Abstract
Sulfated polysaccharides (SPs) from Gracilaria fisheri of Thailand, which were extracted in low-temperature (25 °C) water showed the highest content of phenolic compounds compared with those extracted at high temperature (55 °C). Crude SP antioxidant activity was evaluated by measuring the DPPH free radical scavenging effect which is directly related to the level of phenolic compounds. The sulfate content, total sugar, and SPs yield were also directly related to the extraction temperature. All extracts contained galactose as a major monosaccharide. High antioxidant activity of crude SP, positively correlated with the phenolic compound contents (R2 = 0.996) contributed by the existence of sulfate groups and phenolic compounds. In purified SP, F1 fraction exhibited strong radical scavenging ability, but it was not significantly different compared to crude SP extracted at 25 °C. This indicated that the appropriate density and distribution of sulfate groups in the SP extract showed the best antioxidant activity.
Graphical abstract
Sulfated polysaccharides(SPs) of G. fisheri extracted with water at 25 °C is a new source of natural antioxidants, and purified SP fractions have potential antioxidant activity.
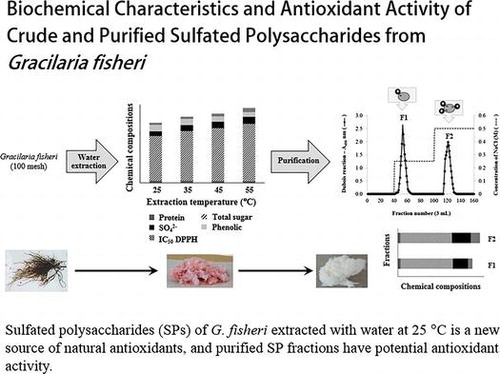
Seaweed is a rich source of natural non-animal sulphated polysaccharides; the chemical structure of these polymers varies according to the seaweed species.Citation1) Sulfated polysaccharides (SPs) are commonly found in three major divisions of seaweed as follows: green seaweed (Chlorophyta), brown seaweed (Phaeophyta), and red seaweed (Rhodophyta). Various isolated SPs from seaweed have been shown to have numerous beneficial biological effects, including antioxidant, anticoagulant, antitumor, antiviral, and anti-inflammatory.Citation2,3) The structural pattern of SPs was similar to the pattern of anionic polymers, such as glycosaminoglycans (GAGs), which were distributed among all of the organisms.Citation4)
Red seaweed diversely produced sulfated galactans, known as agar, and carrageenan, which are the major polysaccharides that compose cell matrix. However, the pattern of sulfate groups and the type of glycosidic linkage in the polysaccharides differ among the species. Polymers of galactans generally contain a linear backbone of an alternatively repeating unit of the 3-linked β-d-galactopyranose and the 4-linked α-galactopyranose. The α-galactose residues have d and l configuration in carrageenans and agars, respectively.Citation5) A portion of the α-galactose residues may exist as the 3,6-anhydro derivative, which has a repeating agarose backbone. The complex mixture of galactan units had a varying structure because various hydroxyl groups within the biopolymer could be substituted by sulfate ester, methyl ether, pyruvate acetal, or single-branching sugar residues.Citation6) Location and proportion of these substituents in galactan strongly affect the physicochemical and the biological activities.Citation7) The bioactive properties of SPs appear to be closely related to their molecular structure, such as substituted groups and position, types of sugars, molecular weight, charge characteristics, and chain conformations.Citation8) Moreover, SPs from red seaweed have high antioxidant activity demonstrating functions such as free radical scavengers and antioxidants for the delay or prevention of oxidative damage in living organisms.Citation9) It is well-known that the antioxidant activity of SPs directly depends on sulfate content, distribution of sulfate groups within the SP backbone, sugar composition, stereochemistry, and phenolic compounds found in the polysaccharide.Citation10,11) The antioxidant properties of carrageenans and ulvans appeared to be related to the sulfate content,Citation4) suggesting that SPs could be useful as non-toxic substances with potential antioxidant activity. Consequently, a suitable method of extraction was used for the efficient recovery of bioactive SPs with the desired functional properties.
Gracilaria fisheri red seaweed, distributed extensively along the southern part of Thailand’s coastlines, emerged as edible seaweed. The Gracilaria genus is an economically important marine seaweed because it is a worldwide source of agarophytesCitation12); this seaweed is typically found throughout the tropical regions of the world. SPs can be prepared by chemical, physical, or enzymatic treatments. Extraction of SPs is typically accomplished by solvent extraction processes employing methanol, ethanol, and acetone, and through water steam and/or distillation.Citation13) The solvent extraction methods have many disadvantages such as low selectivity, need for large amount of organic solvent, and emission of environmental pollution. Moreover, certain solvents are banned in the food industry, especially methanol. In response to these problems, an environmentally friendly SP extraction method from seaweed is water extraction. The biological activities attributed to the SPs of the Gracilaria genus, which are extracted with hot water (100 or 121 °C at 15 psi), include anticancer, anticoagulant, antiviral, and antioxidant activities.Citation14–17) In several reports, high temperatures have been used to extract agar but also less polar compounds.Citation18) When extraction temperature of 70–100 °C was applied, low content of sulfates was present in the SP extracts.Citation12) Sulfate contents of the polysaccharide extracts of Gracilaria sp. and G. fisheri in boiling water were 3.75 and 4.56%, respectively. On the other hand, these extracts showed poor quality due to their low sulfate content and different location of methyl group in the agar structure.Citation19) However, a low extraction temperature (25–28 °C) has been previously reported to increase the sulfate content. In the report by Wang et al.,Citation20) it appeared that the enhanced scavenging effect was related to high sulfate content, attributed to strong hydrogen donating ability. Effectiveness of polysaccharides extracted from G. corticata with water at 35–40 °C had antiviral activityCitation14) and the SPs extracted from G. birdiae with water at 25 °C exhibited antioxidant activityCitation16) was related to the sulfate level. Although the polysaccharides extraction of G. fisheri at high temperature focused on the chemical characteristics and gelling properties, the bioactive substances and antioxidant activities of crude SP extracts and purified SPs with low-temperature water have been limited.
The aim of this study was to extract the SPs from G. fisheri with water at low temperature (25–55 °C), characterize the chemical components of the crude SP extracts and purified SPs, and evaluate their antioxidant activity. The correlation between chemical composition (phenolic compound, total sugar, proteins, and sulfate content) and antioxidant activity was reported.
Materials and methods
Materials and chemicals
The Gracilaria fisheri (Xia et Abbott) Abbott, Zhang et Xia as an asexual structure was selected for this study. Seaweed structure is a thallus bush, with many branches coming from a short stipe; cylindrical branches; three to four irregularly located branches, constricted at bases and tapered toward apices. The seaweed was collected from a commercial seaweed pond in the Chiya District of the Surat Thani Province in the southern part of Thailand, in November 2011. The sample was washed with seawater, tap water, and distilled water and then air-dried. The dried seaweed was milled to a powder, passed through a 100-mesh sieve, and stored in a refrigerator at 4 °C. The seaweed material contained sulfate (8.47%); sugar components included galactose (28.19%) and small amounts of glucose and xylose (9.48 and 1.47%, respectively).
DEAE-Toyopearl 650 M was obtained from Tosoh Bioscience. Ascorbic acid, 1,1-diphenyl-2-picrylhydrazyl (DPPH), bovine serum albumin (BSA), butylated hydroxytoluene (BHT), gallic acid, and Folin–Ciocalteu reagent were purchased from Sigma Chemical Company. Dialysis membranes were produced by Orange Scientific Co., and the molecular weight cutoff was 6.0–8.0 Da. Standard monosaccharides, d-glucose, d-xylose, d-galactose, d-mannose, l-arabinose, and cellobiose were purchased from Sigma. Lichrosolv® water, and silica gel 60 plates were obtained from Merck. All of the chemicals were of analytical grade.
Extraction of SP from G. fisheri
To obtain a high yield of crude SPs that exhibited a high level of antioxidant activity, different temperatures were applied during the extraction process. The milled seaweed (9.0 g) was successively extracted with distilled water (600 mL) at 25–55 °C for 16 h under constant mechanical stirring. Separation of the residues from the extract was performed by centrifugation (9500g for 10 min). The supernatant from each extraction temperature was collected and precipitated by adding three volumes of 99.5% ethanol (v/v); it was stored at 4 °C overnight. Then, the precipitant was redissolved in distilled water, dialyzed with a membrane with a molecular weight cutoff of 6.0–8.0 kDa, and freeze-dried to obtain the crude SP.
Extraction yield
Extractable values were determined in terms of extract yield expressed on the percentage of dried basis and calculated according to the following equation:
Determination of total sugar in sulfated polysaccharides
The total sugar was evaluated according to the phenol-sulfuric acid method.Citation21) A sample solution (0.2 mL) was mixed with 0.2 mL phenol solution (5.0%, w/v), and 1.0 mL concentrated sulfuric acid was added to the reaction mixture. After incubating for 20 min at room temperature, the absorbance was measured at 490 nm with a microplate reader (Metertech Inc., Taiwan), and distilled water was used as a blank. The calculations were based on a calibration curve obtained with galactose. The results were expressed as galactose equivalents in milligrams per gram of extracts.
Monosaccharide composition analysis of SPs
Monosaccharide compositions of the crude SPs were obtained by acid hydrolysis according to National Renewable Energy Laboratory Analysis Procedure (LAP TP-510–42618).Citation22) Briefly, the ground sample (3 mg) was mixed with 3.0 mL of 72.0% H2SO4. The mixture was incubated at room temperature for 1 h and then 84 mL of distilled water were added to the reaction solution to dilute the acid concentration to 4.0%. Subsequently, the mixture was autoclaved at 121 °C for 1 h. The hydrolysate products were neutralized to pH 6.0–7.0 by adding CaCO3; subsequently, the CaCO3 precipitant was removed by filtration, and the supernatant was collected for analysis by high-performance liquid chromatography (HPLC) and thin-layer chromatography (TLC).
The HPLC analysis of the monosaccharide composition of the hydrolysis products was performed with a refractive index detector (Model RID-10A), a Shimadzu LC-20AD pump, and a column oven (CTO-20A) at 85 °C (Shimadzu corp., Japan). Monosaccharides were analyzed using an Aminex HPX-87P column (Bio-Rad). Lichrosolv® water served as the mobile phase with a flow rate of 0.6 mL/min. The samples (20 μL) were injected into the column using an autoinjector (SIL-20AHT). Monosaccharide content identification was performed by standard sugars, d-glucose, d-xylose, d-galactose, d-mannose, l-arabinose, and cellobiose. The monosaccharide contents were expressed as the molar content in the extracts (% mol).
The TLC analysis of the monosaccharide composition of the hydrolysis products was performed on a silica gel 60 plate, using a solvent of n-butanol-acetic acid-distilled water (2:1:1). Sugar spots were detected by heating the plate at 100 °C after spraying the plate with a mixture of 0.125 g α-diphenylamine, 0.125 mL aniline, 12.5 mL acetone, and 1.25 mL of 85.0% phosphoric acid.Citation23)
Determination of protein content in SPs
Protein content was measured as described by Lowry et al.Citation24) using BSA as a standard. The sample solution (0.1 mL) was mixed with 1.0 mL of biuret reagent, which was prepared by mixing 0.5 mL of 1.0% CuSO4 with 0.5 ml of 2% sodium potassium tartrate, followed by the addition of 50 mL of 2% Na2CO3 in 0.1 N NaOH. After incubation at room temperature for 10 min, 50 μL of 50.0% of Folin–Ciocalteu reagent was added and was maintained at room temperature for 30 min. Absorbance was measured at 660 nm with a microplate reader, and distilled water was used as a blank.
Determination of sulfate content in SPs
Sulfate content was determined by the turbidimetric method.Citation25) After acid hydrolysis of the sample (5 mg) with 0.2 mL of 1 M HCl for 8 h at 100 °C, the sulfate content was measured. A standard curve was prepared with a solution of K2SO4 (300–3,000 μg/mL). Then, 3.8 mL trichloroacetic acid (3.0% w/v) and 1.0 mL of a barium chloride-gelatin solution were added, mixed, and incubated at room temperature for 15 min. Absorbance was measured at 360 nm with a UV–visible spectrophotometer, and 0.2 mL of a hydrochloric acid solution was used as a blank.
Determination of phenolic contents in SPs
Phenolic content was determined by the Folin–Ciocalteu method.Citation26) The reaction mixture containing 100 μL of the extract (5 mg/mL) was mixed with 400 μL distilled water and 100 μL Folin–Ciocalteu reagent. After incubating at room temperature for 6 min, 1.0 mL of a 7.0% Na2CO3 solution was added. Finally, the reaction mixture was mixed thoroughly and placed in the dark for 90 min. The absorbance was measured at 760 nm with a UV–visible spectrophotometer, and distilled water (100 μL) was used instead of the extracts as a blank. Calculations were based on a calibration curve obtained with gallic acid. The total phenolic content was expressed as the gallic acid equivalent (GAE) in milligrams per gram of SP extracts.
Measurement of DPPH free radical scavenging activity of SPs
The free radical scavenging activity of the SPs from G. fisheri was determined using a stable free radical, DPPH, according to a slightly modified method of Blois.Citation27) The DPPH-scavenging potential of the extract was measured based on the scavenging ability of stable DPPH radicals by the SPs of G. fisheri. DPPH solution was prepared at the concentration of 2 × 10−4 M in 99.5% ethanol. During the assay, 300 μL of various concentrations of crude SPs (1–15 mg/mL) and 300 μL of freshly prepared DPPH solution were completely mixed. The reaction mixture was allowed to stand at the room temperature for 1 h in the dark. Then, the absorbance was recorded at 517 nm using a microplate reader. Butylated hydroxytoluene (BHT; IC50 = 0.05 mg/mL) and ascorbic acid (IC50 = 0.01 mg/mL) were used as positive controls. The results were expressed in terms of the percentage inhibition of DPPH-scavenging effect, which was calculated as follows:(1)
where Ai is the absorbance of the extract mixed with the DPPH solution, Aj is the absorbance of the same extract mixed with 300 μL ethanol, and Ac is the absorbance of the control with a particular solvent (using distilled water instead of the SP extract).
The results were expressed as IC50 values (Inhibition Concentration 50; the concentration of antioxidant that reduces the free radical DPPH by approximately 50.0%), which were determined from the linear section of the graph of the percentage of DPPH radical scavenging activity against the concentration of the extracts using the following trend line equation: [y = ax + b]. IC50 DPPH was calculated by transforming this equation and using the expression x where the y value is 50.0%.
Purification of SPs
The crude SP, obtained by water extraction at 25 °C, was subjected to an anion-exchange chromatography. The crude SP (400 mg) was dissolved in 3 mL of 50 mM NaOAc (pH 5.5) and applied to a DEAE-Toyopearl 650 M column (1 × 20 cm) equilibrated with the same solution. The column was prepared by a stepwise gradient of 0–3.0 M NaCl in the same solution at a flow rate of 0.8 mL/min. Each 3 mL fraction was collected, and the sugar content of the elution was determined by the phenol-sulfuric acid method. Finally, two elution peaks, F1 and F2, were obtained from DEAE-Toyopearl 650 M column, dialyzed with water, and freeze-dried. The purified SPs were analyzed for chemical properties, such as protein content, total sugar, sulfate content, and phenolic content, and their antioxidant activity. Monosaccharide compositions of purified SPs were analyzed by TLC and HPLC.
Statistical analysis
All of the experiments were conducted in triplicate (n = 3), and the experimental data were subjected to an analysis of variance for a completely randomized design. Significant differences between the mean values of the parameters were determined using the multiple range test (p ≤ 0.05). Correlation analysis was performed using Pearson’s correlation coefficient (r). Statistical analysis was performed using SPSS software (v.17, SPSS, USA).
Results and discussion
Effect of extraction temperature on yield of crude SPs
The procedure used for extraction and purification of SP from G. fisheri is shown in Fig. , while the extraction yield and chemical composition of the crude SPs obtained by water extraction at different temperatures are shown in Table . After the extraction, non-gelling polysaccharides were observed. The temperature of extraction had a significant effect on the yield (p ≤ 0.05), which ranged from 6.03% (25 °C) to 9.49% (55 °C) of seaweed dried weight. The highest yield was found in the extraction at 55°C, but the lower yields corresponded to the low-temperature extraction. Crude SP yield is related to the extraction temperature because the increment of the extraction temperature induced an increase of the internal energy of the molecules and the solubility of the substances, resulting in easily extracted SP.Citation28) In addition, high temperature (55 °C) could break the internal and external hydrogen bonds of the biopolymer with the consequent alteration in the structure features, allowing for the release of most polysaccharides.Citation29) Results revealed that the crude SP yield significantly increased with increased extraction temperature, which was also related to heat-induced increased solubility and diffusion of SP in water.Citation30) Extraction temperature of 40–55 °C also influences the bonds between the polymer chain and substituent groups, such as sulfate and methoxy groups, by rupturing and/or changing the location within the polymer.Citation31,32) Agar extraction temperature of Gracilaria genus has been reported as 70–100 °C,Citation12) but these temperatures decrease the amount of sulfate groups in the polymer chain. Different extraction temperatures significantly affected the agar yield of G. cliftonii.Citation29) A similar extraction temperature was used by Estevez et al.Citation33) who reported that the content of galactans extracted from Gymnogongrus torulosus was 5.10%, which was consistent with the study by Maciel et al.Citation34) in which the content of SP extracted from Brazilian G. birdiae was 6.50%. Although the extraction temperature affected the extractable yield of SP, the season and extraction method also affected SP yield. This result is consistent with the report of Praiboon et al.Citation19) who reported that the G. fisheri from Thailand extracted with boiling water exhibited the highest amount of polysaccharide (13.33%). Therefore, the yield of SP extract from the Gracilaria species is highly variable due to several factors, such as environmental conditions, seasonal variation, physiological factors, and extraction methods.Citation35)
Fig. 1. Schematic diagram of the extraction and purification of SP extracts from Gracilaria fisheri.
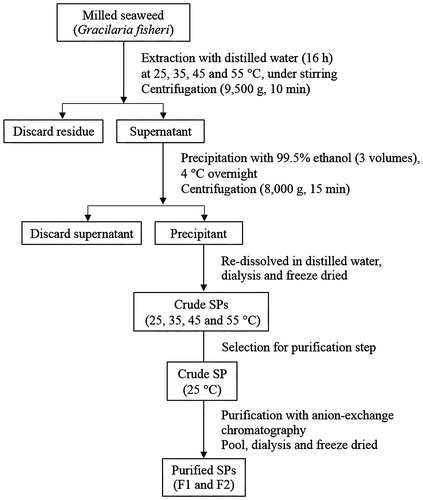
Table 1. Extraction yield, chemical compositions, and scavenging of the DPPH free radical by crude SP extracts obtained from Gracilaria fisheri with water extraction at different temperatures.
Effect of extraction temperature on chemical composition of crude SPs
Protein analysis of crude SPs
Protein content of crude SPs decreased from 3.33 to 2.73% when the extraction temperature increased from 25 to 55 °C (Table ). These results showed a significant difference in the proteins among the crude SPs at each temperature; however, extraction at low temperatures (25 and 35 °C) did not show a significant difference (p ≤ 0.05). Although the extraction temperature affected the crude SP yields, the protein content and the extractable yield exhibited a reverse variation. The lowest protein content was found in the crude SP extracted at 55 °C. It was probably due to the formation of protein–phenolic complexes, resulting in aggregation and ultimate precipitation of associated proteins.Citation36) Moreover, the proteins were partly degraded at high temperature. In fact, proteins are the major component of the cell wall or “cuticle” of seaweed, which are closely associated with SP.Citation37) It was noted that the proteins in crude SPs were not intracellular proteins. During the extraction, the SPs increased solubility and diffusion with increased extraction temperature which led to increased amount of free proteins in the water. By contrast, large free proteins tended to form complexes with anionic compounds; then, the precipitants were removed by the procedure of extraction. In addition, neutral polysaccharides, such as cellulose and xylan, could also limit the protein accessibility.Citation38) However, these values were in agreement with a previous report of Melo et al.Citation18)
Total sugar and monosaccharide composition of crude SPs
The level of total sugar in crude SP extracts obtained from different extraction temperatures is shown in Table . A high percentage of total sugar in the crude SP extracts significantly increased, ranging from 67.09 to 85.99% when the temperature increased from 25 °C to 55 °C. These data relatively corresponded to the extractable yield at each temperature. The monosaccharide composition of all extracts presented galactose as the dominant sugar, but a minor quantity of glucose was identified by TLC analysis (Fig. (A)). According to the analysis by HPLC, galactose was also the major sugar (83.05–87.31%) found in crude SPs, but minor quantities of glucose and xylose (2.00–6.10%) were also found, which was observed at all extraction temperatures, from 25 to 55 °C. These monosaccharides contained a major sugar that existed in seaweed material. In addition, galactose was commonly the major sugar component of polysaccharides synthesized by several species in the Gracilariales order.Citation18,39)
Fig. 2. TLC analysis of the monosaccharide composition in crude (A), and purified (B) SP extracts from Gracilaria fisheri.
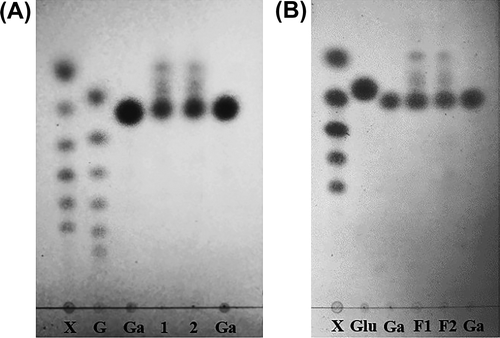
Sulfate content of crude SP
According to Table , crude SPs extracted at high temperature had higher sulfate content than those obtained at low extraction temperature. Sulfate contents ranged from 7.05% (25 °C) to 10.71% (55 °C) of dried extracts. An increase in the extraction temperature significantly affected the sulfate content (p ≤ 0.05). The results might be explained by the high extraction temperatures (44–55 °C) enhancing the polarity and solubility of SPs in water; the hydrogen bonds between hydrophilic polymer chains were ruptured but the sulfate groups were preserved.Citation29) Increase in sulfate content was consistent with the increased extractable yield. However, the high extraction temperature or boiling water (70–100 °C) significantly cleaved the sulfate group linkages and polysaccharides during the extraction,Citation32) which resulted in decreased sulfate content distributed in the extracts. Rodríguez et al.Citation40) reported that the sulfate content of polysaccharides extracted from G. gracilis at a room temperature was 13.70–16.80%, which was higher than that extracted at 90 °C (4.60–4.90%). These results suggested that low extraction temperature (<70 °C) responds to high sulfate content. The presence of sulfate groups in the polysaccharides has been found to be important for bioactive effects, such as antioxidant activity.Citation9)
Phenolic content of crude SPs
There was a statistically significant difference (p ≤ 0.05) between the extraction temperatures for the variability of total phenolic contents in crude SP extracts (Table ). Considerable variation was observed in the total amount of phenolics in all extracts, which ranged from 9.9 (25 °C) to 7.2 (55 °C) mg GAE/g extracts. However, phenolic contents found in crude SPs extracted at low temperature (25 and 35 °C) did not show a significant difference. Phenolic compounds are attached to hydroxyl groups of sugar within SP chains or proteins.Citation41) Thus, low temperature of water contributed to swelling of SPs allowing the SPs that adhered to phenolic compounds to be extracted easily. High-temperature extraction might have ruptured the associated compounds such as phenolics and proteins from the SP chains, resulting in the SP being extracted with smaller amount of associated compounds. The phenolic content of crude SPs was inversely related to the extraction temperature due to the formation of interactions between free proteins and phenolic compounds during the extraction, leading to aggregation and precipitation.Citation36) This result is consistent with the decrease in protein and phenolic contents of crude SP extracts when high extraction temperature was applied.
Effect of extraction temperature on antioxidant activity of crude SPs
DPPH is a free radical-generating compound that is used to evaluate the antioxidant effect of compounds in which the compounds act as free radical scavengers or hydrogen donors.Citation42) In this study, the DPPH free radical scavenging effect of the SP extracts was expressed as IC50 values. According to Table , the IC50 values of crude SPs at different temperatures exhibited significantly different scavenging activities against the DPPH system (p ≤ 0.05). The results from the crude SPs revealed that the DPPH radical scavenging activity increased in a concentration-dependent manner. The highest scavenging ability was found in the low-temperature crude SP extract (25 °C; IC50 = 3.07 mg/mL); however, lower SP activity was obtained by increasing the extraction temperature (55 °C). The observed different scavenging effect of DPPH radicals by the crude SP extracts might be due to their ability to increase the effective extraction of phenolic compounds and sulfate groups in these extracts. Antioxidant activity is found to be linearly proportional to phenolic contents, which have been shown to be potent DPPH radical scavengers.Citation10) This finding was consistent with the report by Wang et al.Citation9) who reported that the polysaccharide extract of red seaweed, Rhodomela condevoides, showed remarkable DPPH radical scavenging ability, which also correlated with phenolic contents. The polysaccharide extracts of G. birdiae and G. cornea exhibited excellent scavenging effects, as evaluated by the DPPH assay, and presented a corresponding amount of phenolic compounds.Citation43) The antioxidant activity of the phenolic compounds found in crude SPs resulted from their redox properties acting as reducing agents; they were also hydrogen donors and free radical quenchers.Citation44) However, several authors have suggested that the presence of sulfate groups in polysaccharides was also responsible for the antioxidant activity in which a high amount of sulfate groups distributed in SP had efficient scavenging activity, which was higher than that of a low sulfate content. Sulfate groups in crude SPs could activate the hydrogen atom of the anomeric carbon, thus affecting the strong hydrogen atom-donating capacity.Citation20) However, other components, such as low molecular weight polysaccharides, pigments, and proteins, could also affect the antioxidant activity.Citation10)
Correlation analysis
The correlation between antioxidant activity and the chemical composition, including the contents of phenolic compounds, sulfates, proteins, and sugar, of the crude SPs was analyzed as shown in Table . Negative correlation between sugar content and scavenging activity against DPPH (R2 = −0.995, p ≤ 0.01) was observed, which suggested that the types of sugars might promote the scavenging effect according to the preferred sugar content. It has been reported that sugar composition or the type of sugar was an important parameter influencing antioxidant capacity.Citation9,11) The correlation between sulfate content and the antioxidant effect was a reverse variation (R2 = −0.965, p ≤ 0.05). Among the various classes of SPs contributing to the potential antioxidant activities are fucoidanCitation45), carrageenansCitation46), and ulvansCitation4), it has been reported that this effect appeared to be related with the high sulfate content. However, a contrary result was observed in this study, which was in accordance with the report of Costa et al.,Citation1) revealing that the bioactivity depends on the distribution of sulfate groups in repeating units rather than the charge density.
Table 2. Pearson’s correlation coefficients (r) of antioxidant activity against DPPH and chemical compositions, phenolic compound, sulfate, total sugar, and protein.
A highly positive correlation was found between the phenolic contents and the scavenging activity against DPPH (R2 = 0.996, p ≤ 0.01). These results suggested that the antioxidant effect might be attributed to phenolic compounds. The protein content was positively correlated with antioxidant effect (R2 = 0.995, p ≤ 0.01). It might be due to the fact that amino group was a cation that could change the polarity of the compound and probably contributed to the effect on antioxidant activity.Citation9) However, proteins in crude SPs have considerably low content, which is rarely promoted for the scavenging activity.
In addition, the antioxidant effect of crude SP was also due to donation of a hydrogen atom that might be attributed to sulfate groups. Moreover, the presence of proteins and phenolic compounds in crude SP seems to hinder the hydrogen donating capacity of sulfate groups. The presence of NH3 and COOH groups on the protein chain could be interacted with negative charge of SP to form a stable electrostatic complex which limited the efficiency of the electrophile characteristic of sulfate groups.Citation47) These results show that the structural feature of the SP without attached compounds is important for the opportunity to elucidate their antioxidant activity, indicating that the purification of crude SP is needed to address these issues. Therefore, the crude SP extracted at 25 °C exhibiting high antioxidant activity, containing high contents of phenolics and proteins that possessed the appropriate features for high purification should be observed their functional properties.
Purification of SPs from G. fisheri
To provide purified and well-characterized SP extracts to elucidate their antioxidant effect, crude SP extracted at 25 °C was further purified by DEAE-diethylaminoethyl ion exchange chromatography (using a Toyopearl® DEAE-650M). The fractions were selected based on the total carbohydrate elution profile (Fig. ). The crude SP extract was primarily composed of two fractions, F1 and F2, which were eluted from the column with 0.25 and 0.5 M NaCl, respectively. The F2 fraction was eluted using higher concentration of eluent than that used to elute the F1 fraction; the F2 fraction contained a high charge density which was different from the F1 fraction. The two polysaccharide fractions were freeze-dried, and the yields of these fractions were 69.50 mg (F1) and 36.50 mg (F2).
Chemical composition of purified SP
Analytical characteristics of the two fractions (F1 and F2) are shown in Table . The purified fractions obtained by anion-exchange chromatography contained small amount of phenolic compounds and high proportion of sulfate content in both fractions compared with crude SP. These results implied that phenolic compounds were not linked to the polysaccharide chains. In addition, each fraction showed only a single and symmetrically sharp peak, indicating that the F1 and F2 fractions were pure. The total sugar of F1 and F2 was 56.33 and 55.63%, respectively. According to TLC analysis, the dominant monosaccharide composition in the two fractions was galactose (Fig. (B)). The HPLC analysis of F1 and F2 fractions also showed galactose as the major sugar; it was estimated at approximately 92.0 and 86.0%, respectively. However, small quantities of glucose (4.0%) and xylose (2.0%) were found in both fractions. The percentage of sulfate content was higher in the F2 fraction than that in the F1 fraction, which revealed that the purified SPs of both fractions contained different total negative charge density. From the results, the ratio of sulfate content/total sugar of the F2 fraction (0.34) was greater than that of the F1 fraction (0.29), whereas purified SP of the F2 fraction contained high charge density produced by the sulfate groups. DPPH free radical scavenging activity of the F1 fraction was significantly higher than that of the F2 fraction due to different distribution of sulfate groups in these polymers.Citation48) A similar observation has been reported for variety of SPs such as fucoidan (SP) from red seaweed, in which the sulfate groups substituted at different positions could induce different scavenging ability of the SP extract.Citation9) Both fractions (F1 and F2) had sulfate content higher than that of crude SPs, but only F1 fraction showed strong antioxidant activity which was not significantly different than for crude SP (25 °C). This indicated that the scavenging effect of crude SPs was affected by the existence of phenolic compounds, proteins, and sulfate groups by donating hydrogen and by their redox properties.Citation42) Although sulfate groups may act as an electrophile that can contribute to the strong hydrogen donation ability, the characteristic of sulfate groups was hindered by the attached compounds. After purification, the high sulfate groups tightly linked to the SP chains which exhibited high hydrogen donating ability, led to a potential antioxidant effect. Therefore, the purified SPs play an important role in absorbing and neutralizing free radicals, which are effective scavengers of free radicals by donating hydrogen.Citation20,49) This is in agreement with some previous studies of SP extracts from several sources.Citation50)
Table 3. Chemical compositions of crude SP extract (25 °C) of Gracilaria fisheri and purified fractions of SP obtained from anion-exchange chromatography on DEAE-Toyopearl 650 M.
In conclusion, water-extracted crude SPs contained proteins, sugars, phenolic compounds, and sulfates; however, the crude SPs extracted at low temperature (25 °C) had the highest level of phenolic compounds which had significant effects on the scavenging activity. Phenolic compounds and proteins were positively correlated with the antioxidant effect, but a negative correlation was found for the sulfate content and total sugar. After purification, the SP fractions revealed that the radical scavenging activity of the extracts was the action of sulfate substituents. This finding is a first report of crude SPs extracted from G. fisheri at low temperature; its purified SP showed highly potent antioxidant activity. The SPs from G. fisheri extracted by water at low temperature were easily and economically prepared, demonstrating their possible use as a rich source of natural antioxidants with potential applications in the food industry.
Author contributions
S.I. designed the experiments, carried out acquisition of data, statistical analysis, and prepared the manuscript. N.L. conceived of the research, assisted in the design and interpretation of experiments. N.L. and K.R. carried out critical revision of the manuscript for important intellectual content. C.T., P.P., and R.W. assisted in administrative, technical, or material support. All authors read and approved the final manuscript.
Disclosure statement
No potential conflict of interest was reported by the authors.
Funding
This work was supported by the Royal Golden Jubilee Ph.D. Program [grant number PHD/0274/2550] of the Thailand Research Fund; National Research University Project of Thailand, Office of the Higher Education Commission (2014–2015) of King Mongkut’s University of Technology Thonburi, Thailand.
Notes
Abbreviations: DPPH, 1,1-diphenyl-2-picrylhydrazyl: GAE, gallic acid equivalent; IC50, inhibition concentration 50; HPLC, high-performance liquid chromatography; SP, sulfated polysaccharide; TLC, thin-layer chromatography
References
- Costa LS, Fidelis GP, Cordeiro SL, et al. Biological activities of sulfated polysaccharides from tropical seaweeds. Biomed. Pharmacother. 2010;64:21–28.10.1016/j.biopha.2009.03.005
- Ngo D-H, Kim S-K. Sulfated polysaccharides as bioactive agents from marine algae. Int. J. Biol. Macromol. 2013;62:70–75.10.1016/j.ijbiomac.2013.08.036
- Patel S. Therapeutic importance of sulfated polysaccharides from seaweeds: updating the recent findings. Biotechnology. 2012;2:171–185.
- Qi H, Zhang Q, Zhao T, et al. Antioxidant activity of different sulfate content derivatives of polysaccharide extracted from Ulva pertusa (Chlorophyta) in vitro. Int. J. Biol. Macromol. 2005;37:195–199.10.1016/j.ijbiomac.2005.10.008
- Knutsen SH, Myslabodski DE, Larsen B, et al. A modified system of nomenclature for red algal galactans. Bot. Mar. 1994;37:163–169.
- Usov AI. Structural analysis of red seaweed galactans of agar and carrageenan groups. Food Hydrocoll. 1998;12:301–308.10.1016/S0268-005X(98)00018-6
- Freile-Pelegrín Y, Murano E. Agars from three species of Gracilaria (Rhodophyta) from Yucatán Peninsula. Bioresour. Technol. 2005;96:295–302.10.1016/j.biortech.2004.04.010
- Ye H, Wang K, Zhou C, et al. Purification, antitumor and antioxidant activities in vitro of polysaccharides from the brown seaweed Sargassum pallidum. Food Chem. 2008;111:428–432.10.1016/j.foodchem.2008.04.012
- Wang J, Zhang Q, Zhang Z, et al. Synthesized phosphorylated and aminated derivatives of fucoidan and their potential antioxidant activity in vitro. Int. J. Biol. Macromol. 2009;44:170–174.10.1016/j.ijbiomac.2008.11.010
- Devi KP, Suganthy N, Kesika P, et al. Bioprotective properties of seaweeds: in vitro evaluation of antioxidant activity and antimicrobial activity against food borne bacteria in relation to polyphenolic content. BMC Complem. Altern. M. 2008;8:1–11.
- Ghosh P, Adhikari U, Ghosal PK, et al. In vitro anti-herpetic activity of sulfated polysaccharide fractions from Caulerpa racemosa. Phytochemistry. 2004;65:3151–3157.10.1016/j.phytochem.2004.07.025
- Marinho-Soriano E, Bourret E. Polysaccharides from the red seaweed Gracilaria dura (Gracilariales, Rhodophyta). Bioresour. Technol. 2005;96:379–382.10.1016/j.biortech.2004.04.012
- Herrero M, Cifuentes A, Ibañez E. Sub- and supercritical fluid extraction of functional ingredients from different natural sources: plants, food-by-products, algae and microalgae: a review. Food Chem. 2006;98:136–148.10.1016/j.foodchem.2005.05.058
- Chattopadhyay K, Ghosh T, Pujol CA, et al. Polysaccharides from Gracilaria corticata: sulfation, chemical characterization and anti-HSV activities. Int. J. Biol. Macromol. 2008;43:346–351.10.1016/j.ijbiomac.2008.07.009
- Lee S-H, Athukorala Y, Lee J-S, et al. Simple separation of anticoagulant sulfated galactan from marine red algae. J. Appl. Phycol. 2008;20:1053–1059.10.1007/s10811-007-9306-0
- Souza BWS, Cerqueira MA, Bourbon AI, et al. Chemical characterization and antioxidant activity of sulfated polysaccharide from the red seaweed Gracilaria birdiae. Food Hydrocoll. 2012;27:287–292.10.1016/j.foodhyd.2011.10.005
- Zandi K, Tajbakhsh S, Nabipour I, et al. In vitro antitumor activity of Gracilaria corticata (a red alga) against Jurkat and molt-4 human cancer cell lines. Afr. J. Biotechnol. 2010;9:6787–6790.
- Melo MRS, Feitosa JPA, Freitas ALP, et al. Isolation and characterization of soluble sulfated polysaccharide from the red seaweed Gracilaria cornea. Carbohydr. Polym. 2002;49:491–498.10.1016/S0144-8617(02)00006-1
- Praiboon J, Chirapart A, Akakabe Y, et al. Physical and chemical characterization of agar polysaccharides extracted from the Thai and Japanese secies of Gracilaria. ScienceAsia. 2006;32:11–17.10.2306/scienceasia1513-1874.2006.32(s1).011
- Wang J, Guo H, Zhang J, et al. Sulfated modification, characterization and structure–antioxidant relationships of Artemisia sphaerocephala polysaccharides. Carbohydr. Polym. 2010;81:897–905.10.1016/j.carbpol.2010.04.002
- DuBois M, Gilles KA, Hamilton JK, et al. Colorimetric Method for Determination of Sugars and Related Substances. Anal. Chem. 1956;28:350–356.10.1021/ac60111a017
- Sluiter A, Hames B, Ruiz R, et al. Determination of structural carbohydrates and lignin in biomass. Golden (CO): Laboratory Analytical Procedures (LAP), National Renewable Energy Laboratory (NREL); 2012; p. 1–15.
- Sornyotha S, Kyu KL, Ratanakhanokchai K. Purification and detection of linamarin from cassava root cortex by high performance liquid chromatography. Food Chem. 2007;104:1750–1754.10.1016/j.foodchem.2006.10.071
- Lowry OH, Rosebrough NJ, Farr AL, et al. Protein measurement with the Folin phenol reagent. J. Biol. Chem. 1951;193:265–275.
- Dodgson KS, Price RG. A note on the determination of the ester sulphate content of sulphated polysaccharides. Biochem. J. 1962;84:106–110.10.1042/bj0840106
- Dewanto V, Wu X, Adom KK, et al. Thermal processing enhances the nutritional value of tomatoes by increasing total antioxidant activity. J. Agric. Food. Chem. 2005;50:3010–3014.
- Blois MS. Antioxidant determinations by the use of a stable free radical. Nature. 1958;181:1199–1200.10.1038/1811199a0
- Cacace JE, Mazza G. Mass transfer process during extraction of phenolic compounds from milled berries. J. Food Eng. 2003;59:379–389.10.1016/S0260-8774(02)00497-1
- Kumar V, Fotedar R. Agar extraction process for Gracilaria cliftonii (Withell, Millar, & Kraft, 1994). Carbohydr. Polym. 2009;78:813–819.10.1016/j.carbpol.2009.07.001
- Mohsen SM, Ammer ASM. Total phenolic contents and antioxidant activity of corn tassel extracts. Food Chem. 2009;112:595–598.10.1016/j.foodchem.2008.06.014
- Andriamanantoanina H, Chambat G, Rinaudo M. Fractionation of extracted Madagascan Gracilaria corticata polysaccharides: structure and properties. Carbohydr. Polym. 2007;68:77–88.10.1016/j.carbpol.2006.07.023
- Craigie JS, Jurgens A. Structure of agars from Gracilaria tikvahiae rhodophyta: location of 4-O-methyl-l-galactose and sulphate. Carbohyd. Polym. 1989;11:265–278.10.1016/0144-8617(89)90002-7
- Estevez JM, Fernandez PV, Kasulin L, et al. Chemical and in situ characterization of macromolecular components of the cell walls from the green seaweed Codium fragile. Glycobiology. 2009;19:212–228.
- Maciel JS, Chaves LS, Souza BWS, et al. Structural characterization of cold extracted fraction of soluble sulfated polysaccharide from red seaweed Gracilaria birdiae. Carbohydr. Polym. 2008;71:559–565.10.1016/j.carbpol.2007.06.026
- Armisen R. World-wide use and importance of Gracilaria. J. Appl. Phycol. 1995;7:231–243.10.1007/BF00003998
- Siriwardhana N, Kim K-N, Lee K-W, et al. Optimisation of hydrophilic antioxidant extraction from Hizikia fusiformis by integrating treatments of enzymes, heat and pH control. Int. J. Food Sci. Technol. 2008;43:587–596.10.1111/ifs.2008.43.issue-4
- Fuller MP, Gibor A. Microorganisms as digestors of seaweed cell walls. Hydrobiologia. 1987;151:405–409.10.1007/BF00046160
- Fleurence J. Seaweed proteins. Trends Food Sci. Tech. 1999;10:25–28.10.1016/S0924-2244(99)00015-1
- Mollet J-C, Rahaoui A, Lemoine Y. Yield, chemical composition and gel strength of agarocolloids of Gracilaria gracilis, Gracilariopsis longissima and the newly reported Gracilaria cf. vermiculophylla from Roscoff (Brittany, France). J. Appl. Phycol. 1998;10:59–66.10.1023/A:1008051528443
- Rodríguez MC, Matulewicz MC, Noseda MD, et al. Agar from Gracilaria gracilis (Gracilariales, Rhodophyta) of the Patagonic coast of Argentina – Content, structure and physical properties. Bioresour. Technol. 2009;100:1435–1441.10.1016/j.biortech.2008.08.025
- Farvin KHS, Jacobsen C. Phenolic compounds and antioxidant activities of selected species of seaweeds from Danish coast. Food Chem. 2013;138:1670–1681.10.1016/j.foodchem.2012.10.078
- Matthäus B. Antioxidant activity of extracts obtained from residues of different oilseeds. J. Agric. Food. Chem. 2002;50:3444–3452.10.1021/jf011440s
- de Sousa Oliveira Vanderlei E, de Araujo IWF, Quindere ALG, et al. The involvement of the HO-1 pathway in the anti-inflammatory action of a sulfated polysaccharide isolated from the red seaweed Gracilaria birdiae. Inflamm. Res. 2011;60:1121–1130.
- Athukorala Y, Kim KN, Jeon YJ. Antiproliferative and antioxidant properties of an enzymatic hydrolysate from brown alga, Ecklonia cava. Food Chem. Toxicol. 2006;44:1065–1074.10.1016/j.fct.2006.01.011
- Wang J, Zhang Q, Zhang Z, et al. Antioxidant activity of sulphated polysaccharide fractions extracted from Laminaria japonica. Int. J. Biol. Macromol. 2008;42:127–132.10.1016/j.ijbiomac.2007.10.003
- Rocha de Souza MC, Marques CT, Dore CMG, et al. Antioxidant activities of sulphated polysaccharides from brown and red seaweeds. J. Appl. Phycol. 2007;19:153–160.
- de Kruif CG, Tuinier R. Polysaccharide protein interactions. Food Hydrocoll. 2001;15:555–563.10.1016/S0268-005X(01)00076-5
- Zhang Z, Zhang Q, Wang J, et al. Chemical modification and influence of function groups on the in vitro-antioxidant activities of porphyran from Porphyra haitanensis. Carbohydr. Polym. 2010;79:290–295.10.1016/j.carbpol.2009.08.004
- Petitou M, Casu B, Lindahl U. 1976–1983, a critical period in the history of heparin: the discovery of the antithrombin binding site. Biochimie. 2003;85:83–89.10.1016/S0300-9084(03)00078-6
- Mulloy B. The specificity of interactions between proteins and sulfated polysaccharides. An. Acad. Bras. Ciênc. 2005;77:651–664.10.1590/S0001-37652005000400007