Abstract
Advanced glycation end-products (AGEs) are extremely accumulated in the retinal vascular and epithelial cells of diabetes mellitus (DM) patients, particularly with diabetic retinopathy (DR). To elucidate the pathogenesis of the AGE-induced toxicity to retinal epithelial cells, we investigated the role of Fas–Fas ligand (FasL) signaling and mitochondrial dysfunction in the AGE-induced apoptosis. Results demonstrated that the AGE-BSA- induced apoptosis of retinal ARPE-19 cells. And the AGE-BSA treatment caused mitochondrial dysfunction, via deregulating the B-cell lymphoma 2 (Bcl-2) signaling. Moreover, the Fas/FasL and its downstreamer Caspase 8 were promoted by the AGE-BSA treatment, and the exogenous α-Fas exacerbated the activation of Caspase 3/8. On the other side, the siRNA-mediated knockdown of Fas/FasL inhibited the AGE-BSA-induced apoptosis. Taken together, we confirmed the activation of Fas–FasL signaling and of mitochondrial dysfunction in the AGE-BSA-promoted apoptosis in retinal ARPE-19 cells, implying the important role of Fas–FasL signaling in the DR in DM.
Graphical abstract
AGE-BSA activates the Fas–FasL signaling in human retinal ARPE-19 cellsPromoted Fas, FasL and activated caspase 8 in the AGE-BSA-treated ARPE-19 cells, revealed by western blot analysis.
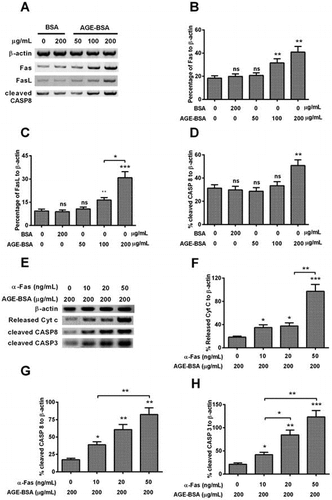
Diabetic retinopathy (DR) is the most severe vas capillary complication of diabete that can cause irreversible blind, and has become the main disease causing blind. Previous research performed by WHO predicted DR-causing blind will account for 4.8% in 37,000,000 blind people worldwide.Citation1) The pathogenesis of DR is very complicated; it often begins with the change of retinal blood vessel including retinal inflammation, vascular permeability increase, and abnormal angiogenesis in retina.Citation2) It is believed that mitochondrial dysfunction-induced apoptosis plays an important role in the pathogenesis of DR.Citation3,4)
In diabetic patients, proteins, amino acids, lipids, and nucleic acids can react non-enzymatically with the sugars including glucose, fructose, and aldotriose to form panels of covalent compounds, which is termed “advanced glycation end-product” (AGE).Citation5) It has been found that AGEs accumulate in many organisms with accelerated rate in diabetic patients, which is a dominant reason to cause the selected loss of cells around retinal capillary.Citation6) Many evidences suggest that the binding of AGE to its receptor RAGE induces oxidative stress (OxS) and causes inflammation and thrombus formation, which play a key role in the pathological change of blood vessel.
OxS is intimately linked to the AGE-formation, multiple reports indicate that the increased OxS plays a significant role in the incidence of diabetic complications, such as retinopathy.Citation7,8) As well, AGEs accumulate in the blood capillary cells irreversibly in the later stage, and binding of AGEs to RAGE activate the NF-ĸB and produce overdose reactive oxygen species (ROS) which activate apoptosis consequently.Citation9) Caspase-mediated Fas–FasL pathway is also a classic pathway to induce the apoptosis. Particularly, the study on the Caspase-mediated Fas–FasL apoptotic pathway was often neglected in those researches. Although multiple evidences indicate the AGEs play an important role in the pathogenesis of retinopathy by OxS-induced apoptosis.Citation10–12) However, it is not clear about the molecular mechanism of AGEs-induced Fas–FasL apoptotic pathway in the diabetic retina.
In present research, to discover the pathogenesis of the AGE-induced toxicity in retinal epithelial cells, we investigated the AGEs-induced apoptosis and mitochondrial dysfunction in the AGE-treated ARPE-19 cells, and performed detailed research on Fas apoptotic pathway, and determined the influence of apoptosis-related genes silences on the AGE-induced apoptosis.
Materials and methods
Cell culture and AGE-BSA treatment
Human retinal ARPE-19 cells were purchased from the American Type Culture Collection, and were cultured in Dulbecco’s Modified Eagle Medium: Nutrient Mixture F-12 (DMEM/F-12) (Life technologies, USA) supplemented with 10% fetal bovine serum (Invitrogen, Carlsbad, CA, USA), 100 U/mL penicillin, and 100 mg/mL streptomycin at 37 °C in 5% CO2. Cell layer was treated by trypsin-EDTA solution and was subcultured at a ratio of 1:3 to 1:5 every 3–4 days.Citation13) BSA and AGE-BSA were purchased from BioVision (Mountain View, CA, USA). Briefly, the AGE-BSA was produced by reacting BSA with glycolaldehyde under sterile conditions followed by extensive dialysis and purification steps. Fluorescence of AGE-BSA was confirmed by fluorescence spectrophotometry with Ex./Em. = 370/440 nm. Glycated BSA shows a 7000% increase in fluorescence in compared to control BSA.
For the AGE-BSA treatment, ARPE-19 cells were seeded in 12-well plate and were cultured to 80–90% confluence. Then, the confluent cells were treated with 0, 50, 100, 150, or 200-μg/mL AGE-BSA (with BSA as control) for 24 or 48 h.
Determination of complex IV activity and mitochondrial membrane potential (MMP)
The enzyme activity of complex IV was examined with the mouse mitochondrial respiratory chain complex IV ELISA Kit (Jianglaibio, shanghai, China) according to the kit’s manual. In brief, ARPE-19 cells post treatments were collected and were re-suspended for the protein isolation with a Mitochondria Isolation Kit for Tissue (Life technologies, USA). The protein samples were quantified and diluted, and were added into plate wells to incubate at room temperature for 3 h. Then 300 μL Solution 1 was added into each well for washing, and 200 μL of Assay Solution was added into each well carefully. Subsequently, the binding was assayed by a plate reader which was set up to a kinetic program to measure absorbance at 550 nm at 30 °C for 120 min, with measurement interval of approximately 1 min. Results were described as percentage to complex IV activity of control ARPE-19 cells.
The MMP was assayed with a MMP Assay Kit (Bio Excellence International TechCo, Beijing, China) according to the kit’s instruction.1 × 106 ARPE-19 cells were incubated with the 500-μl dying solution containing JC-1 for 20 min at 37 °C. After washing twice with dying binding buffer, the cells were resuspended in 500-μL 1×Incubation Buffer for flow cytometry with excitation/emission fluorescence of 488/530 nm.
Apoptosis assay
The apoptosis of ARPE-19 cells was examined with an Annexin V-FITC apoptosis detection kit (Sigma, Aldrich, USA). Briefly, the treated cells were centrifugated at 2000 rpm for 5 min and washed twice with PBS, then the cells were suspended in the 400 μL 1×Binding Buffer at a concentration of 1 × 105 cells/mL, then 5 μL of Annexin V-FITC and Propidium Iodide was added in turn and mixed, the treated cells were placed in the dark at RT for 5–15 min to perform flow cytometry analysis. And the percentage of Annexin V-positive cells or Annexin V-plus-PI positive cells was calculated. All experiments were performed in triplicate.
MTT cell viability assay
ARPE-19 cells viability was measured with MTT method in 96-well plates. Briefly, after cells were treated by indicated dosages of AGE-BSA or BSA, 20 μL of MTT in the final concentration of 5 mg/ml was added and the cells were incubated for 4 h at 37 °C. Then the supernatants were carefully removed, 200 μL of DMSO was added into each well. The plate was put into a 37 °C incubator to dissolve air bubbles, OD570 value of each well was measured at 570 nm wavelength using a microplate reader (Thermo scientific, USA). The results were expressed as (A570 of control wells-A570 of treated wells)/(A570 of control wells-A570 of blank wells) × 100%.
Real-time PCR
ARPE-19 cells were prepared using Qiagen RNeasy Mini kit (Qiagen, German). Total RNA was converted to cDNA using SuperScript® III Reverse Transcriptase (Invitrogen, USA). The qPCR was made using an ABI PRISM 7300 detection system at 95 °C for 5 min, followed by 40 cycles of 95 °C for 15 s and 60 °C for 1 min. Housekeeping gene β-actin was simultaneously amplified to standardize the amount of sample RNA. Relative quantification of gene transcription level was performed by the -ΔΔct method.Citation14)
Western blot
ARPE-19 cells were seeded at a density of 5 × 105 cells/well in 6-well plate with 2-ml culture medium per well. After 48 h, cells were treated with AGE-BSA, α-Fas + AGE-BSA, and SiRNA transfection at the indicated dosages, separately. Then cells were collected and were lysed with lysis buffer (Invitrogen, USA) on ice for 20 min. The cell lysates were centrifugated at 15,000×g at 4 °C for 30 min. The supernatant was collected as the total cellular protein extract. Protein concentration was determined using the BCA Protein Assay Kit (Kangwei shiji company, Beijing). The samples of total cellular protein were loaded onto 10% SDS polyacrylamide gel. The separated proteins were electrophoretically transferred to PVDF membranes (Bio-rad, USA). The membranes were blocked overnight in blocking buffer containing PBST and 5% non-fatty milk. Then the membranes were incubated with primary antibodies against Bcl-2, Bcl-XL, Bax, Cyt c, Caspase 3, Fas and Fasl, and Caspase 8 for 1 h separately, and were washed with PBST for 4 times subsequently. Following incubating with the secondary HRP-conjugated antibody for 1 h, the PVDF membranes were washed for 4 times and were treated with ECL reagent and exposed to X-ray film. Each band was quantified using Image J software.
Statistical analysis
Data are depicted as the mean ± standard deviation that is calculated from three independent experiments. Student’s t-test was used for a comparison between two groups. One-way analysis of variance was used for multiple comparisons between three or more groups. p < 0.05 was considered as a statistically significant difference.
Results
AGE-BSA treatment could reduce the viability and induce apoptosis in human retinal ARPE-19 cells efficiently
To invest the effect of AGE-BSA on cells’ viability and apoptosis, ARPE-19 cells were exposed to different concentrations (50, 100, 150, and 200 μg/mL) of AGE-BSA and 200 μg/mL BSA as negative control for 24 or 48 h, separately. Then the percentage of viable cells was determined by MTT assay, as shown in Fig. (A), the percentages of groups treated by 150 or 200 μg/mL of AGE-BSA were decreased by 22% (p < 0.05) or 33% (p < 0.01), respectively. And such viability reduction was repeated at either 24 or 48 h (Fig. (B)), the percentage of viable cells, the AGE-BSA caused 20 or 40% reduction at 24 or 48 h after treatment. Specially, statistical difference was also observed between two groups. We also checked the percentage of apoptotic cells of groups, as indicated in Fig. (C), when the concentration of AGE-BSA reached 100 μg/mL, the apoptotic percentage of treated cells rose to a higher level when compared with the control group (p < 0.05). As well, the apoptotic percentage displayed a dosage-dependent increase when the concentrations of AGE-BSA reached over 100 μg/mL.
Fig. 1. AGE-BSA reduces the viability and induces apoptosis in human retinal ARPE-19 cells.
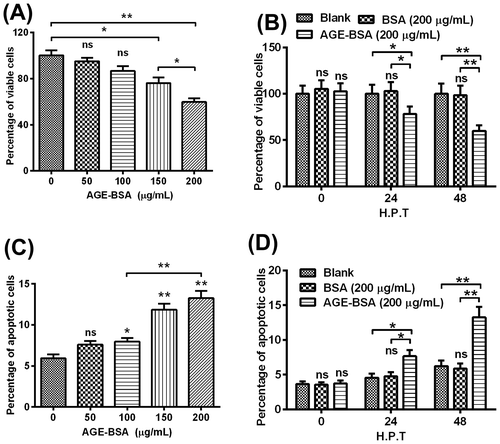
Then we checked the percentages of apoptotic cells, respectively, at the different time points post-treatment. The percentages of apoptotic cells in the AGE-BSA-treated groups displayed statistic differences whether compared with the blank or with the BSA-treated group, at 24 h and 48 h post-treatment, respectively (Fig. (D)). In detail, as to the apoptotic rate, it was up-regulated by 60 and 110%, respectively, at 24 or 48 h post-treatment, compared with the BSA-treated groups.
AGE-BSA induced apoptosis by interfering the balance of Bcl-2 and Bax and promoting the mitochondrial dysfunction in human retinal ARPE-19 cells
The relative MMP level or Complex IV activity were determined after treatment of 200 μg/mL of AGE-BSA/BSA for 48 h. As indicated in Fig. (A) and (B), AGE-BSA treatment decreased the percentages of complex IV factor and MMP by 30% (p < 0.01) and 25% (p < 0.05) for each. In the next step, we checked the expressions of β-actin, Bcl-2, Bcl-XL, and Bax, respectively. Intuitively, expressions of Bcl-2 and Bcl-XL were greatly down-regulated and Bax expression was improved (Fig. (C)). After quantifying the protein amount of each band, we found the Bcl-2 and Bcl-XL were down-regulated by 67% (p < 0.01) and 43% (p < 0.05) when compared with BSA treatment group, respectively; on the contrary, Bax was improved by 122% (p < 0.01) in comparison with the control group(Fig. (D)). At last, we checked the expression levels of key apoptosis-related molecules: Cyt c and cleaved Caspase 3, and the house-keeping gene β-actin by WB; obviously, AGE-BSA treatment caused the highest expression levels of Cyt c and cleaved Caspase 3 (Fig. (E)), the Cyt c release and cleaved Caspase 3 were up-regulated by 43% (p < 0.01) and 188% (p < 0.01) in comparison with control for each.
Fig. 2. AGE-BSA promotes the mitochondrial dysfunction in human retinal ARPE-19 cells.
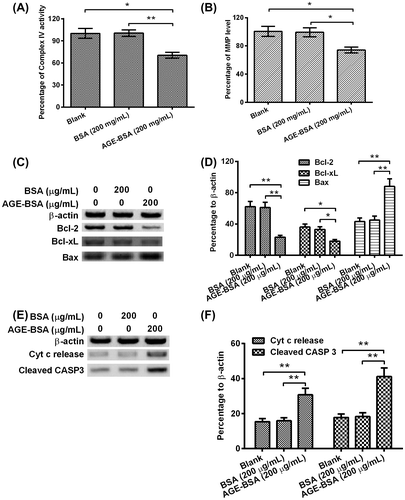
AGE-BSA-induced apoptosis in human retinal ARPE-19 cells by activating the Fas–FasL signaling
To further investigate the signal pathway activated by AGE-BSA, we determined the expressions of Fas, Fas-L, cleaved Caspase 8, and β-actin using WB in the ARPE-19 cells treated by different concentrations of AGE-BSA and BSA (Fig. (A)). As shown in the Fig. (B) and (C), when the concentration of AGE-BSA reached over 100 μg/mL, the percentages of Fas/FasL to β-actin displayed statistical differences in comparison with groups of blank, BSA, and 50 μg/mL AGE-BSA, respectively. As well, the percentage of FasL to β-actin of 200 μg/ml of AGE-BSA-treated group was elevated by 50% (p < 0.01) when compared with the group treated by 100 μg/mL of AGE-BSA. For percentage of cleaved Caspa8 to β-actin, only 200 μg/mL of AGE-BSA treatment improved that by 42.8% or so (p < 0.01) compared with the other groups (Fig. (D)).
Fig. 3. AGE-BSA activates the Fas–FasL signaling in human retinal ARPE-19 cells.
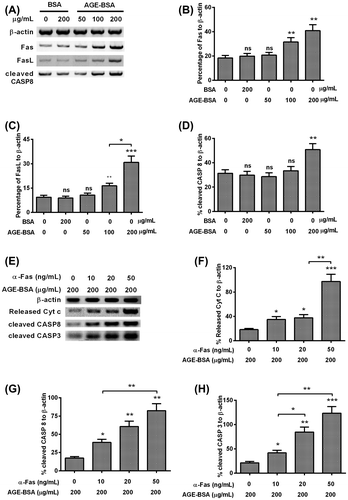
Besides, in order to discover the synergy of Fas combined with AGE-BSA on the cells apoptosis, we checked the expressions of released Cyt c, cleaved Caspase 8, cleaved Caspase 3, and β-actin in the treated cells (Fig. (E)). As indicated in Fig. (F)–(H), the Cyt c release, cleaved Caspase 8, and cleaved Caspase 3 all displayed a α-Fas dose-dependent increase, and reached the top when the concentration of α-Fas was 50 ng/ml. For the Cyt c release, it was improved by 60% (p < 0.01) under synergized treatment with 50 ng/mL of α-Fas when compared with the group treated by 20 ng/mL of α-Fas. Similar results were also observed in the cleaved Caspase 8 and Caspase 3, for cleaved Caspase 8, it increased by 40% (p < 0.01) in the 50 ng/mL α-Fas treated group compared with 10 ng/mL α-Fas group. As to the cleaved Caspase 3, it was up-regulated by 45% (p < 0.05) and 65% (p < 0.01) in the 20-ng/mL α-Fas-treated group and 50-ng/mL α-Fas-treated group compared with 10 ng/mL α-Fas-treated group, respectively.
Fas or FasL silence suppressed the AGE-BSA-induced activation of Caspase 8 and Caspase 3 in human retinal ARPE-19 cells
To confirm the knockdown of Fas/Fas-L on AGE-BSA-induced activation of Caspase 8 and Caspase 3 in human retinal ARPE-19 cells, scramble siRNA, siRNA-Fas, and siRNA-FasL were synthesized and transfected into ARPE-19 cells. The siRNA-Fas and siRNA-FasL inhibited the mRNA level of Fas and Fas-L efficiently (Fig. (A) and (B)). Then the Fas, Fas-L, cleaved Caspase 8, and cleaved Caspase 3 in the cells transfected by scrambler siRNA, siRNA-Fas, or with siRNA-FasL, in the presence of 200 μg/mL AGE-BSA (Fig. (C)). As indicated in Fig. (D), siRNA-Fas + AGE-BSA and siRNA-FasL + AGE-BSA caused 20% (p < 0.01) and 13% (p < 0.01) reduction on Fas and FasL expressions, respectively. Consequently, siRNA-Fas + AGE-BSA and siRNA-FasL + AGE-BSA both reduced cleaved Caspase 8 and Caspase 3 efficiently (Fig. (E)).
Fig. 4. Fas or FasL knockdown with siRNA inhibited the AGE-BSA-induced activation of Caspase 8 and Caspase 3 in human retinal ARPE-19 cells.
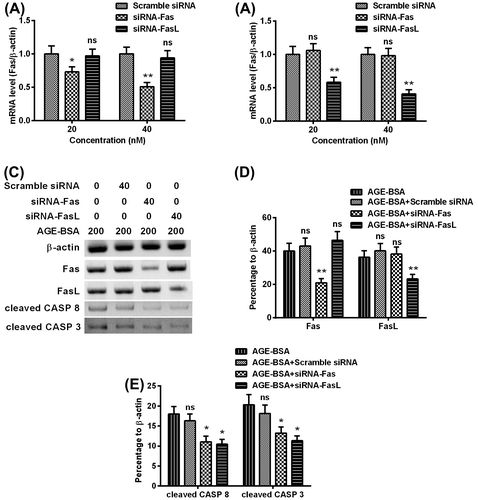
Discussions
In this research, we found the AGE-BSA reduced the viability and induced aopotpsis in human retinal ARPE-19 cells. In detail, AGEs promoted the mitochondrial dysfunction in ARPE-19 cells and down-regulated the anti-apoptosis related molecules, Bcl-2 and Bcl-XL, and improved the apoptosis-related molecule: Bax subsequently. Next, we checked the Fas/FasL induced apoptosis and found the Fas–FasL signaling-induced Caspase 8 were greatly promoted by the AGE-BSA treatment, and the exogenous α-Fas aggravated the Cyt c release, Caspase 8 activation and Caspase 3 activation. Besides, the silence of Fas/FasL inhibited the AGE-BSA-induced activation of Caspase 8 and Caspase 3.
Previous research indicated that AGEs induced apoptosis in retinal pericytes, corneal endothelial cells, neuronal cells, renal mesangial cells, etc.Citation15–17) Above all, enhanced apoptosis of these cells is related to diabetic complications such as retinopathy, neuropathy, and nephropathy.Citation18,19) To date, the mechanisms of AGEs-induced apoptosis are still not very clear. Previous reports indicated that AGEs may induce the OxS, and improve the pro-apoptotic genes expressions and inhibit the expression of anti-apoptotic genes Bcl-2 in corneal epithelial cells.Citation20) Bcl-2 and Bax are considered as key apoptosis-related genes, Bcl-2 is anti- and Bax is pro-apoptotic gene separately, and apoptosis is regulated by the balance of Bcl-2 and Bax expressions.Citation21) Over-expressed Bax/ Bcl-2 promotes/inhibits cell’s apoptosis, respectively, and the relative level of Bcl-2 to Bax determines the initiation of cell apoptosis.Citation22,23) In our research, we also found the AGE-BSA treatment decreased the Bcl-2 and Bcl-XL expressions and improved the Bax expression simultaneously. Besides, the quantifications of complexIV and MMP demonstrated the AGE-BSA-induced apoptosis by promoting the mitochondrial dysfunction in human retinal ARPE-19 cells.
Another Fas–FasL apoptotic pathway was also studied in the research. So far, fewer researches have been performed to investigate the Fas apoptotic pathway induced by AGEs. Interestingly, we observed that AGE-BSA activated the Fas–FasL signaling in human retinal ARPE-19 cells, and the Fas and FasL expressions displayed a AGE-BSA dose-dependent increase. Also, exogenous α-Fas synergized with AGE exacerbated the Cyt c release, Caspase 8 activation and Caspase 3 activation. These results indicate the importance of Fas–FasL signaling on the pathogenesis of DR.
OxS is also considered as an important factor causing DR; exaggerated production of ROS has been implicated in many diabetic complications: neuropathy, nephropathy, and retinopathy.Citation24–26) Above all, OxS is intimately related to the AGE formation.Citation27,28) Thus, AGE formation and OxS are synergized for the development of diabetes-related complications via the cell apoptosis.Citation29,30) In this research, we did not investigate the effect of OxS on the apoptosis in human retinal cells, further research should be performed to discover the unique mechanism of OxS-induced apoptosis.
In conclusion, we confirmed the activation of Fas–FasL signaling and of mitochondrial dysfunction in the AGE-BSA-promoted apoptosis in retinal ARPE-19 cells, and disclosed the molecular mechanism of Fas–FasL signaling and of mitochondrial dysfunction in the DR in DM. This research will provide valuable information for the prevention and treatment of DR.
Authors contribution
Pu Wang and Yiqiao Xing designed the study; Pu Wang, Changzheng Chen, and Zhen Chen performed experiments. Zhimin Qian performed the statistical analysis. And Pu Wang and Yiqiao Xing wrote the paper.
Disclosure statement
No potential conflict of interest was reported by the authors.
References
- Resnikoff S, Pascolini D, Etya’ale D, et al. Global data on visual impairment in the year 2002. Bull. World Health Organ. 2004;82:844–851.
- Park K, Chen Y, Hu Y, et al. Nanoparticle-mediated expression of an angiogenic inhibitor ameliorates ischemia-induced retinal neovascularization and diabetes-induced retinal vascular leakage. Diabetes. 2009;58:1902–1913.10.2337/db08-1327
- Joshi MS, Williams D, Horlock D, et al. Role of mitochondrial dysfunction in hyperglycaemia-induced coronary microvascular dysfunction: Protective role of resveratrol. Diab. Vasc. Dis. Res. 2015;12:208–216.10.1177/1479164114565629
- Soto-Urquieta MG, López-Briones S, Pérez-Vázquez V, et al. Curcumin restores mitochondrial functions and decreases lipid peroxidation in liver and kidneys of diabetic db/db mice. Biol. Res. 2014;47:74.10.1186/0717-6287-47-74
- Yamagishi S, Inagaki Y, Okamoto T, et al. Advanced glycation end product-induced apoptosis and overexpression of vascular endothelial growth factor and monocyte chemoattractant protein-1 in human-cultured mesangial cells. J. Biol. Chem. 2002;277:20309–20315.10.1074/jbc.M202634200
- Iwamoto K, Kanno K, Hyogo H, et al. Advanced glycation end products enhance the proliferation and activation of hepatic stellate cells. J. Gastroenterol. 2008;43:298–304.10.1007/s00535-007-2152-7
- Giordano C, Roberts R, Krentz K, et al. Catalase therapy corrects oxidative stress-induced pathophysiology in incipient diabetic retinopathy. Invest. Ophthalmol. Vis. Sci. 2015;56:3095–3102.
- Yokota T, Kamimura N, Igarashi T, et al. Protective effect of molecular hydrogen against oxidative stress caused by peroxynitrite derived from nitric oxide in rat retina. Life Sci. 2015;43:568–577.
- Sheikpranbabu S, Haribalaganesh R, Banumathi E, Lee kJ, et al. Pigment epithelium-derived factor inhibits advanced glycation end-product-induced angiogenesis and stimulates apoptosis in retinal endothelial cells. Life Sci. 2009;85:719–731.10.1016/j.lfs.2009.09.015
- Deng J, Wu G, Yang C, et al. Rosuvastatin attenuates contrast-induced nephropathy through modulation of nitric oxide, inflammatory responses, oxidative stress and apoptosis in diabetic male rats. J. Transl. Med. 2015;13:53.10.1186/s12967-015-0416-1
- Orman D, Vardi N, Ates B, et al. Aminoguanidine mitigates apoptosis, testicular seminiferous tubules damage, and oxidative stress in streptozotocin-induced diabetic rats. Tissue Cell. 2015;47:284–290.
- Koyuturk M, Sacan O, Karabulut S, et al. The role of ghrelin on apoptosis, cell proliferation and oxidant-antioxidant system in the liver of neonatal diabetic rats. Diabetes. 2015;39:834–841.
- Dunn KC, Aotaki-Keen AE, Putkey FR, et al. ARPE-19, a human retinal pigment epithelial cell line with differentiated properties. Exp. Eye Res. 1996;62:155–170.10.1006/exer.1996.0020
- Chen J, Feilotter HE, Paré GC, et al. MicroRNA-193b represses cell proliferation and regulates cyclin d1 in melanoma. Am. J. Pathol. 2010;176:2520–2529.10.2353/ajpath.2010.091061
- Denis U, Lecomte M, Paget C, et al. Advanced glycation end-products induce apoptosis of bovine retinal pericytes in culture: involvement of diacylglycerol/ceramide production and oxidative stress induction. Free Radic. Biol. Med. 2002;33:236–247.10.1016/S0891-5849(02)00879-1
- Kaji Y, Amano S, Usui T, et al. Expression and function of receptors for advanced glycation end products in bovine corneal endothelial cells. Invest. Ophthalmol. Vis. Sci. 2003;44:521–528.10.1167/iovs.02-0268
- Kasper M, Roehlecke C, Witt M, et al. Induction of apoptosis by glyoxal in human embryonic lung epithelial cell line L132. Am. J. Respir. Cell Mol. Biol. 2000;23:485–491.10.1165/ajrcmb.23.4.4117
- Yamagishi S, Inagaki Y, Amano S, et al. Pigment epithelium-derived factor protects cultured retinal pericytes from advanced glycation end product-induced injury through its antioxidative properties. Biochem. Biophys. Res. Commun. 2002;296:877–882.10.1016/S0006-291X(02)00940-3
- Yamagishi S, Maeda S, Matsui T, et al. Role of advanced glycation end products (AGEs) and oxidative stress in vascular complications in diabetes. Biochim. Biophys. Acta. 2012;1820:663–671.10.1016/j.bbagen.2011.03.014
- Shi L, Yu X, Yang H, et al. Advanced glycation end products induce human corneal epithelial cells apoptosis through generation of reactive oxygen species and activation of JNK and p38 MAPK pathways. PLoS One. 2013;8:e66781.10.1371/journal.pone.0066781
- Takagi-Morishita Y, Yamada N, Sugihara A, et al. Mouse uterine epithelial apoptosis is associated with expression of mitochondrial voltage-dependent anion channels, release of cytochrome C from mitochondria, and the ratio of Bax to Bcl-2 or Bcl-X. Biol. Reprod. 2003;68:1178–1184.
- Yu X, Kubota H, Wang R, et al. Involvement of Bcl-2 family genes and Fas signaling system in primary and secondary male germ cell apoptosis induced by 2-bromopropane in rat. Toxicol. Appl. Pharmacol. 2001;174:35–48.10.1006/taap.2001.9187
- Tsukahara S, Yamamoto S, Tin Tin Win S, et al. Inhalation of low-level formaldehyde increases the bcl-2/bax expression ratio in the hippocampus of immunologically sensitized mice. Neuroimmunomodulation. 2006;13:63–68.10.1159/000094829
- Forbes JM, Coughlan MT, Cooper ME. Oxidative stress as a major culprit in kidney disease in diabetes. Diabetes. 2008;57:1446–1454.10.2337/db08-0057
- Weinberg E, Maymon T, Weinreb M. AGEs induce caspase-mediated apoptosis of rat BMSCs via TNFalpha production and oxidative stress. J. Mol. Endocrinol. 2014;52:67–76.
- Wang XL, Yu T, Yan QC, et al. AGEs promote oxidative stress and induce apoptosis in retinal pigmented epithelium cells RAGE-dependently. Curr. Med. Chem. 2015;56:449–460.
- Loske C, Neumann A, Cunningham AM, et al. Cytotoxicity of advanced glycation endproducts is mediated by oxidative stress. J. Neural. Transm. 1998;105:1005–1015.10.1007/s007020050108
- Maiese K, Chong ZZ, Shang YC. Mechanistic insights into diabetes mellitus and oxidative stress. Curr. Med. Chem. 2007;14:1729–1738.10.2174/092986707781058968
- Liu L, Lao W, Ji QS, et al. Lycium barbarum polysaccharides protected human retinal pigment epithelial cells against oxidative stress-induced apoptosis. Int. J. Ophthalmol. 2015;8:11–16.
- Ma J, Liu T, Dong X. Advanced glycation end products of bovine serum albumin-induced endothelial-to-mesenchymal transition in cultured human and monkey endothelial cells via protein kinase B signaling cascades. Mol. Vis. 2010;16:2669–2679.