Abstract
A marine acidophilic sulfur-oxidizing bacterium, Acidithiobacillus thiooxidans strain SH, was isolated to develop a bioleaching process for NaCl-containing sulfide minerals. Because the sulfur moiety of sulfide minerals is metabolized to sulfate via thiosulfate as an intermediate, we purified and characterized the thiosulfate dehydrogenase (TSD) from strain SH. The enzyme had an apparent molecular mass of 44 kDa and was purified 71-fold from the solubilized membrane fraction. Tetrathionate was the product of the TSD-oxidized thiosulfate and ferricyanide or ubiquinone was the electron acceptor. Maximum enzyme activity was observed at pH 4.0, 40 °C, and 200 mM NaCl. To our knowledge, this is the first report of NaCl-stimulated TSD activity. TSD was structurally different from the previously reported thiosulfate-oxidizing enzymes. In addition, TSD activity was strongly inhibited by 2-heptyl-4-hydroxy-quinoline N-oxide, suggesting that the TSD is a novel thiosulfate:quinone reductase.
Graphical abstract
Tetrathionate-forming thiosulfate dehydrogenase purified from At. thiooxidans SH showed the maximum enzyme activity at pH 4.0, 40°C, and 200 mM NaCl.
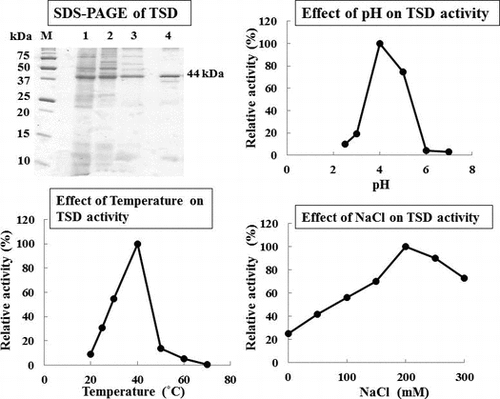
Acidophilic chemoautotrophic iron- or sulfur-oxidizing bacteria, such as Acidithiobacillus ferrooxidans and Acidithiobacillus thiooxidans, are used for the bioleaching of sulfide minerals. NaCl is a crucial factor in this process, as it inhibits the activities and growth of bioleaching micro-organisms.Citation1) Because ocean deposits are rich in pyrite, chalcopyrite, and marcasite, marine micro-organisms show advantages in the bioleaching of marine sulfide minerals. We isolated an acidophilic marine sulfur-oxidizing bacterium—At. thiooxidans strain SH—from Seto Inland Sea, Japan, to develop a bioleaching process for NaCl-containing sulfide minerals.Citation2,3) The bacterium grows chemoautotrophically by deriving energy from the oxidation of elemental sulfur and reduced inorganic sulfur compounds (RISCs) such as thiosulfate and tetrathionate.
Thiosulfate-metabolizing activity is most important for bioleaching, as the sulfur moiety of sulfide minerals is thought to be metabolized via thiosulfate as an intermediate.Citation4) At the genomic level, three draft genome sequences of At. thiooxidans have been released including those from ATCC 19377,Citation5) Licanantay,Citation6) and A01.Citation7) Based on the documented models in other Acidithiobacillus species, such as At. ferrooxidans and At. caldus, hypothetical models for sulfur oxidation in At. thiooxidans have been proposed.Citation5,7) In these models, extracellular elemental sulfur is activated to form thiol-bound sulfane sulfur atoms and is then transferred to the periplasm. Thiol-bound sulfane sulfur atoms are oxidized by a sulfur dioxygenase to produce sulfite, which reacts with the sulfur atom to produce thiosulfate without enzymatic catalysis.Citation4,8) Because a gene homologous to doxDA encoding a thiosulfate, quinone oxidoreductase (TQO)Citation9) was detected in the At. thiooxidans genome, thiosulfate is thought to be catalyzed by TQO to generate tetrathionate in the periplasm.Citation5,7) Alternatively, because two incomplete sulfur oxidizing (SOX) systems were found in the genome of At. thiooxidans, thiosulfate may be catalyzed by the SOX system to generate sulfate and elemental sulfur as described for the thiosulfate-oxidizing system in Allochromatium vinosum.Citation10)
At least four enzymes associated with thiosulfate oxidation have been identified in micro-organisms.Citation11) Among these, comparatively little is known about the enzymes catalyzing the oxidative condensation of two molecules of thiosulfate to tetrathionate. As described above, TQO from the archaeon Acidianus ambivalens has been characterized at the molecular genetic level (Supplemental Table 1).Citation9) Homologous genes have been identified in At. thiooxidans, Citation5–7) At. ferrooxidans,Citation12) and At. caldusCitation13) genomes. The other thiosulfate dehydrogenase characterized at the molecular genetic level is TsdA from the purple sulfur bacterium Al. vinosum.Citation10) Database searches have revealed the presence of tsdA-related genes in several different proteobacteria, such as Rhodopseudomonas palustris, Pseudomonas fluorescens, Thiobacillus denitrificans, and Thiomonas intermedia; however, tsdA-related genes were not found in the genomes of At. thiooxidans, At. caldus, and At. ferrooxidans.Citation14) We also characterized the tetrathionate-forming thiosulfate dehydrogenase (TSD) from At. ferrooxidans ATCC 23270 at the molecular genetic level.Citation11) Although database searches revealed the occurrence of homologous proteins in other bacteria, such as Acidiphilium multivorum, Thiomonas intermedis, Hyphomicrobium sp., Bulkholderia cenocepacia, and Hydrogenobaculum sp., homologous proteins, the occurrence was not found in At. caldus and At. thiooxidans.Citation11) These results indicate that At. caldus and At. thiooxidans use different tetrathionate-forming thiosulfate dehydrogenases from TSD in At. ferrooxidans or Al. vinosum. Although thiosulfate dehydrogenase containing c-type heme has been purified from At. thiooxidans JCM 7814 (Supplemental Table 1), the assignment of a gene to this enzyme has not been achieved.Citation15) In this report, we describe the purification and characterization of a novel tetrathionate-forming TSD from At. thiooxidans strain SH.
Materials and methods
Bacterial strains, media, and growth conditions
Acidithiobacillus thiooxidans strain SH (NBRC 101132) was grown on minimal salts medium (pH 5.0) containing 10 mM Na2S2O3 and 0.35 M NaCl . The mineral salts medium was composed of the following basal salts (in g/L): (NH4)2SO4 (3.0), Na2SO4·10H2O (3.2), KCl (0.1), K2HPO4 (0.05), MgSO4·7H2O (0.5), FeSO4 (0.02), and Ca(NO3)2 (0.01). One milliliter of the following trace element solution (in mg/L) was added to the medium: FeCl3·6H2O (11.0), CuSO4·5H2O (0.5), HBO3 (2.0), MnSO4·H2O (2.0), Na2MoO4·2H2O (0.8), CoCl2·6H2O (0.6), and ZnSO4·7H2O (0.9). Cells were grown with continuous aeration at 30 °C.
Enzyme assay
TSD activity was measured by monitoring the reduction of ferricyanide as an electron acceptor. The reaction mixture contained 50 mM citrate buffer (pH 4.0), 1 mM K-ferricyanide, 10 mM Na-thiosulfate, 200 mM NaCl, and the enzyme preparation. The reaction was initiated by adding thiosulfate at 40 °C. The reduction of ferricyanide was monitored by measuring the absorbance of the reaction mixture at 420 nm (1.02 mM−1·cm−1). One unit of activity (U) is defined as 1 μmol ferricyanide reduced per minute. TQO activity was also measured by monitoring the decrease in absorbance at 275 nm (12.25 mM−1·cm−1) due to the reduction of ubiquinone. The reaction mixture contained 50 mM citrate buffer (pH 4.0), 30 μM ubiquinone-2 (Eizai Co., Tokyo, Japan), 10 mM Na-thiosulfate, 200 mM NaCl, and the enzyme preparation. Thiosulfate:cytochrome c oxidoreductase activity was measured as the increase in absorbance at 550 nm. The reaction mixture contained 50 mM citrate buffer (pH 4.0), 0.1 mg/mL of an oxidized horse-heart cytochrome c, 10 mM Na-thiosulfate, 200 mM NaCl, and the enzyme preparation. All reaction rates were corrected for the non-enzymatic reaction by heat-inactivated enzyme (10 min at 100 °C). All measurements were performed in triplicate. Each data point is shown as the arithmetic mean value.
Purification of TSD from At. thiooxidans strain SH
After 7 days of cultivation in basal salts medium supplemented with thiosulfate and NaCl, cells were harvested by centrifugation at 6000×g for 10 min. The cells were washed 3 times with 0.1 M K-phosphate buffer (pH 6.3) and disrupted by three passages through a French press cell at 1500 kg/cm2, followed by sonication on ice (Ultrasonic homogenizer VP-300; Taitec, Tokyo, Japan; 23% intensity cycles of 30 s on, 60 s off for a total of 30 min). Unbroken cells and cellular debris were removed by centrifugation at 10,000×g for 10 min. The resulting supernatant (cell-free extract) was further centrifuged at 110,000×g for 60 min to prepare the membrane and soluble (cytoplasmic and periplasmic) fractions. Since TSD activity was detected in the membrane fraction, the membrane fraction was treated with n-dodecyl-β-d-maltopyranoside (DM) at a concentration of 3 mg of DM per mg of protein at 4°C overnight with stirring. The resulting suspension was centrifuged at 110,000×g for 60 min to obtain the solubilized membrane fraction. The fraction was dialyzed to 20 mM Bis-Tris buffer (pH 6.4) before loading on an anion-exchange column chromatography using a Q-Sepharose (Tosoh, Tokyo, Japan) equilibrated with the same buffer. TSD activity was detected in the unadsorbed fraction. The fraction was dialyzed against 20 mM citrate buffer (pH 4.2), concentrated using a Vivapore 10/20 (Sartorius Stedim Biotech, Germany), and applied to a prepacked TSKgel G3000 column (Tosoh) equilibrated with 20 mM citrate buffer (pH 4.2). Fractions showing TSD activity were pooled and stored at −20 °C. All chromatography steps were carried out using an ÄKTAprime plus chromatography system (GE Healthcare, Little Chalfont, UK).
Protein analysis
SDS-polyacrylamide gel electrophoresis (PAGE) was performed in 12.5% (w/v) polyacrylamide gel with Tris-glycine buffer. Proteins were detected by Coomassie Brilliant Blue staining. The apparent molecular mass of the native enzyme was estimated by gel permeation chromatography as described above. Aldolase (158 kDa), albumin (67 kDa), ovalbumin (43 kDa), chymotrypsinogen A (25 kDa), myoglobin (17 kDa), and ribonuclease A (13.7 kDa) were used as molecular mass standards. To determine the N-terminal amino acid sequence, proteins separated by SDS-PAGE were electroblotted onto a polyvinylidene difluoride membrane (Hybond-P, GE Healthcare) using a blotting apparatus (Semidry Blot AE-6677, Atto Co., Tokyo, Japan). The N-terminal amino acid sequence was analyzed by Edman sequencing analysis using an automatic protein sequencer (Model 270, PE Biosystems, Waltham, MA, USA). Alternatively, the Coomassie Brilliant Blue-stained protein band was excised from gel and subjected to the in-gel trypsin digestion. After extraction of the peptide using an In-Gel Tryptic Digestion Kit (Pierce, Rockford, IL, USA), the peptide solution was analyzed by HPLC-Chip/QTOF (G6520 and G4240, Agilent Technologies, Santa Clara, CA, USA). The results obtained were analyzed using the MASCOT software (Matrix Science Inc., Boston, MA, USA).
The spectra of the oxidized and reduced enzyme were recorded using a UV/visible spectrophotometer (V-530, JASCO Co., Tokyo, Japan). The reduced enzyme was prepared by adding dithionite to the enzyme solution.
Analysis of sulfur compounds
Thiosulfate and tetrathionate were analyzed by an ion chromatographic method developed by Miura et al.Citation16) Briefly, thiosulfate oxidation by TSD was carried out at pH 4.0 and 40°C in a reaction mixture containing 1 mM thiosulfate, 1 mM ferricyanide, 200 mM NaCl, and 5 μg enzyme. β-Alanine buffer (pH 4.0) was used rather than citrate buffer (pH 4.0), as citrate buffer interferes with the detection of thiosulfate by HPLC analysis. Thirty minutes after the addition of thiosulfate to the reaction mixture, 100 μL of the reaction mixture was used to analyze the reaction products. An ion chromatographic system composed of a Model LC-20A pump (Shimadzu, Kyoto, Japan), a Model SPD-20AV photometric detector (Shimadzu), and a Sunfire C18 separating column (Waters, Co., Milford, MA, USA) was used for analyses. An acetonitrile–water (20:80, v/v) solution containing 6 mM tetrapropylammonium hydroxide was used as the mobile phase.
Results and discussion
Detection of TSD activity in thiosulfate-grown At. thiooxidans strain SH
The presence of TSD activity in the cell-free extracts of At. thiooxidans strain SH cells aerobically grown on thiosulfate was analyzed. When the TSD activity was measured in a reaction mixture containing a cell-free extract, ferricyanide, and thiosulfate, low activities were detected within the pH range of 1.5–5.0 at 40 °C by subtracting the non-enzymatic values (obtained by using the heat-inactivated enzyme). The addition of NaCl at the concentration up to 300 mM to the reaction mixture activated the TSD activity. The highest TSD activity in the cell-free extract was obtained in the reaction mixture at 200 mM NaCl. Since our previous research on the TSD from At. ferrooxidans ATCC 23270 demonstrated the requirement of sulfate ion for enzyme activity,Citation11) the effect of 100 mM Na2SO4 on enzyme activity was examined. However, Na2SO4 did not have an activating effect on TSD from strain SH. To our knowledge, there are no reports of NaCl-stimulated TSD activity. The activities of TSD in a membrane fraction and a soluble fraction prepared from cell-free extract were analyzed to determine the localization of TSD. The TSD activity (0.76 U/mg) was detected in the membrane fraction (Table ). No activity was detected in the soluble fraction. Although TQO in Ac. ambivalens has been reported to be localized in the membrane,Citation9) TSDs in the other bacteria, such as Thiobacillus acidophilus,Citation17) Thiobacillus sp.W5,Citation18) Al. vinosum,Citation10) At. thiooxidans JCM7814,Citation15) and At. ferrooxidans,Citation11,19) have been purified from the soluble fraction (Supplemental Table 1). These results suggest that At. thiooxidans strain SH possesses an unique TSD for thiosulfate metabolism.
Table 1. Purification of thiosulfate dehydrogenase from the membrane fraction of thiosulfate-grown At. thiooxidans strain SH.
Purification of TSD from thiosulfate-grown At. thiooxidans strain SH
The enzyme was successfully solubilized from the membrane fraction with DM and purified from the solubilized membrane fraction in a two-step chromatographic procedure using a Q-Sepharose anion-exchange fast flow column and gel filtration chromatography. Approximately 71-fold enrichment with a recovery of 4.3% of the total activity was obtained from the cell-free extract (Table ). SDS-PAGE analysis of the final preparation showed a major band with an apparent molecular mass of 44 kDa (Fig. ). The apparent molecular mass of the native enzyme was calculated to be 99 kDa by gel permeation chromatography (TSK gel G3000) (data not shown), suggesting that TSD in strain SH is a homodimer.
Fig. 1. SDS-PAGE analysis of partially purified thiosulfate dehydrogenase. Proteins were stained with Coomassie Brilliant Blue. Lane M, molecular markers; lane 1, membrane fraction; lane 2, DM-solubilized fraction; lane 3, Q-Sepharose; and lane 4, Gel filtration.
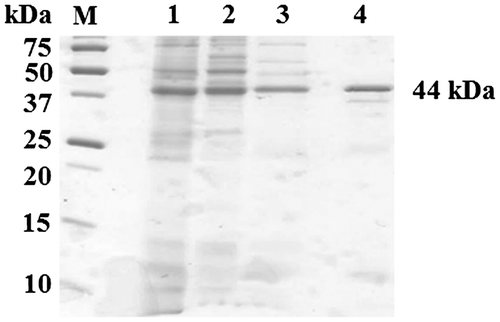
Some thiosulfate dehydrogenases catalyzing the oxidation of thiosulfate to tetrathionate have been reported to contain a c-type heme molecule in their proteins (Supplemental Table 1).Citation10,15,17,18) UV–visible absorption spectra of the TSD showed absorption peaks for the reduced form at 275, 340, and 415 nm (Supplemental Fig. 2). The absence of heme groups in the protein was suggested because distinct absorption peaks at approximately 550 nm were not detected. TQO from Ac. ambivalens, which catalyzes the conversion of thiosulfate to tetrathionate using quinone as an electron acceptor, involves a mixture of caldariella quinone, sulfolobus quinone, and menaquinone non-covalently bound to the protein.Citation9) Involvement of quinone in the TSD from strain SH was not clearly revealed because the amount of the final preparation was very low. This should be evaluated in future studies.
Biochemical properties of TSD from strain SH
Maximum enzyme activity was observed at pH 4.0 (Fig. (A)) and a temperature of 40°C (Fig. (B)). Since the TSD activity in cell-free extract was activated in the reaction mixture supplemented with NaCl, the effect of NaCl on purified TSD was analyzed. TSD showed the maximum activity at the same concentration of NaCl (200 mM) as observed in the cell-free extract (Fig. (C)). The effect of several other ions on TSD activity was examined. The activity was stimulated by KCl and MgCl2, although the stimulatory effects of these compounds were lower than those of NaCl. A slight stimulatory effect on the activity was observed with MgSO4, while Na2SO4 at a concentration of 100 mM showed no stimulation effect on the activity (Table ). The effect of Na2SO4 on TSD activity in the reaction mixture containing 200 mM NaCl was tested. The TSD activity was inhibited by increasing the concentrations of Na2SO4 (Table ). These results suggested that chloride ion (Cl−) and magnesium ion (Mg2+) stimulated the TSD activity. Sodium ion (Na+) and sulfate ion (SO42−) appeared to have no effect on TSD activity. High concentrations of Na+ inhibited enzyme activity (Table ).
Fig. 2. Effect of pH (A), temperature (B), NaCl (C), or thiosulfate (D) on thiosulfate dehydrogenase activity.
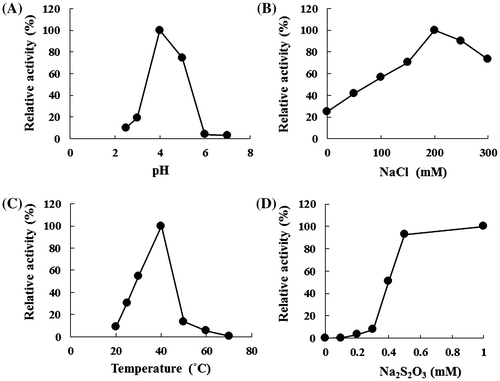
Table 2. Effect of chemicals on thiosulfate dehydrogenase activity.
Table 3. Inhibition of thiosulfate dehydrogenase activity by various chemicals.
Our previous study revealed that NaCl at an optimum concentration of 0.35 M stimulated the growth of strain SH on elemental sulfur, and that LiCl and KCl could not sustain the growth.Citation2) When the thiosulfate-oxidizing activity in strain SH grown on sulfur was measured by the consumption of O2, NaCl did not affect the activity. Although thiosulfate dehydrogenase activity at pH 4.0 was also detected in a cell-free extract of strain SH grown on sulfur, the activity was not affected by NaCl.Citation3) The results obtained in the present study contradict the results of previous studies. Although the source of this contradiction remains unclear, the difference in the substrate used for growth may explain the results. Since thiosulfate is thought to be generated as one of the intermediates in sulfur metabolism in At. thiooxidans,Citation4,8) a thiosulfate metabolizing enzyme is required for the sulfur-grown cells to metabolize the resulting thiosulfate. In the present study, because thiosulfate was used as the substrate for growth, a thiosulfate-metabolizing enzyme must catalyze the first catabolic step of thiosulfate. Although additional studies are required to clarify these issues, strain SH grown on sulfur may use a different thiosulfate-metabolizing system. It has been proposed that an incomplete SOX system similar to the system in Al. vinosumCitation10) is involved in thiosulfate metabolism in At. thiooxidans grown on sulfur,Citation20) suggesting that the enzyme system, which does not require NaCl to activate enzyme activity, is mainly used in sulfur-grown cells. To confirm this hypothesis, tsd and sox gene expressions should be examined in the cells grown on sulfur, thiosulfate, or tetrathionate.
The kinetic parameters of thiosulfate dehydrogenase were determined by examining the effect of thiosulfate concentration in the reaction mixture. The relationship between substrate concentration and reaction rate was represented by a sigmoidal curve, which showed similar characteristics to an allosteric enzyme. The apparent Km was estimated to be 0.4 mM. (Fig. (D)). The Km value showed a similar level of substrate specificity to the substrate specificity of other thiosulfate-oxidizing enzymes (Supplemental Table 1).
Stoichiometry of thiosulfate oxidation
The end-products of thiosulfate oxidation in the presence of ferricyanide as the artificial electron acceptor by TSD were analyzed by HPLC. HPLC analyses revealed a new peak, which showed the same retention time and shape as tetrathionate, which was used as a standard (Supplemental Fig. 3). The analysis revealed the conversion of 1 mM thiosulfate to 0.437 mM tetrathionate, indicating that TSD catalyzed the conversion of two molecules of thiosulfate to one molecule of tetrathionate.
Substrate specificity and electron acceptors
TSD activity was evaluated using Na-sulfite and Na-tetrathionate as the substrates at concentrations of 10 mM. Reduction of ferricyanide was not observed when these sulfur compounds were used as substrates. Sulfide was not tested because it chemically reduces ferricyanide. Since cytochrome c or ubiquinone is thought to be the electron acceptor in vivo, TSD activity was measured using a ubiquinone (Q2) or a horse heart cytochrome c as an electron acceptor. Horse heart cytochrome c was not used as an electron acceptor, whereas ubiquinone (Q2) was used as the electron acceptor with the specific activity of 7.38 mM (min/ mg). These results suggest that the native electron acceptor of TSD from strain SH is ubiquinone.
Inhibitors
Sulfite has been reported to be a strong inhibitor of thiosulfate dehydrogenase activities from Al. vinosum, T. acidophilus, and Thiobacillus sp. W5 (Supplemental Table 1).Citation10,17,18) TQO activity from Ac. ambivalens is also strongly inhibited by sulfite.Citation9) At micromolar concentrations, sulfite was a potent inhibitor of TSD activity from strain SH (Table ). Although N-ethylmaleimide, which reacts with cysteine residues, moderately inhibits TQO activity from Ac. ambivalens (54% inhibition at 1 mM),Citation9) it had no effect on TSD activities from T. acidophilus and Thiobacillus sp W5.Citation17,18) TSD activity from strain SH was reduced to 84% at 1 mM, suggesting the absence of cysteine residues in the catalytic site of TSD. 2-heptyl-4-hydroquinoline N-oxide, which inhibits the activity of enzymes using quinone as the coenzyme for the reaction, reduced the TSD activity to 12% at 1 μM (Table ). Since the TSD from strain SH was able to use Q2 as an electron acceptor, this result suggests that TSD from strain SH has TQO activity. Therefore, further detailed investigations using ubiquinone are necessary to clarify the properties of this enzyme and will be the subject of a future communication.
Identification of the gene encoding TSD
Three draft genome sequences of At. thiooxidans strains are now available. We hypothesized that a homologous protein with TSD in strain SH could be found in the database; thus, we initially attempted to determine the gene from the N-terminal amino acid sequence of the 44-kDa protein. However, the amino acid sequence was not determined by the Edman sequencing analysis. Therefore, we analyzed peptide fragments produced from the in-gel trypsin digestion using HPLC-Chip/QTOF. Amino acid sequences for ten peptide fragments of the 44-kDa protein were determined (Supplemental Table 2). Analysis using MASCOT software revealed no protein with a high score and coverage in the databases, indicating that a homologous protein to the 44-kDa protein is not present in other At. thiooxidans strains whose genome sequences have been determined.
Recently, a comparative genomic analysis of three At. thiooxidans strains revealed that the At. thiooxidans Licanantay genome contains additional elements that may be associated with adaptation to the environment, although there is a large core of genes shared by all species strains.Citation6) Since several of the genes have been identified in a unique genomic region, the authors suggested that they are a part of genomic islands acquired by horizontal transfer. Similarly, we hypothesized that strain SH acquires a unique gene encoding the 44-kDa protein by horizontal transfer, as the protein with a high score and coverage was not found in other At. thiooxidans genomes. To evaluate this hypothesis, the whole genome sequence analysis of strain SH is currently underway.
Conclusion
Tetrathionate-forming TSD was purified and characterized for the first time from the solubilized membrane fraction of a marine sulfur-oxidizing bacterium, At. thiooxidans strain SH. Comparison with thiosulfate metabolizing enzymes from other micro-organisms showed that TSD from strain SH was structurally different from those of previously reported thiosulfate-oxidizing enzymes. To our knowledge, this is the first report of thiosulfate dehydrogenase whose activity is stimulated by NaCl. TSD from strain SH was able to use ubiquinone (Q2) as the electron acceptor, suggesting that TSD had TQO activity. TQO from Ac. ambivalens is the only TQO consisting of two subunits (DoxDA) and that has been characterized at the molecular genetic level.Citation9) Although further detailed investigations are necessary to clarify the properties of TSD from strain SH, the enzyme may be a novel TQO.
Authors contributions
T.K. and K.K. designed the research. S.S. and E.Y. performed the experiments. T.K. and K.K. supervised all the enzyme experiments and analyzed the data. S.S drafted the manuscript. K.K. carried out the critical revision of the manuscript for important intellectual content. All authors have read and approved the final manuscript.
Disclosure statement
No potential conflict of interest was reported by the authors.
Supplemental material
The supplemental material for this paper is available at http://dx.doi.org/10.1080/09168451.2015.1088377.
Funding
This work was supported by Ministry of Education, Culture, Sports, Science and Technology, Japan.
Acknowledgments
We are grateful to Dr. Tsugumi Shiokawa (Advanced Science Research Center, Okayama University) for her assistance in analyzing the N-terminal amino acid sequence and peptide fragments produced by the in-gel trypsin digestion of the 44-kDa protein.
SupplementaryFig.__Table.docx
Download MS Word (397.1 KB)References
- Wang Y, Su L, Zhang L, et al. Bioleaching of chalcopyrite by defined mixed moderately thermophilic consortium including a marine acidophilic halotolerant bacterium. Bioresour. Technol. 2012;121:348–354.
- Kamimura K, Higashino E, Moriya S, et al. Marine acidophilic sulfur-oxidizing bacterium requiring salts for the oxidation of reduced inorganic sulfur compounds. Extremophiles. 2003;7:95–99.
- Kamimura K, Higashino E, Kanao T, et al. Effects of inhibitors and NaCl on the oxidation of reduced inorganic sulfur compounds by a marine acidophilic, sulfur-oxidizing bacterium, Acidithiobacillus thiooxidans strain SH. Extremophiles. 2005;9:45–51.
- Rohwerder T, Sand W. The sulfane sulfur of persulfides is the actual substrate of the sulfur-oxidizing enzymes from Acidithiobacillus and Acidiphilium spp. Microbiology. 2003;149:1699–1710.
- Valdes J, Ossandon F, Quatrini R, et al. Draft genome sequence of the extremely acidophilic biomining bacterium Acidithiobacillus thiooxidans ATCC 19377 provides insight into the evolution of the Acidithiobacillus genus. J. Bacteriol. 2011;193:7003–7004.
- Travisany D, Cortés MP, Latorre M, et al. A new genome of Acidithiobacillus thiooxidans provides insights into adaptation to a bioleaching environment. Res. Microbiol. 2014;165:743–752.
- Yin H, Zhang X, Li X, et al. Whole-genome sequencing reveals novel insights into sulfur oxidation in the extremophile Acidithiobacillus thiooxidans. MBC Microbiol. 2014;14:179–193.
- Suzuki I. Oxidation of inorganic sulfur compounds: chemical and enzymatic reactions. Can. J. Microbiol. 1999;45:97–105.
- Müller FH, Bandeiras TM, Urich T, et al. Coupling of the pathway of sulphur oxidation to dioxygen reduction: characterization of a novel membrane-bound thiosulphate:quinone oxidoreductase. Mol. Microbiol. 2004;53:1147–1160.
- Hensen D, Sperling D, Trüper HG, et al. Thiosulphate oxidation in the phototrophic sulphur bacterium Allochromatium vinosum. Mol. Microbiol. 2006;62:794–810.
- Kikumoto M, Nogami S, Kanao T, et al. Tetrathionate-forming thiosulfate dehydrogenase from the acidophilic, chemolithoautotrophic bacterium Acidithiobacillus ferrooxidans. Appl. Environ. Microbiol. 2013;79:113–120.
- Valdés J, Pedroso I, Quatrini R, et al. Acidithiobacillus ferrooxidans metabolism: from genome sequence to industrial applications. BMC Genomics. 2008;9:597.
- Mangold S, Valdés J, Holmes DS, et al. Sulfur metabolism in the extreme acidophile Acidithiobacillus caldus. Front Microbiol. 2011;2:17.
- Denkmann K, Grein F, Zigann R, et al. Thiosulfate dehydrogenase: a widespread unusual acidophilic c-type cytochrome. Environ. Microbiol. 2012;14:2673–2688.
- Nakamura K, Nakamura M, Yoshikawa H, et al. Purification and properties of thiosulfate dehydrogenase from Acidithiobacillus thiooxidans JCM7814. Biosci. Biotechnol. Biochem. 2001;65:102–108.
- Miura Y, Kawaoi A. Determination of thiosulfate, thiocyanate and polythionates in a mixture by ion-pair chromatography with ultraviolet absorbance detection. J. Chromatogr. A. 2000;884:81–87.
- Meulenberg R, Pronk JT, Hazeu W, et al. Purification and partial characterization of thiosulphate dehydrogenase from Thiobacillus acidophilus. J. Gen. Microbiol. 1993;139:2033–2039.
- Visser JM, de Jong GAH, Robertson LA, et al. Purification and characterization of a periplasmic thiosulfate dehydrogenase from the obligately autotrophic Thiobacillus sp. W5. Arch. Microbiol. 1997;166:372– 378.
- Janiczek O, Zemanova J, Mandl M. Purification and some properties of thiosulfate dehydrogenase from Acidithiobacillus ferrooxidans. Prep. Biochem. Biotechnol. 2007;37:101–111.
- Bobadilla Fazzini RA, Cortés MP, Padilla L, et al. Stoichiometric modeling of oxidation of reduced inorganic sulfur compounds (Riscs) in Acidithiobacillus thiooxidans. Biotechnol. Bioeng. 2013;110:2242–2251.