Abstract
An oxidative metabolite of lutein, 3′-hydroxy-ε,ε-caroten-3-one, inhibited the differentiation of 3T3-L1 cells to adipocytes and the subsequent triacylglycerol production, but lutein did not. The α,β-unsaturated carbonyl structure of 3′-hydroxy-ε,ε-caroten-3-one was considered to participate in the inhibitory effect, suggesting that this lutein metabolite has the potential to prevent metabolic syndrome.
Graphical abstract
Effects of lutein and 3′-hydroxy-ε,ε-caroten-3-one on the differentiation of 3T3-L1 cells to adipocytes. Berberine chloride at 1 μg/mL (2.7 μM) was used as a positive control.
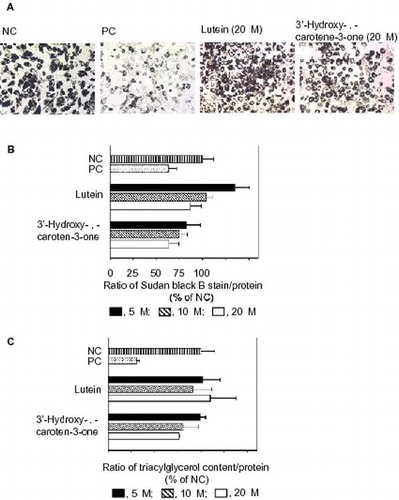
Key words:
Lutein, which is the major carotenoid present in green leafy vegetables, corn, and egg yolk, accumulates in human plasma and tissues, particularly the macula of the retina, at highly concentrated levels. It has been thought to work as an antioxidantCitation1) and as a blue light filterCitation2) to prevent eye diseases such as age-related macular degeneration. In addition to these preventive effects, lutein has been reported to show several biological activities including anti-cancer,Citation3) anti-inflammatory,Citation4) and anti-atherogenicCitation5) actions in cultured cells and experimental animals.
Not only carotenoids per se but also their metabolites are thought to have biological actions. For example, retinol, which is formed from β-carotene, α-carotene, and β-cryptoxanthin by a central cleavage enzyme, functions as vitamin A. Apo-10′-lycopenoic acid, which is thought to be a metabolite of lycopene generated by the actions of carotene 9′,10′-oxygenase, was reported to increase the expression of sirtuin 1 and to decrease hepatic steatosis of mice fed a high-fat diet.Citation6) We recently found that 3′-hydroxy-ε,ε-caroten-3-one (Fig. ) and ε,ε-caroten-3,3′-dione, which were converted from lutein by a dehydrogenase in mouse liver,Citation7) showed an inhibitory effect on nitric oxide (NO) production in RAW264 cells stimulated with lipopolysaccharide—namely, an anti-inflammatory action.Citation8) The metabolites were the predominant carotenoids in mice fed with lutein, and their amounts in liver reached approximately 2.5-fold the amount of unconverted lutein.Citation7) These results strongly suggest that lutein metabolites may be responsible for some part of the biological actions of lutein.
The anti-obesity actions of carotenoids have recently received a great deal of attention with a view to contributing to the prevention of metabolic syndrome, since diverse carotenoids such as fucoxanthin, neoxanthin, β-carotene, and β-cryptoxanthin have all been reported to inhibit the adipocyte differentiation of 3T3-L1 cells and thereby to reduce the cellular triacylglycerol (TG) production.Citation9–12) On the other hand, lutein was not reported to inhibit the adipocyte differentiation of 3T3-L1 cells.Citation10) If lutein metabolites contribute to the inhibitory effect, this will become a typical case of the metabolites showing biological actions. Hence, in the present study, to assess the possibility of the anti-obesity action of lutein metabolites, we evaluated the effect of 3′-hydroxy-ε,ε-caroten-3-one on the differentiation of 3T3-L1 cells.
Materials and methods
Materials
Lutein and its metabolite, 3′-hydroxy-ε,ε-caroten-3-one, were prepared as previously reported.Citation8) Sudan Black B was purchased from Tokyo Chemical Industry Co., Ltd. (Tokyo). Triglyceride (TG) E-test Wako, dexamethasone, glucose, and paraformaldehyde were purchased from Wako Pure Chemical Industries, Ltd. (Osaka). Insulin, 3-isobutyl-1-methylxanthine, and berberine chloride were purchased from Sigma-Aldrich (St. Louis, MO). Dulbecco’s Modified Eagle’s Medium (DMEM) was purchased from Nissui Pharmaceutical (Tokyo). The DMEM used was of a low glucose-type (1.0 g/L glucose), so we increased the glucose concentration to 4.5 g/L. Tetrahydrofuran (THF) was purchased from Nacalai Tesque, Inc. (Kyoto). THF was purified in a neutral alumina column just before use.
Cell culture
The mouse pre-adipocyte cell line 3T3-L1 was obtained from the American Type Culture Collection (Rockville, MD). The cells were incubated in a culture medium consisting of DMEM, 10% heat-inactivated fetal bovine serum, 4 mM L glutamine, 40 units/mL penicillin, and 40 μg/mL streptomycin at 37 °C in a humidified atmosphere of 5% CO2 in air. The cells were grown by passaging twice per week.
Differentiation, induction, and cell treatment with carotenoid
Cells were seeded in a 48-well plate at 1 × 104 cells per well; each well also contained 200 μL of culture medium. After reaching confluence, the cells were incubated for 3–4 days in the induction medium as described below. Thereafter, the cells were treated twice per week with the insulin medium as described below. The culture medium described above was supplemented with 10 μg/mL insulin, 0.5 mM 3-isobutyl-1-methylxanthine, and 0.25 μM dexamethasone and used as the induction medium for initiation of differentiation. 3-Isobutyl-1-methylxanthine and dexamethasone dissolved in dimethyl sulfoxide (DMSO) were added to the culture medium, and the final concentration of DMSO in the medium was 0.2% (v/v). The carotenoid medium as described below was supplemented with 10 μg/mL insulin, and used as the insulin media for the treatment with carotenoid.
Preparation of carotenoid medium
Media containing carotenoid were prepared by a liquid drying method as described previously.Citation13) In brief, the carotenoid dissolved in the purified THF was added to the culture medium. THF in the medium was dried in a centrifugal evaporator. The medium was passed through a 0.2-μm filter to sterilize, and then was used as the carotenoid medium. To determine the concentration of carotenoid, one part of the carotenoid medium was diluted 41-fold with dichloromethane/methanol (1:9, v/v) and subjected to HPLC analysis as described below.
Sudan Black B staining
After cell treatment as described above, the plates were kept on ice. The treated cells were washed with 0.2 mL of 10 mM taurocholate in PBS and then washed twice with PBS. The cells were then fixed with 4% paraformaldehyde for 1 h on ice. After washing twice in PBS, the fixed cells were stained with 0.03% (w/v) Sudan Black B/75% 2-propanol at room temperature for 20 min. Sudan Black B was used to analyze the intracellular production of oil droplets. The stained cells were observed with a microscope after washing three times with 40% 2-propanol. After these observations, Sudan Black B was extracted twice with 100 μL of 2-propanol. The extracts were transferred to a 96-well assay plate and then analyzed at 595 nm with a microplate reader (Tecan Group, Männedorf, Switzerland). Because we considered that berberine or carotenoid could have reduced the number of cells or inhibited cell growth, we normalized the obtained values according to the protein content of the cells, which were measured as follows. After extracting Sudan Black B, the cells were dried and then 100 μL of PBS was added to the dried cells. They were then homogenized with a probe-type sonicator (Ultra S, VP-5S; Taitec, Saitama), and the cell homogenate was collected to determine the protein content by the Lowry method.
Analyses of TG and carotenoid present in cells and medium
To evaluate the TG content in cells, the cells were treated and washed as described above, then homogenized in 200 μL of PBS with the probe-type sonicator. An aliquot (100 μL) of the cell homogenate was subjected to analysis with a commercial TG E-test WAKO kit. After incubation at 37 °C for 60 min, the supernatants of the reaction mixtures were transferred to a 96-well assay plate and then analyzed at 595 nm with a microplate reader. The obtained values were normalized to the protein content in the cells. An aliquot (40 μL) of the cell homogenate was taken to determine the protein content as described above.
To evaluate the amount of carotenoid uptake by cells, carotenoids were extractedCitation14) from an aliquot (50 μL) of the cell homogenate and then analyzed by HPLC as described below. Results were normalized to the protein content. To measure the residual amounts of carotenoid in medium at the end of incubation, aliquots of the medium after filtration were analyzed as described above.
HPLC analysis
Carotenoids were analyzed by HPLC (using an LC-20AT pump, an SPD-M10A photodiode array detector, and a CTO-10AS column oven at a constant temperature of 25 °C; Shimadzu, Kyoto, Japan) on an ODS-80Ts column (2.0 × 150 mm) with an ODS-S1 precolumn (2.0 × 10 mm; Tosoh, Tokyo). Solvent A was acetonitrile/methanol/water (75:15:10, v/v/v) containing 0.1% ammonium acetate, and solvent B was methanol/ethyl acetate (70:30, v/v) containing 0.1% ammonium acetate. An isocratic analysis was performed at a flow rate of 0.2 mL/min with solvent A/B (50:50, v/v). The carotenoids were quantified from the peak area at 450 nm for lutein and 440 nm for 3′-hydroxy-ε,ε-caroten-3-one using a calibration curve of the authentic standard.
All experiments using carotenoid were conducted under dim yellow light to minimize isomerization and the degradation of carotenoids by light irradiation.
Statistical analysis
The data were analyzed for homogeneity of variances by the Bartlett test. When homogenous variances were confirmed, the data were evaluated by one-way ANOVA, followed by the Tukey–Kramer test for the comparison of multiple groups and Student’s t-test for the comparison of two groups. The values underwent log transformation before the test, if necessary. P-values <0.05 were considered significant.
Semi-empirical molecular orbital calculation
The optimized structures of the end groups in carotenoids (lutein and 3′-hydroxy-ε,ε-caroten-3-one) and the heat of formation were calculated by AM1 semi-empirical methods in Chem3D Pro (CambridgeSoft, Cambridge, MA).
Results
Evaluation of oil drops in 3T3-L1 cells by Sudan Black B staining
Oil drops were formed inside the differentiated 3T3-L1 cells. Fig. (A) shows Sudan Black B staining of the oil drops in each group of treated cells under observation by a microscope. We determined the quantity of Sudan Black B as shown in Fig. (B). The positive control (PC) markedly reduced the formation of the oil drop, compared with the negative control (NC). Lutein at 5–20 μM and 3′-hydroxy-ε,ε-caroten-3-one at 5–10 μM did not reduce the production. However, 20 μM 3′-hydroxy-ε,ε-caroten-3-one did significantly reduce it.
Fig. 2. Effects of lutein and 3′-hydroxy-ε,ε-caroten-3-one on the differentiation of 3T3-L1 cells to adipocytes.
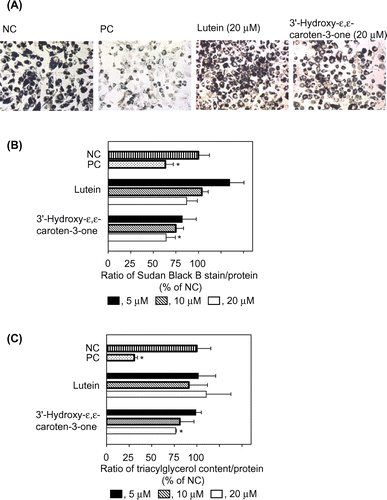
Evaluation of TG contents in 3T3-L1 cells
We also evaluated the effect of lutein and 3′-hydroxy-ε,ε-caroten-3-one on the differentiation of 3T3-L1 cells to adipocytes by measuring the TG contents. Similar to the results using Sudan Black B, the PC markedly reduced the TG contents, compared with the NC. Among the carotenoid treatments tested, only 3′-hydroxy-ε,ε-caroten-3-one at 20 μM significantly reduced the contents (Fig. (C)).
Calculation of end group structures of carotenoids
To predict the structures of 3-hydroxy β-end groups in lutein (Fig. (A)) and 3-oxo β-end groups in 3′-hydroxy-ε,ε-caroten-3-one (Fig. (B)), we simulated the conformations of these end groups by semi-empirical molecular orbital calculation using the AM1 method. The predicted stable structures were very different between these end groups. The calculated heat of formation are −47.66 kcal/mol for 3-hydroxy β-end group and −25.96/−26.35 kcal/mol for 3-oxo β-end group (6S/6R), respectively.
Evaluation of carotenoid amounts in 3T3-L1 cells and medium
Fig. (A) shows the levels of lutein and 3′-hydroxy-ε,ε-caroten-3-one that were accumulated in the treated cells. The accumulated levels of 3′-hydroxy-ε,ε-caroten-3-one were higher than those of lutein. The amounts of cellular carotenoids might be dependent on their oxidative stability in the medium during the incubation. However, the residual concentrations of 3′-hydroxy-ε,ε-caroten-3-one in medium (10.2 μM) after the second incubation at an initial concentration of 20 μM were significantly lower than that of lutein (13.7 μM) (Fig. (B)). Based on the calculation from their initial concentrations and the amounts of cellular accumulations, the carotenoid lost by oxidation in medium during the incubation were 28.8% for lutein and 36.0% for 3′-hydroxy-ε,ε-caroten-3-one, respectively, indicating that the oxidative stability of these carotenoids were similar. In addition, the formation of 3′-hydroxy-ε,ε-caroten-3-one from lutein was not found in cells and in the medium. Thus, the higher accumulation of 3′-hydroxy-ε,ε-caroten-3-one would be due to the high level of uptake by cells.
Fig. 4. Amounts of carotenoid in the cells and medium.
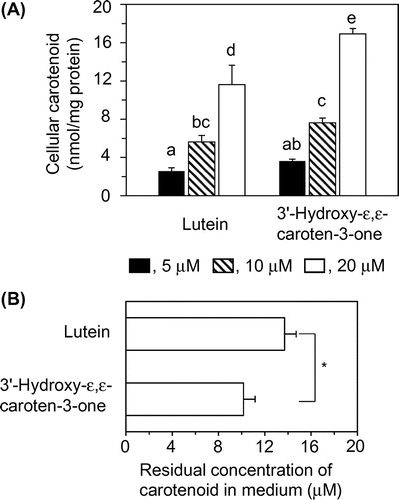
Discussion
In previous experiments to determine the anti-obesity action of carotenoids using 3T3-L1 cells, Oil-Red O has been used effectively as a staining reagent to detect oil droplets.Citation9–12,15) However, the carotenoid-accumulated cells turned red, which could create the mistaken impression that carotenoid was not effective at inhibiting the differentiation of 3T3-L1 cells. Thus, we used Sudan Black B, since its color is unaffected by the color of cellular carotenoid. The present study is the first to demonstrate that a lutein metabolite, 3′-hydroxy-ε,ε-caroten-3-one, significantly inhibited the adipocyte differentiation of 3T3-L1 cells, but lutein did not.
The structure of 3-oxo ε-end group of 3′-hydroxy-ε,ε-caroten-3-one, which was converted from 3-hydroxy β-end group of lutein, may participate in the inhibitory effect on the differentiation of 3T3-L1 cells. In agreement with previous studies that indicate the structures of 3-hydroxy ε-end group of lactucaxanthin and 3-hydroxy β-end group of lutein/zeaxanthin,Citation16) we found the conformation of 3-oxo ε-end group was very different from that of 3-hydroxy β-end group. The heat of formation value of the ε-end group was much higher than that of the β-end group, indicating that 3′-hydroxy-ε,ε-caroten-3-one had a highly reactive functional group. In fact, 3′-hydroxy-ε,ε-caroten-3-one has a Michael acceptor because an α,β-unsaturated carbonyl moiety is present in the ring structure, but lutein does not. Michael reaction acceptors are known to show strong biological activity.Citation17) 3′-Hydroxy-ε,ε-caroten-3-one and lutein have the same ring structure as 3-oxo-α-damascone and 3-hydroxy-β-damascone, respectively. 3-Oxo-α-damascone has been shown to more strongly inhibit inducible nitric oxide synthase (iNOS) than 3-hydroxy-β-damascone.Citation18) We recently reported that 3′-hydroxy-ε,ε-caroten-3-one inhibited the NO production and iNOS expression in RAW264 cells.Citation8) These results suggested an α,β-unsaturated carbonyl moiety in common with 3′-hydroxy-ε,ε-caroten-3-one and 3-oxo-α-damascone exhibited the effect.
In addition, anti-obesity effect has been reported in a study using an animal model. Lutein supplementation reduced the liver TG amounts in high-fat diet mice.Citation19) We previously found a remarkable accumulation of 3′-hydroxy-ε,ε-caroten-3-one in mice fed with lutein.Citation7) Thus, 3′-hydroxy-ε,ε-caroten-3-one transformed from lutein would exert an anti-obesity action. Hereafter, to definitively establish the mechanism underlying the effect of 3′-hydroxy-ε,ε-caroten-3-one, in addition to the chemical structural studies, biological mechanistic studies will be needed.
The higher cellular accumulation of 3′-hydroxy-ε,ε-caroten-3-one relative to lutein may also participate in the inhibitory effect on differentiation, since we previously found that the cellular lutein concentration and its anti-oxidant action in cells were lower than those of other carotenoids such as β-carotene, β-cryptoxanthin, and astaxanthin.Citation13) In contrast to this, other studies have reported that the accumulation of carotenoid in cells was not associated with biological activities such as anti-malignantCitation20) or anti-angiogenic effects.Citation21) Therefore, the association between cellular carotenoid accumulation and the anti-obesity action of 3′-hydroxy-ε,ε-caroten-3-one also deserves future study.
In conclusion, 3′-hydroxy-ε,ε-caroten-3-one, which is an oxidative metabolite of lutein, inhibited the differentiation of 3T3-L1 cells to adipocytes and the subsequent TG production, but lutein did not. The characteristic chemical structure of 3′-hydroxy-ε,ε-caroten-3-one participated in the inhibitory effect. Large amounts of carotenoid metabolites including 3′-hydroxy-ε,ε-caroten-3-one have been found to be accumulated in human serum. Thus, carotenoid metabolites would have potential as active substances to induce specific biological actions.
Authors contributions
E.K.-N. designed the study. E.K.-N. and M.H. performed the experiment. M.K. and A.N. contributed materials. E.K.-N. analyzed the data and wrote the manuscript. All authors contributed to the critical revision of the manuscript.
Disclosure statement
No potential conflict of interest was reported by the authors.
References
- Miller NJ, Sampson J, Candeias LP, et al. Antioxidant activities of carotenes and xanthophylls. FEBS Lett. 1996;384:240–242.10.1016/0014-5793(96)00323-7
- Junghans A, Sies H, Stahl W. Macular pigments lutein and zeaxanthin as blue light filters studied in liposomes. Arch. Biochem. Biophys. 2001;391:160–164.10.1006/abbi.2001.2411
- Ugocsai K, Varga A, Molnár P, et al. Effects of selected flavonoids and carotenoids on drug accumulation and apoptosis induction in multidrug-resistant colon cancer cells expressing MDR1/LRP. In Vivo. 2005;19:433–438.
- Oh J, Kim JH, Park JG, et al. Radical scavenging activity-based and AP-1-targeted anti-inflammatory effects of lutein in macrophage-like and skin keratinocytic cells. Mediators Inflamm. 2013;2013:787042.
- Dwyer JH, Navab M, Dwyer KM, et al. Oxygenated carotenoid lutein and progression of early atherosclerosis: the Los Angeles atherosclerosis study. Circulation. 2001;103:2922–2927.10.1161/01.CIR.103.24.2922
- Chung J, Koo K, Lian F, et al. Apo-10'-lycopenoic acid, a lycopene metabolite, increases sirtuin 1 mRNA and protein levels and decreases hepatic fat accumulation in ob/ob mice. J. Nutr. 2012;142:405–410.10.3945/jn.111.150052
- Yonekura L, Kobayashi M, Terasaki M, et al. Keto-carotenoids are the major metabolites of dietary lutein and fucoxanthin in mouse tissues. J. Nutr. 2010;140:1824–1831.10.3945/jn.110.126466
- Nagao A, Maoka T, Ono H, et al. A 3-hydroxy β-end group in xanthophylls is preferentially oxidized to a 3-oxo ε-end group in mammals. J. Lipid Res. 2015;56:449–462.10.1194/jlr.P055459
- Maeda H, Hosokawa M, Sashima T, et al. Fucoxanthin and its metabolite, fucoxanthinol, suppress adipocyte differentiation in 3T3-L1 cells. Int. J. Mol. Med. 2006;18:147–152.
- Okada T, Nakai M, Maeda H, et al. Suppressive effect of neoxanthin on the differentiation of 3T3-L1 adipose cells. J. Oleo Sci. 2008;57:345–351.10.5650/jos.57.345
- Lobo GP, Amengual J, Li HN, et al. Beta, beta-carotene decreases peroxisome proliferator receptor gamma activity and reduces lipid storage capacity of adipocytes in a beta, beta-carotene oxygenase 1-dependent manner. J. Biol. Chem. 2010;285:27891–27899.10.1074/jbc.M110.132571
- Shirakura Y, Takayanagi K, Mukai K, et al. β-Cryptoxanthin suppresses the adipogenesis of 3T3-L1 cells via RAR activation. J. Nutr. Sci. Vitaminol. 2011;57:426–431.10.3177/jnsv.57.426
- Jaswir I, Kobayashi M, Koyama T, et al. Antioxidant behaviour of carotenoids highly accumulated in HepG2 cells. Food Chem. 2012;132:865–872.10.1016/j.foodchem.2011.11.053
- Sugawara T, Kushiro M, Zhang H, et al. Lysophosphatidylcholine enhances carotenoid uptake from mixed micelles by Caco-2 human intestinal cells. J. Nutr. 2001;131:2921–2927.
- Lai CS, Tsai ML, Badmaev V, et al. Xanthigen suppresses preadipocyte differentiation and adipogenesis through down-regulation of PPARγ and C/EBPs and modulation of SIRT-1, AMPK, and FoxO pathways. J. Agric. Food Chem. 2012;60:1094–1101.10.1021/jf204862d
- Phillip D, Hobe S, Paulsen H, et al. The binding of Xanthophylls to the bulk light-harvesting complex of photosystem II of higher plants. A specific requirement for carotenoids with a 3-hydroxy-beta-end group. J. Biol. Chem. 2002;277:25160–25169.10.1074/jbc.M202002200
- Liu H, Talalay P. Relevance of anti-inflammatory and antioxidant activities of exemestane and synergism with sulforaphane for disease prevention. Proc. Natl. Acad. Sci. USA. 2013;110:19065–19070.10.1073/pnas.1318247110
- Gerhäuser C, Klimo K, Hümmer W, et al. Identification of 3-hydroxy-β-damascone and related carotenoid-derived aroma compounds as novel potent inducers of Nrf2-mediated phase 2 response with concomitant anti-inflammatory activity. Mol. Nutr. Food Res. 2009;53:1237–1244.10.1002/mnfr.v53:10
- Han H, Cui W, Wang L, et al. Lutein prevents high fat diet-induced atherosclerosis in ApoE-deficient mice by inhibiting NADPH oxidase and increasing PPAR expression. Lipids. 2015;50:261–273.10.1007/s11745-015-3992-1
- Bertram JS, Pung A, Churley M, et al. Diverse carotenoids protect against chemically induced neoplastic transformation. Carcinogenesis. 1991;12:671–678.10.1093/carcin/12.4.671
- Manabe Y, Hirata T, Sugawara T. Suppressive effects of carotenoids on the antigen-induced degranulation in RBL-2H3 rat basophilic leukemia cells. J. Oleo Sci. 2014;63:291–294.10.5650/jos.ess13169