Abstract
Administration of macromolecule compositions in medicine and cosmetics always exhibited low bioavailability due to the limitation of transmembrane transport. Here, human epidermal growth factor (hEGF) was fused with glutathione S-transferase (GST) and Pep-1, the first commercial cell-penetrating peptide, in Escherichia coli. The fusion protein was firstly purified with the affinity chromatography, and then the GST tag was released by TEV protease. Final purification was achieved by the ion exchange chromatography. The biological activities and the transmembrane ability of the obtained products were determined using scratch wound-healing assay, MTT analysis, and immunofluorescence assay. The results showed that both rhEGF and Pep-1-fused hEGF were soluble expressed in E. coli. The fusion of Pep-1 could markedly increase the transmembrane ability of EGF, whereas it did not interfere with the growth-stimulating and migration-promoting functions of hEGF on fibroblasts. This research provided a novel strategy for the transmembrane transport of protein-derived cosmetics or drugs.
Graphical abstract
Fusion with pep-1, a cell-penetrating peptide, enhances the transmembrane ability of human EGF.
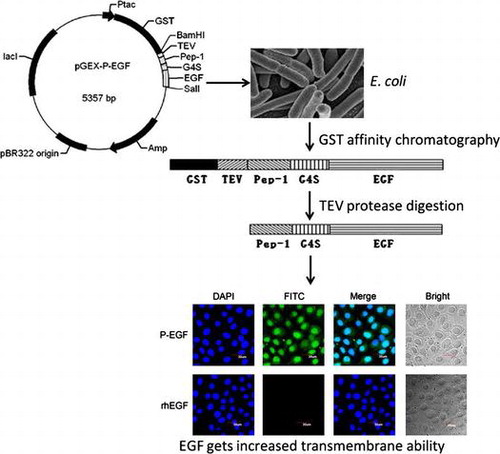
Key words:
The cell membrane is a barrier that completely blocks passive diffusion of hydrophilic macromolecules. Thereby, administration of macromolecule compositions (such as polypeptides, proteins, DNA, RNA) in medicine always exhibited low bioavailability due to the limitation of transmembrane transport. In addition, many peptides and proteins, such as epidermal growth factor (EGF), superoxide dismutase (SOD), collagen, have also been applied in the cosmetics to alter the aging process.Citation1,2) However, because of the skin cells which functions as barriers to prevent penetration of most foreign large molecules, delivery of peptides or proteins into skin is a major challenge.Citation3,4)
Human EGF (hEGF) is a 6.2 kDa single-chain polypeptide consisting of 53 amino acid residues and three intramolecular disulfide bonds. It can promote the proliferation and differentiation of fibroblast cells, stimulate the synthesis of collagen, and inhibit the secretion of gastric acid. Therefore, hEGF has significant functions in healing of skin wound and oral/gastroesophageal ulcers, removing wrinkle, keeping skin moist, soft and elastic, and delaying skin aging.Citation5–8) Besides being used as a medicine, hEGF has also been widely used in cosmetics industry. However, in fact, previous studies showed that the absorption of hEGF is very low, suggesting that more efficient permeation strategies should be developed in the hEGF-contained drugs and cosmetics.Citation9,10)
Cell-penetrating peptides (CPPs), also known as protein transduction domains (PTDs) or membrane transduction peptides,Citation11) are short peptides (≤40 amino acids) with ability to penetrate cell membranes and translocate different cargo proteins or peptides into cells.Citation12) Increasing evidence has indicated that a variety of macromolecules, including of nucleic acids, polypeptides, and synthetic drugs, can obtain effective intracellular delivery when they were co-administrated with CPPs.Citation13) Among the CPPs, Pep-1 is a synthetic amphipathic peptide consisted of the following domains from its N-terminus: a hydrophobic tryptophan-rich motif (KETWWETWWTEW), a spacer (SQP), and a hydrophilic lysine-rich domain (KKKRKV). In this study, to improve the transmembrane ability of hEGF, Pep-1 was fused to the N-terminus of hEGF and expressed in Escherichia coli. The recombinant fusion protein was then purified, and its biological activity, and transmembrane ability were also analyzed.
Materials and methods
Bacterial strains, plasmids, and cells
E. coli DH5α was used as the host for subcloning and plasmid amplification. E. coli BL21 (DE3) was used as the expression host. The cDNA of MDA-MB-231 human breast cancer cells was used as templates for the cloning of hEGF gene. Plasmid pGEX-6P-3 was used as the expression vector. The monkey fibroblast COS-7 were cultured in F12 medium added with 10% (v/v) fetal bovine serum at 37 °C in a humidified atmosphere containing 5% CO2.
Construction of the recombinant plasmids
To construct the expression plasmid for the Pep-1-fused hEGF (P-EGF), firstly, the gene of TEV protease recognition site, Pep-1, and flexible linker (G4S) was amplified and connected with overlapping polymerase chain reaction (PCR) using primer P1, P2, and P3. The hEGF gene was amplified from cDNA of MDA-MB-231 cells using primer P4 and P5. The products of these two PCR amplifications were then mixed to use as a new template and amplified with primer P1 and P5 to generate the coding sequence of P-EGF. The obtained PCR product was digested with BamHI and SalI and inserted into pGEX-6P-3 plasmid. The recombinant construction was analyzed by restriction-enzyme digestion and sequencing to confirm sequence fidelity, and the positive recombinant plasmid was named as pGEX-P-EGF. In addition, the expression plasmid for hEGF alone (rhEGF) was also constructed. Briefly, the gene for rhEGF fused with TEV protease recognition site was amplified from pGEX-P-EGF plasmid with primer P6 and P5, and then inserted into pGEX-6P-3 vector to generate the recombinant plasmid pGEX-rhEGF. All the primers used in this study were shown in the supplementary Table S1. The schematic representation of the construction was illustrated in Fig. S1.
Expression and purification of recombinant proteins
The recombinant plasmids were transformed into E. coli BL21 (DE3) for expression. A single colony containing the recombinant plasmid was cultured in medium containing 50 μg/ml ampicillin at 37 °C. When the cultures reached an OD600 of 0.6, isopropyl-beta-D-thiogalactopyranoside (IPTG) was added to the broth for induction of the recombinant protein. After the induction, the bacterial cells were lysed by sonication in phosphate buffer and then the supernatant obtained after the centrifugation (13,000×g, 30 min) was collected as the soluble protein sample. In order to find optimal conditions for the soluble expression of proteins, effects of culture medium, temperature, rotational speeds, IPTG concentrations, and induction times on the yield of recombinant products were tested. The proportion of fusion protein P-EGF in total protein was analyzed using BCA assay and quantified with QuantityOne software (Bio-Rad, Inc., Hercules, California, USA). Then, the target protein was separated using the affinity chromatography with glutathione resins, and then the purified GST-fused protein was specifically digested with TEV protease at 4 °C overnight to cleave the GST tag. Finally, the ion exchange chromatography (HiTrap Q HP, GE Healthcare, USA) was performed to purify the P-EGF or rhEGF from the cleaved protein solution. The protein was detected by sodium dodecyl sulfate-polyacrylamide gel electrophoresis (SDS–PAGE).
Western blot analysis
Equal amount of total protein was loaded on SDS–PAGE. Then samples were transferred to nitrocellulose membranes and blocked for 60 min at room temperature in 5% skim milk powder (wt/vol). The membranes were immunoblotted with mouse anti-hEGF (1:250, Santa Cruz, USA) antibodies overnight at 4 °C, and then incubated with IRDyeTM-800 conjugated anti-mouse secondary antibodies (Li-COR Biosciences, USA) for 30 min at room temperature. The specific proteins were visualized by Odyssey Infrared Imaging System (Gene Company, China).
Cell migration assay
The COS-7 cells were grown to 95% confluent in 24-well plates. The cell monolayer was then scratched with a sterile pipette tip to generate a wound. The remaining cells were washed twice with warm culture medium to remove cell debris, and then treated with rhEGF, P-EGF, or the commercial hEGF agent. The width (W) of scratch was photographed using an inverted phase contrast microscope (Olympus, Japan) and measured using Hprsnap6 software. The migration rate was calculated and expressed as fold compared to the control group.
MTT assay
In brief, COS-7 cells were prepared in basal media at a density of 2 × 104 cells/mL and seeded 100 μL/well on sterile 96-well tissue culture plate. After incubation for 24 h, cells were treated with rhEGF or P-EGF for 72 h, and then methylthiazolyldiphenyl-tetrazolium bromide (MTT) (5 mg/mL) was added to the wells (20 μL/well). The plates were incubated in the cell incubator for an additional 4 h, then the supernatant was removed and 100 μL of dimethyl sulfoxide was added into each well. After incubation for 10 min, the absorbance was measured at 570 nm using Synergy4 Hybrid Multi-Mode Reader (BioTek Instruments, USA). At the same time, a commercial hEGF agent (Peprotech, USA) was also detected as the positive control.
Immunofluorescence assay
COS-7 cells were incubated with rhEGF or P-EGF. Forty-eight hours later, cells were fixed with 4% paraformaldehyde for 30 min, and then permeabilized with 0.2% Triton X-100 for 30 min and blocked with 5% BSA for 30 min. After being incubated with monoclonal mouse anti-EGF antibody at 4 °C overnight, cells were stained with FITC-conjugated rabbit anti-mouse IgG (Santa Cruz) for 1 h, and then washed with PBS three times. Finally, the samples were visualized under FV1000 laser scanning confocal microscope (Olympus). 4′, 6-diamidino-2-phenylindole (DAPI) (Beyotime, China) staining was used to highlight the nuclei.
Results
Construction of the recombinant plasmids
To construct the recombinant plasmids, the coding sequences of hEGF and Pep-1-fused hEGF (P-EGF) were amplified by overlapping PCR, and then inserted into pGEX-6P-3 vector (Fig. S1). As shown in Fig. S2, the results of restriction digestion showed that the target fragments had been successfully ligated with the vector, and the results of DNA sequencing also validated that the GST tag gene and the inserted sequence were combined into one open reading frame, suggesting that the recombinant plasmids were constructed successfully.
Optimization of the soluble expression of recombinant proteins
The recombinant plasmids pGEX-rhEGF and pGEX-P-EGF were transformed into E. coli BL21 (DE3) for overexpression. To establish the best condition for soluble expression, effects of culture medium, temperature, rotational speeds, IPTG concentrations, and induction times on the yield of recombinant products were investigated. As shown in Fig. , the optimum process for soluble expression of P-EGF was as follows: the recombinant strain was firstly cultured in M9 medium containing appropriate antibiotics at 37 °C. When the OD600 of cultures reached 0.6, 0.2 mM IPTG was added to the broth for induction of the recombinant protein at 20 °C, 200 rpm for 8 h. As for rhEGF, the optimized conditions were LB medium, induction temperature 20 °C, rotational speed 200 rpm, IPTG concentration 0.4 mM, induction time 10 h. With the optimum conditions, the yield of soluble Pep-1-fused hEGF and rhEGF could reach 132 and 274 mg/L.
Fig. 1. Optimization of the fermentation conditions for the soluble expression of recombinant rhEGF and P-EGF.
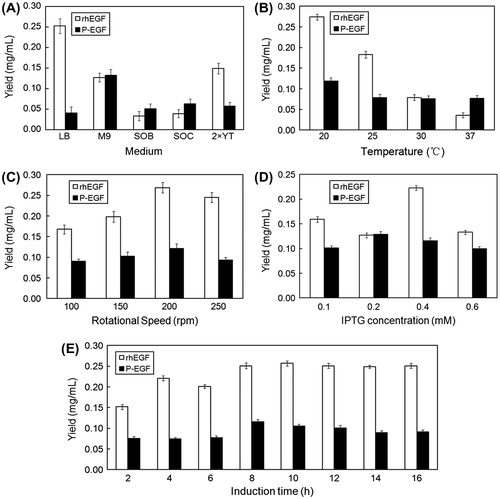
Purification and identification of the recombinant proteins
After the IPTG induction, the bacteria were split by supersonic and the recombinant GST-fused proteins were firstly purified with the glutathione affinity chromatography. As shown in Fig. (A) and (B), GST-fused proteins with the expected molecular mass could be seen in the expression product of bacteria transformed with pGEX-rhEGF and pGEX-P-EGF, respectively. After the affinity chromatography, the purified GST-fused proteins were subsequently cleaved by TEV protease digestion, and the cleaved products were then separated by the ion exchange chromatography to obtain the final target proteins rhEGF and P-EGF. As shown in Fig. (A) and (C), the target proteins (rhEGF ~ 6.2 kD, P-EGF ~ 11.2 kD) could be released from the GST-fused proteins after the TEV protease digestion, and they could be successfully purified by the ion exchange chromatography. Furthermore, Western blot was also performed to confirm the recombinant proteins. As shown in Fig. (D), both the purified products, rhEGF and P-EGF, could be detected as a single band, suggesting that the recombinant proteins had been expressed and purified successfully.
Fig. 2. Purification and identification of the recombinant proteins.
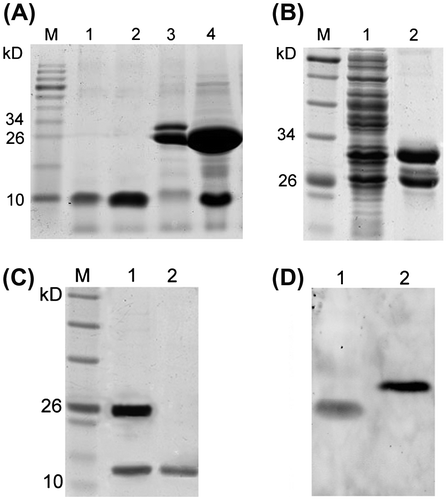
Effects of rhEGF and P-EGF on the migration and proliferation of COS-7 fibroblasts
To confirm whether the recombinant rhEGF and P-EGF had correct bioactivities, the effects of the recombinant proteins on the proliferation and migration of COS-7 fibroblasts were detected with the MTT assay and the scratch wound-healing assay, respectively. As shown in Fig. , similar with the commercial EGF, both rhEGF and P-EGF could promote the growth and motility of the fibroblasts. Furthermore, the results also showed that P-EGF could exhibit comparable potency as rhEGF, indicating that the fusion of the cell-penetrating peptide Pep-1 did not affect the function of hEGF.
Fig. 3. Effects of rhEGF and P-EGF on the migration and proliferation of COS-7 fibroblast cells.
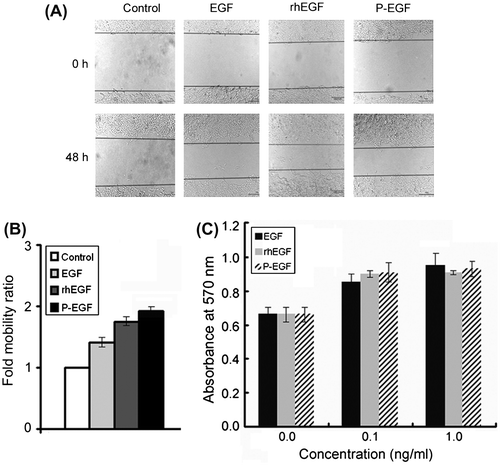
Effect of Pep-1 fusion on the transmembrane ability of EGF
To determine whether the fusion protein P-EGF had the ability of transmembrane transport, the immunofluorescence assay was performed. As shown in Fig. , compared with rhEGF, P-EGF was widely distributed on the cell membrane in the cytoplasm and the nucleus. This result suggested that fusion of cell-penetrating peptide Pep-1 could increase the transmembrane ability of EGF. What’s more, notably, there was still some P-EGF located on the cell membrane, implying that P-EGF could still bind with EGF receptor, which is a membrane-associated tyrosine kinase receptor. To our opinion, this is the reason why the fusion of the cell-penetrating peptide Pep-1 did not affect the function of EGF.
Discussion
CPPs are a growing family of peptides, including of transactivator of transcription (TAT) family (such as Tat and oligoarginine), penetralia family (such as p-Antp and plsl), chimeric CPPs (such as MPG peptides and transportan), Pep-1, etc.Citation14–19) In this study, it showed that the fusion of Pep-1 could markedly increase the transmembrane ability of hEGF, while it did not interfere with the growth-stimulating and migration-promoting functions of EGF on fibroblasts. Similarly, in 2013, Ruan and his colleagues has reported that hEGF fused with TD-1, another CPP, could not only exhibited similar bioactivity with native hEGF, but also possessed considerable higher transdermal ability than that of hEGF and the co-administration of TD-1 and hEGF.Citation5) Compared with TD-1 and other CPPs, Pep-1 is of particular interest due to its outstanding delivery rates and exclusive transmembrane mechanisms. Accumulating evidence has demonstrated that Pep-1-based protein delivery strategy presents several advantages, including rapid delivery of proteins into cells with very high efficiency, stability in physiological buffers, lack of toxicity, and lack of sensitivity to serum.Citation19) Previous studies have shown that the transmembrane ability of Pep-1 was dependent on its high affinity for the phospholipid bilayer in the cell membrane, it penetrated rapidly, in <10 min, into the nucleus of a variety of cells, and could even exhibit high transmembrane capability in many primary cell lines and different animal models.Citation19–21) In addition, although Pep-1 alone could localize to the nucleus, the cargo proteins could still have appropriate subcellular and correct effects.Citation19,20) Furthermore, while many other CPPs must be covalently bound to their cargos, Pep-1 can form non-covalent complexes with a broad spectrum of peptides, proteins, and nanoparticles, and facilitate both endocytic and non-endocytic uptake of cargos.Citation19,22) Therefore, Pep-1 has been approved as the first commercial CPP (Chariot kit, Active Motif, USA) available for the transduction of proteins.Citation19,23) Except our study, researchers has successfully delivered several other cosmetic or medical proteins, including elastin, SOD, FK506-binding proteins (FK506BP) into cells in the treatment of skin-aging symptoms or some diseases, via Pep-1.Citation19,24–26)
Many previous reports have demonstrated that Pep-1 can associate rapidly with cargoes in solution through non-covalent hydrophobic interactions and form complexes without the need for prior chemical cross-linking or chemical modifications.Citation21,27) However, to prepare the non-covalent complex formation between Pep-1 and cargos, native Pep-1 without any fusion tags is required. As Pep-1 is a 21-residue peptide, which is too small to be efficiently expressed with genetic engineering, it is usually produced using the method of chemical synthesis, which is limited in the industry for the high cost. Therefore, in recent years, fusion expression strategy of Pep-1 and cargo peptides or proteins (such as SOD, FK506BP, Heme oxygenase-1, Paraoxonase 1, PEA-15) has been widely explored by many researchers, and the results suggested that fusion expression with Pep-1 could obtain pleasing cell-permeable efficiency in cells in vitro, and penetrate easily even the blood-brain barrier in vivo.Citation28–33) Similarly, in this study, fusion of Pep-1 and hEGF could not only ensure the high expression level of the recombinant protein in E. coli, but also endue the protein with high transmembrane capability in Cos-7 fibroblasts.
In order to avoid the interfering of Pep-1 on the formation of correct conformation and activities of hEGF, here, Pep-1 and hEGF were linked with a flexible linker G4S, which could develop correct space structure and keep intrinsic biological activities of the side proteins independently.Citation5) In addition, the three disulfide bonds formed by six cysteine residues in the sequence of hEGF are required for its biologically activity, and these disulfide bonds challenged the active production in E. coli.Citation34) To solve this problem, we fused Pep-1-hEGF with GST, which could help the formation of disulfide bond. Besides, a TEV protease recognition site was added between GST and the target proteins to cleave the GST tag in the purification.
In summary, our research established a practical, simple, and easy method for the production of hEGF, and provided a novel strategy for the transmembrane transport of hEGF and other peptide-derived drugs or cosmetics.
Authors contributions
Xue-Gang Luo and Tong-Cun Zhang designed the experiments. De-Yun Ma, Yue Wang, Wen Li, Chong-Xi Wang, Ying-Ying He, Xiang-Chao Gu and Xiu-Mei Li performed the experiments. Xue-Gang Luo, De-Yun Ma and Yue Wang analyzed data. Hao Zhou contributed reagents, materials and tools. Xue-Gang Luo, De-Yun Ma and Yue Wang wrote the paper.
Disclosure statement
No potential conflict of interest was reported by the authors.
Supplemental material
The supplemental material for this paper is available at http://dx.doi.org/10.1080/09168451.2015.1091714.
Table_S1.doc
Download MS Word (35 KB)Supplementary_figure_legends.doc
Download MS Word (31.5 KB)Fig._S2.tif
Download TIFF Image (155.1 KB)Fig._S1.tif
Download TIFF Image (57.1 KB)References
- Gorouhi F, Maibach HI. Role of topical peptides in preventing or treating aged skin. Int. J. Cosmet. Sci. 2009;31:327–345.10.1111/ics.2009.31.issue-5
- Secchi G. Role of protein in cosmetics. Clin. Dermatol. 2008;26:321–325.10.1016/j.clindermatol.2008.04.004
- Torin Huzil J, Sivaloganathan S, Kohandel M, et al. Drug delivery through the skin: molecular simulations of barrier lipids to design more effective noninvasive dermal and transdermal delivery systems for small molecules, biologics, and cosmetics. Wiley Interdiscip. Rev. Nanomed. Nanobiotechnol. 2011;3:449–462.
- Brown MB, Martin GP, Jones SA, et al. Dermal and transdermal drug delivery systems: current and future prospects. Drug Delivery. 2006;13:175–187.10.1080/10717540500455975
- Ruan RQ, Wang SS, Wang CL, et al. Transdermal delivery of human epidermal growth factor facilitated by a peptide chaperon. Eur. J. Med. Chem. 2013;62:405–409.10.1016/j.ejmech.2012.12.054
- Hardwicke J, Schmaljohann D, Boyce D, et al. Epidermal growth factor therapy and wound healing–past, present and future perspectives. Surgeon. 2008;6:172–177.10.1016/S1479-666X(08)80114-X
- Herbst RS. Review of epidermal growth factor receptor biology. Int. J. Radiat. Oncol. Biol. Phys. 2004;59:S21–S26.10.1016/j.ijrobp.2003.11.041
- Boonstra J, Rijken P, Humbel B, et al. The epidermal growth factor. Cell Biol. Int. 1995;19:413–430.10.1006/cbir.1995.1086
- Jeon SO, Hwang HJ, Oh DH, et al. Enhanced percutaneous delivery of recombinant human epidermal growth factor employing nano-liposome system. J. Microencapsulation. 2012;29:234–241.10.3109/02652048.2011.646327
- Duconge J, Prats PA, Valenzuela C, et al. Topical disposition of two strengths of a 125I-rhEGF jelly in rat skin wounds. Biopharm. Drug Dispos. 2004;25:193–201.10.1002/(ISSN)1099-081X
- Patel LN, Zaro JL, Shen WC. Cell penetrating peptides: intracellular pathways and pharmaceutical perspectives. Pharm. Res. 2007;24:1977–1992.10.1007/s11095-007-9303-7
- Zorko M, Langel U. Cell-penetrating peptides: mechanism and kinetics of cargo delivery. Adv. Drug Delivery Rev. 2005;57:529–545.10.1016/j.addr.2004.10.010
- Jarver P. The use of cell-penetrating peptides as a tool for gene regulation. Drug Discovery Today. 2004;9:395–402.10.1016/S1359-6446(04)03042-9
- Oehlke J, Scheller A, Wiesner B, et al. Cellular uptake of an α-helical amphipathic model peptide with the potential to deliver polar compounds into the cell interior non-endocytically. Biochimica et Biophysica Acta (BBA) – Biomembranes. 1998;1414:127–139.
- Tara B, Wyman FN, Zelphati O, et al. Design, synthesis, and characterization of a cationic peptide that binds to nucleic acids and permeabilizes bilayers. Biochemistry. 1997;36:3008–3017.
- Rittner K, Benavente A, Bompard-Sorlet A, et al. New basic membrane-destabilizing peptides for plasmid-based gene delivery in vitro and in vivo. Mol. Ther. 2002;5:104–114.10.1006/mthe.2002.0523
- Fernández-Carneado J, Kogan MJ, Castel S, et al. Potential peptide carriers: amphipathic proline-rich peptides derived from the N-terminal domain of gamma-zein. Angew. Chem. Int. Ed. Engl. 2004;43:1811–1814.10.1002/(ISSN)1521-3773
- Morris MCVP, Chaloin L, Heitz F, et al. A new peptide vector for efficient delivery of oligonucleotides into mammalian cells. Nucleic Acids Res. 1997;25:2730–2736.10.1093/nar/25.14.2730
- Morris MC, Depollier J, Mery J, et al. A peptide carrier for the delivery of biologically active proteins into mammalian cells. Nat. Biotechnol. 2001;19:1173–1176.10.1038/nbt1201-1173
- Henriques ST, Castanho MA, Pattenden LK, et al. Fast membrane association is a crucial factor in the peptide pep-1 translocation mechanism: a kinetic study followed by surface plasmon resonance. Biopolymers. 2010;94:314–322.10.1002/bip.21367
- Deshayes S, Morris M, Heitz F, et al. Delivery of proteins and nucleic acids using a non-covalent peptide-based strategy. Adv. Drug Delivery Rev. 2008;60:537–547.10.1016/j.addr.2007.09.005
- Petrescu AD, Vespa A, Huang H, et al. Fluorescent sterols monitor cell penetrating peptide Pep-1 mediated uptake and intracellular targeting of cargo protein in living cells. Biochim. Biophys. Acta. 2009;1788:425–441.10.1016/j.bbamem.2008.09.015
- Loudet A, Han J, Barhoumi R, et al. Non-covalent delivery of proteins into mammalian cells. Org. Biomol. Chem. 2008;6:4516–4522.10.1039/b809006h
- Nasrollahi SA, Fouladdel S, Taghibiglou C, et al. A peptide carrier for the delivery of elastin into fibroblast cells. Int. J. Dermatol. 2012;51:923–929.
- Zhang YE, Wang JN, Tang JM, et al. In vivo protein transduction: delivery of PEP-1-SOD1 fusion protein into myocardium efficiently protects against ischemic insult. Mol. Cells. 2009;27:159–166.10.1007/s10059-009-0020-4
- Ahn EH, Kim DW, Shin MJ, et al. Fenobam promoted the neuroprotective effect of PEP-1-FK506BP following oxidative stress by increasing its transduction efficiency. BMB Rep. 2013;46:561–566.10.5483/BMBRep.2013.46.11.080
- Gros E, Deshayes S, Morris MC, et al. A non-covalent peptide-based strategy for protein and peptide nucleic acid transduction. Biochim. Biophys. Acta. 2006;1758:384–393.10.1016/j.bbamem.2006.02.006
- Kim SY, Sohn EJ, Kim DW, et al. Transduced PEP-1-FK506BP ameliorates atopic dermatitis in NC/Nga mice. J. Invest. Dermatol. 2011;131:1477–1485.10.1038/jid.2011.49
- Eum WS, Kim DW, Hwang IK, et al. In vivo protein transduction: biologically active intact pep-1-superoxide dismutase fusion protein efficiently protects against ischemic insult. Free Radical Biol. Med. 2004;37:1656–1669.
- Kwon SW, Sohn EJ, Kim DW, et al. Anti-inflammatory effect of transduced PEP-1-heme oxygenase-1 in Raw 264.7 cells and a mouse edema model. Biochem. Biophys. Res. Commun. 2011;411:354–359.10.1016/j.bbrc.2011.06.147
- Kim MJ, Jeong HJ, Kim DW, et al. PEP-1-PON1 protein regulates inflammatory response in raw 264.7 macrophages and ameliorates inflammation in a TPA-induced animal model. PLoS ONE. 2014;9:e86034.10.1371/journal.pone.0086034
- Ahn EH, Kim DW, Shin MJ, et al. PEP-1-PEA-15 protects against toxin-induced neuronal damage in a mouse model of Parkinson’s disease. Biochim. Biophys. Acta. 2014;1840:1686–1700.10.1016/j.bbagen.2014.01.004
- Kim W, Kim DW, Yoo DY, et al. Neuroprotective effects of PEP-1-Cu,Zn-SOD against ischemic neuronal damage in the rabbit spinal cord. Neurochem. Res. 2012;37:307–313.10.1007/s11064-011-0613-0
- Abdull Razis AF, Ismail EN, Hambali Z. Expression of recombinant human epidermal growth factor in Escherichia coli and characterization of its biological activity. Appl. Biochem. Biotechnol. 2007;144:249–261.