocene II binds to VDAC and increases superoxide level in mitochondria, which leads to immediate stopping of trichothecene production via suppression of ATPCL and TRI6 expression levels and acetyl-CoA amount by an unknown mechanism.
Conclusions
Mycotoxin production inhibitors can be obtained from a variety of sources. They may be useful for mycotoxin control in crops and for basic research on the regulatory mechanisms of fungal metabolism. Microbial metabolites, plant constituents, especially essential oils, and known pesticides are important sources for developing practically useful inhibitors. Microbes that produce mycotoxin production inhibitors may be useful as biocontrol agents. The modes of action of mycotoxin production inhibitors, such as precocene II, afford important clues for clarifying the regulatory mechanisms for fungal secondary metabolite production and for developing novel methods to control mycotoxin contamination. Additional applied and basic research should be performed to obtain information regarding mycotoxin production inhibitors.
Disclosure statement
No potential conflict of interest was reported by the authors.
Funding
This work was supported by the Program for Promotion of Basic and Applied Researches for Innovations in Bio-oriented Industry; Research project for improving food safety and animal health.
References
- Richard JL, Payne GA. Mycotoxins: risks in plant, animal and human systems. Ames, IA: Council for Agricultural Science and Technology Press; 2003.
- Bennett JW, Klich M. Mycotoxins. Clin. Microbiol. Rev. 2003;16:497–516.10.1128/CMR.16.3.497-516.2003
- Strosnider H, Azziz-Baumgartner E, Banziger M, et al. Workgroup report: public health strategies for reducing aflatoxin exposure in developing countries. Environ. Health Perspectives. 2006;114:1898–1903.
- Wu F. Global impacts of aflatoxin in maize: trade and human health. World Mycotoxin J. 2015;8:137–142.10.3920/WMJ2014.1737
- Liu Y, Chang CCH, Marsh GM, et al. Population attributable risk of aflatoxin-related liver cancer: systematic review and meta-analysis. Eur. J. Cancer. 2012;48:2125–2136.10.1016/j.ejca.2012.02.009
- Abbas HK, Wilkinson JR, Zablotowicz RM, et al. Ecology of Aspergillus flavus, regulation of aflatoxin production, and management strategies to reduce aflatoxin contamination of corn. Toxin Rev. 2009;28:142–153.10.1080/15569540903081590
- Abbas HK, Weaver MA, Horn BW, et al. Selection of Aspergillus flavus isolates for biological control of aflatoxins in corn. Toxin Rev. 2011;30:59–70.10.3109/15569543.2011.591539
- Ehrlich KC, Moore GG, Mellon JE, et al. Challenges facing the biological control strategy for eliminating aflatoxin contamination. World Mycotoxin J. 2015;8:225–233.10.3920/WMJ2014.1696
- Jermnak U, Chinaphuti A, Poapolathep A, et al. Prevention of aflatoxin contamination by a soil bacterium of Stenotrophomonas sp. that produces aflatoxin production inhibitors. Microbiology. 2013;159:902–912.10.1099/mic.0.065813-0
- Holmes RA, Boston RS, Payne GA. Diverse inhibitors of aflatoxin biosynthesis. Appl. Microbiol. Biotechnol. 2008;78:559–572.10.1007/s00253-008-1362-0
- Gnonlonfin GJB, Adjovi Y, Gbaguidi F, et al. scopoletin in cassava products as an inhibitor of aflatoxin production. J. Food Safety. 2011;31:553–558.10.1111/jfs.2011.31.issue-4
- Cleveland TE, Carter-Wientjes CH, De Lucca AJ, et al. Effect of soybean volatile compounds on Aspergillus flavus growth and aflatoxin production. J. Food Sci. 2009;74:H83–H87.10.1111/jfds.2009.74.issue-2
- De Lucca AJ, Carter-Wientjes CH, Boué S, et al. Volatile trans-2-hexenal, a soybean aldehyde, inhibits Aspergillus flavus growth and aflatoxin production in corn. J. Food Sci. 2011;76:M381–M386.10.1111/jfds.2011.76.issue-6
- Yan S, Liang Y, Zhang J, et al. Autoxidated linolenic acid inhibits aflatoxin biosynthesis in Aspergillus flavus via oxylipin species. Fungal Gen. Biol. 2015;81:229–237.
- Gunterus A, Roze LV, Beaudry R, et al. Ethylene inhibits aflatoxin biosynthesis in Aspergillus parasiticus grown on peanuts. Food Microbiol. 2007;24:658–663.10.1016/j.fm.2006.12.006
- Huang JQ, Jiang HF, Zhou YQ, et al. Ethylene inhibited aflatoxin biosynthesis in due to oxidative stress alleviation and related to glutathione redox state changes in Aspergillus flavus. Int. J. Food Microbiol. 2009;130:17–21.10.1016/j.ijfoodmicro.2008.12.027
- Jayashree T, Subramanyam C. Oxidative stress as a prerequisite for aflatoxin production by Aspergillus parasiticus. Free Radical Biol. Med. 2000;29:981–985.10.1016/S0891-5849(00)00398-1
- Grintzalis K, Vernardis SI, Klapa MI, et al. Role of oxidative stress in sclerotial differentiation and aflatoxin B1 biosynthesis in Aspergillus flavus. Appl. Environ. Microbiol. 2014;80:5561–5571.10.1128/AEM.01282-14
- Alpsoy L. Inhibitory effect of essential oil on aflatoxin activities. Afr. J. Biotechnol. 2010;9:2474–2481.
- Razzaghi-Abyaneh M, Shams-Ghahfarokhi M, Yoshinari T, et al. Inhibitory effects of Satureja hortensis L. essential oil on growth and aflatoxin production by Aspergillus parasiticus. Int. J. Food Microbiol. 2008;123:228–233.10.1016/j.ijfoodmicro.2008.02.003
- Razzaghi-Abyaneh M, Yoshinari T, Shams-Ghahfarokhi M, et al. Dillapiol and apiol as a specific inhibitors of the biosynthesis of aflatoxin G1 in Aspergillus parasiticus. Biosci. Biotechnol. Biochem. 2007;71:2329–2332.10.1271/bbb.70264
- Yoshinari T, Yaguchi A, Takahashi-Ando N, et al. Spiroethers of German chamomile inhibit production of aflatoxin G1 and trichothecene mycotoxin by inhibiting cytochrome P450 monooxygenases involved in their biosynthesis. FEMS Microbiol. Lett. 2008;284:184–190.10.1111/fml.2008.284.issue-2
- Jermnak U, Yoshinari T, Sugiyama Y, et al. Isolation of methyl syringate as a specific aflatoxin production inhibitor from the essential oil of Betula alba and aflatoxin production inhibitory activities of its related compounds. Int. J. Food Microbiol. 2012;153:339–344.10.1016/j.ijfoodmicro.2011.11.023
- Abhishek RU, Mohana DC, Thippeswamy S, et al. Evaluation of Phyllanthus polyphyllu L. extract and its active constituent as a souce of antifungal, anti-aflatoxigenic, and antioxidant activities. Int. J. Food Prop. 2015;18:585–596.10.1080/10942912.2013.853187
- Sakuda S, Ono M, Furihata K, et al. Aflastatin A, a novel inhibitor of aflatoxin production of Aspergillus parasiticus, from Streptomyces. J. Am. Chem. Soc. 1996;118:7855–7856.10.1021/ja960899d
- Sakuda S, Ono M, Ikeda H, et al. Blasticidin A as an inhibitor of aflatoxin production by Aspergillus parasiticus. J. Antibiot. 2000;53:1265–1271.10.7164/antibiotics.53.1265
- Yoshinari T, Akiyama T, Nakamura K, et al. Dioctatin A is a strong inhibitor of aflatoxin production by Aspergillus parasiticus. Microbiology. 2007;153:2774–2780.10.1099/mic.0.2006/005629-0
- Sakuda S. Mycotoxin production inhibitors from natural products. Mycotoxins. 2010;60:79–86.10.2520/myco.60.79
- Yoshinari T, Noda Y, Yoda K, et al. Inhibitory activity of blasticidin A, a strong aflatoxin production inhibitor, on protein synthesis of yeast: selective inhibition of aflatoxin production by protein synthesis inhibitors. J. Antibiot. 2010;63:309–314.10.1038/ja.2010.36
- Yoshinari T, Sakuda S, Watanabe M, et al. New metabolic pathway for converting blasticidin S in Aspergillus flavus and inhibitory activity of aflatoxin production by blasticidin S metabolites. J. Agric. Food Chem. 2013;61:7925–7931.10.1021/jf402745c
- Yan PS, Song Y, Sakuno E, et al. Cyclo(L-Leucyl-L-Prolyl) produced by Achromobacter xylosoxidans inhibits aflatoxin production by Aspergillus parasiticus. Appl. Environ. Microbiol. 2004;70:7466–7473.10.1128/AEM.70.12.7466-7473.2004
- Dutton MF, Anderson MS. Inhibition of aflatoxin biosynthesis by organophosphorous compounds. J. Food Prot. 1980;43:381–384.
- Wheeler MH, Bhatnagar D, Rojas MG. Chlobenthiazone and tricyclazole inhibition of aflatoxin biosynthesis by Aspergillus flavus. Pestic. Biochem. Physiol. 1989;35:315–323.10.1016/0048-3575(89)90092-8
- Sakuda S, Prabowo DF, Takagi K, et al. Inhibitory effects of respiration inhibitors on aflatoxin production. Toxins. 2014;6:1193–1200.10.3390/toxins6041193
- Basimia T, Rezaee S, Zamanizadeh HR, et al. SAHA, histone deacetylase inhibitor causes reduction of aflatoxin production and conidiation in the Aspergillus flavus. Int. J. Biosci. 2013;3:9–16.
- Lin JQ, Zhao XX, Wang CC, et al. 5-Azacytidine inhibits aflatoxin biosynthesis in Aspergillus flavus. Ann. Microbiol. 2013;63:763–769.10.1007/s13213-012-0531-7
- Zhang JD, Han L, Yan S, et al. The non-metabolizable glucose analog D-glucal inhibits aflatoxin biosynthesis and promotes kojic acid production in Aspergillus flavus. BMC Microbiol. 2014;14:95/1–95/9.
- Pestka JJ, Smolinski AT. Deoxynivalenol: toxicology and potential effects on humans. J. Toxicol. Environ. Health B Crit. Rev. 2005;8:39–69.10.1080/10937400590889458
- Bai GH, Desjardins AE, Plattner RD. Deoxynivalenol-non-producing Fusarium graminearum causes initial infection, but does not cause disease spread in wheat spikes. Mycopathologia. 2001;153:91–98.
- Kimura M, Tokai T, Takahashi-Ando N, et al. Molecular and genetic studies of Fusarium trichothecene biosynthesis: pathways, genes, and evolution. Biosci. Biotechnol. Biochem. 2007;71:2105–2123.10.1271/bbb.70183
- Seong KY, Pasquali M, Zhou X, et al. Global gene regulation by Fusarium transcription factors Tri6 and Tri10 reveals adaptations for toxin biosynthesis. Mol. Microbiol. 2009;72:354–367.10.1111/mmi.2009.72.issue-2
- Nasmith CG, Walkowiak S, Wang L, et al. Tri6 is a global transcription regulator in the phytopathogen Fusarium graminearum. PLoS Pathog. 2011;7:e1002266.10.1371/journal.ppat.1002266
- Desjardins AE, Plattner RD, Spencer GF. Inhibition of trichothecene toxin biosynthesis by naturally occurring shikimate aromatics. Phytochem. 1988;27:767–771.10.1016/0031-9422(88)84090-1
- Desjardins AE, Plattner RP, Beremand MN. Ancymidol blocks trichorhecene biosynthesis and leads to accumulation of trichodiene in Fusarium sporotrichioides and Gibberella pulicaris. Appl. Environ. Micorobiol. 1987;53:1860–1865.
- Alexander NJ, McCormick SP, Blackburn JA. Effects of xanthotoxin treatment on trichothecene production in Fusarium sporotrichioides. Can. J. Microbiol. 2008;54:1023–1031.10.1139/W08-100
- Takahashi-Ando N, Ochiai N, Tokai T, et al. A screening system for inhibitors of trichothecene biosynthesis: hydroxylation of trichodiene as a target. Biotechnol. Lett. 2008;30:1055–1059.10.1007/s10529-008-9649-x
- Zamir LO, Rotter B, Devor KA, et al. Target-oriented inhibitors of the late stages of trichothecene biosynthesis. 2. In vivo inhibitors and chick embryotoxicity bioassay. J. Agric. Food Chem. 1992;40:681–685.10.1021/jf00016a032
- Hesketh AR, Gledhill L, Bycroft BW, et al. Potential inhibitors of trichothecene biosynthesis in Fusarium culmorum: epoxidation of a trichodiene derivative. Phytochemistry. 1993;32:93–104.
- Miller JD, Fielder DA, Dowd PF, et al. Isolation of 4-acetyl-benzoxazolin-2-one (4-ABOA) and diferuloylputrescine from an extract of gibberella ear rot-resistant corn that blocks mycotoxin biosynthesis, and the insect toxicity of 4-ABOA and related compounds. Biochem. Syst. Ecol. 1996;24:647–658.10.1016/S0305-1978(96)00050-6
- Bakan B, Bily AC, Melcion D, et al. Possible role of plant phenolics in the production of trichothcenes by Fusarium graminearum strains on different fractions of maize kernels. J. Agric. Food Chem. 2003;51:2826–2831.10.1021/jf020957g
- Boutigny AL, Barreau C, Atanasova-Penichon V, et al. Ferulic acid, an efficient inhibitor of type B trichothecene biosynthesis and Tri gene expression in Fusarium liquid cultures. Mycol. Res. 2009;113:746–753.10.1016/j.mycres.2009.02.010
- Ponts N, Pinson-Gadais L, Boutigny AL, et al. Cinnamic-derived acids significantly affect Fusarium graminearum growth and in vitro synthesis of type B trichothecenes. Phytopathol. 2011;101:929–934.10.1094/PHYTO-09-10-0230
- Kulik T, Buśko M, Pszczółkowska A, et al. Plant lignans inhibit growth and trichothecene biosynthesis in Fusarium graminearum. Lett. Appl. Microbiol. 2014;59:99–107.10.1111/lam.2014.59.issue-1
- Bollina V, Kushalappa AC. In vitro inhibition of trichothecene biosynthesis in Fusarium graminearum by resistance-related endogenous metabolites identified in barley. Mycology. 2011;2:291–296.
- Qi PF, Johnston A, Balcerzak M, et al. Effect of salicylic acid on Fusarium graminearum, the major causal agent of fusarium head blight in wheat. Fungal Biol. 2012;116:413–426.10.1016/j.funbio.2012.01.001
- Aristimuño Ficoseco MEA, Vattuone MA, Audenaert K, et al. Antifungal and antimycotoxigenic metabolites in Anacardiaceae species from northwest Argentina: isolation, identification and potential for control of Fusarium species. J. Appl. Microbiol. 2014;116:1262–1273.10.1111/jam.2014.116.issue-5
- Pani G, Scherm B, Azara E, et al. Natural and natural-like phenolic inhibitors of type B trichothecene in vitro production by the wheat (Triticum sp.) pathogen Fusarium culmorum. J. Agric. Food Chem. 2014;62:4969–4978.10.1021/jf500647h
- Roinestad KS, Montville TJ, Rosen JD. Inhibition of trichothecene biosynthesis in Fusarium tricinctum by sodium bicarbonate. J. Agric. Food Chem. 1993;41:2344–2346.10.1021/jf00036a024
- Roinestad K, Montville TJ, Rosen JD. Mechanism for sodium bicarbonate inhibition of trichothecene biosynthesis in Fusarium tricinctum. J. Agric. Food Chem. 1994;42:2025–2028.10.1021/jf00045a036
- Pinson-Gadais L, Richard-Forget F, Frasse P, et al. Magnesium represses trichothecene biosynthesis and modulates Tri5, Tri6, and Tri12 genes expression in Fusarium graminearum. Mycopathologia. 2008;165:51–59.10.1007/s11046-007-9076-x
- Vasavada AB, Hsieh DPH. Effects of metals on 3-acetyldeoxynivalenol production by Fusarium graminearum R2118 in submerged cultures. Appl. Environ. Microbiol. 1988;54:1063–1065.
- Tsuyuki R, Yoshinari T, Sakamoto N, et al. Enhancement of trichothecene production in Fusarium graminearum by cobalt chloride. J. Agric. Food Chem. 2011;59:1760–1766.10.1021/jf103969d
- Marín S, Velluti A, Ramos AJ, et al. Effect of essential oils on zearalenone and deoxynivalenol production by Fusarium graminearum in non-sterilized maize grain. Food Microbiol. 2004;21:313–318.10.1016/j.fm.2003.08.002
- Yaguchi A, Yoshinari T, Tsuyuki R, et al. Isolation and identification of precocenes and piperitone from essential oils as specific inhibitors of trichothecene production by Fusarium graminearum. J. Agric. Food Chem. 2009;57:846–851.10.1021/jf802813h
- Alertsen AR. Ageratochromene, a heterocyclic compound from the essential oils of some Ageratum Species. Acta Chem. Scand. 1955;9:1725–1726.10.3891/acta.chem.scand.09-1725
- Bowers WS, Ohta T, Cleere JS, et al. Discovery of insect anti-juvenile hormones in plants. Science. 1976;193:542–547.10.1126/science.986685
- Jiao F, Kawakami A, Nakajima T. Effects of different carbon sources on trichothecene production and Tri gene expression by Fusarium graminearum in liquid culture. FEMS Microbiol. Lett. 2008;285:212–219.10.1111/fml.2008.285.issue-2
- Sakamoto N, Tsuyuki R, Yoshinari T, et al. Correlation of ATP citrate lyase and acetyl CoA levels with trichothecene production in Fusarium graminearum. Toxins. 2013;5:2258–2269.10.3390/toxins5112258
- Unnithan GC, Nair KK, Bowers WS. Precocene-induced degeneration of the corpus allatum of adult females of the bug Oncopeltus fasciatus. J. Insect Physiol. 1977;23:1081–1094.10.1016/0022-1910(77)90137-8
- Piulachs MD, Cassier P, Bellés X. Ultrastructural changes induced by precocene II and 3,4-dihydroprecocene II in the corpora allata of Blattella germanica. Cell Tissue Res. 1989;258:91–99.
- Hammond AH, Garle MJ, Fry JR. Mechanism of toxicity of precocene II in rat hepatocyte cultures. J. Biochem. Toxicol. 1995;10:265–273.10.1002/(ISSN)1522-7146
- Furukawa T, Sakamoto N, Suzuki M, et al. Precocene II, a trichothecene production inhibitor, binds to voltage-dependent anion channel and increases the superoxide level in mitochondria of Fusarium graminearum. PLOS ONE. 2015;10:e0135031.10.1371/journal.pone.0135031
- Blachly-Dyson E, Forte M. VDAC channels. IUBMB Life. 2001;52:113–118.
- Han D, Antunes F, Canali R, et al. Voltage-dependent anion channels control the release of the superoxide anion from mitochondria to cytosol. J. Biol. Chem. 2003;278:5557–5563.10.1074/jbc.M210269200
- Lustgarten MS, Bhattacharya A, Muller FL, et al. Complex I generated, mitochondrial matrix-directed superoxide is released from the mitochondria through voltage dependent anion channels. Biochem. Biophys. Res. Commun. 2012;422:515–521.10.1016/j.bbrc.2012.05.055
- Ponts N, Pinson-Gadais L, Verdal-Bonnin MN, et al. Accumulation of deoxynivalenol and its 15-acetylated form is significantly modulated by oxidative stress in liquid cultures of Fusarium graminearum. FEMS Microbiol. Lett. 2006;258:102–107.10.1111/fml.2006.258.issue-1