Abstract
Reconstitution of whole biosynthetic genes in Aspergillus oryzae has successfully applied for total biosynthesis of various fungal natural products. Heterologous production of fungal metabolites sometimes suffers unexpected side reactions by host enzymes. In the studies on fungal polyketides solanapyrone and cytochalasin, unexpected oxidations of terminal olefin of biosynthetic intermediates were found to give one and four by-products by host enzymes of the transformants harboring biosynthetic genes. In this paper, we reported structure determination of by-products and described a simple solution to avoid the undesired reaction by introducing the downstream gene in the heterologous production of solanapyrone C.
Graphical abstract
Heterologous production in Aspergillus oryzae.
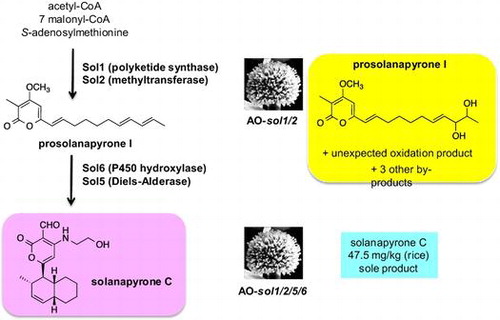
Heterologous expression of entire biosynthetic gene cluster of fungal natural products has become a practical method to study biosynthetic pathway and to produce natural products.Citation1,2) We successfully applied Aspergillus oryzae expression system to total biosynthesis of diterpene,Citation3) indole diterpenes,Citation4–6) polyketideCitation7) and to genome mining of novel metabolite.Citation8) During the projects on fungal metabolites, we have found unexpected reactions catalyzed by host, resulting in formation of by-products derived from intermediates accumulated in the transformants harboring the biosynthetic genes of two polyketide metabolites cytochalasin K (1) and solanapyrone A (2a). Here, we describe structure determination of modified intermediates and a method for suppressing undesired products.
Results and discussion
Tang and co-workers reported identification of the biosynthetic gene cluster of cytochalasin consisting of seven enzyme genes ccsA-G.Citation9) And they proposed the cytochalasin biosynthetic pathway as shown in Scheme . Recently, we have succeeded to isolate a linear intermediate analog 4 from the A. oryzae transformant AO-ccsAC harboring polyketide synthase-nonribosomal peptide synthetase (PKS-NRPS) and trans-acting enoyl reductase genes ccsA and ccsC.Citation10) 4 is most likely derived by 2-step reductions of 3 with reductases in A. oryzae although many other possibilities cannot be excluded. This strongly suggested that CcsA is capable for constructing the octaketide connected with phenylalanine in collaboration with CcsC and that the CcsA R domain catalyzes reductive cleavage of thio-tethered PKS-NRPS product. In this study, HPLC analysis of AO-ccsAC extracts also showed a large peak of structurally related metabolite 4A (Fig. ). UV and 1H NMR spectra were very similar to 4. HR-MS data of 4A showed molecular formula C28H41NO4 and in its 1H NMR spectrum, a hydroxymethyl signal was observed at 4.23 ppm in place of allylic methyl group at 1.58 ppm in 4, suggesting that 4A is an oxidation product of terminal methyl group. This was further supported by extensive 2D-NMR data. As we did not introduce any oxidation enzyme gene, 4A is most likely derived by oxidation of 4 with unknown monooxygenase in A. oryzae.
Fig. 2. HPLC profiles of the extracts from A. oryzae transformants; (i) AO-sol1; (ii) AO-sol1/2; (iii) AO-sol1/2/6; (iv) AO-sol1/2/6/5.
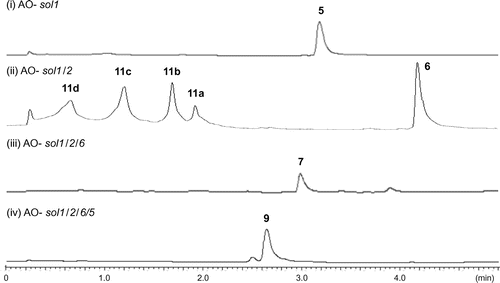
Fig. 4. Undesired oxidations of fungal metabolites found in heterologous expression of biosynthetic gene clusters in A. oryzae.
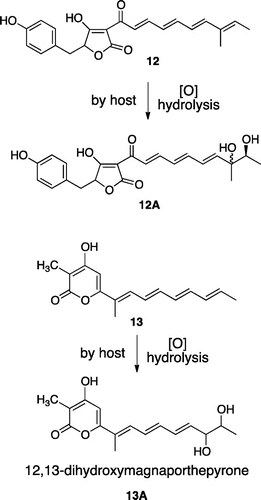
In parallel to cytochalasin biosynthesis, we investigated total biosynthesis of phytotoxins solanapyrones to examine the versatility of A. oryzae expression system. Solanapyrones (2a, 2b) were isolated as phytotoxins of a causal fungus Alternaria solani of potato early blight disease.Citation11,12) And later it was found that 2a showed a specific inhibitory activity against DNA polymerase β.Citation13) Based on co-isolation of chiral [4 + 2] cycloadducts 2a (exo) and 2b (endo), involvement of Diels–Alderase has been proposed (Scheme ).Citation14) Extensive studies with feeding experiments established the biosynthetic pathway via Diels–Alder reaction of prosolanapyrone III (8) giving 2a and 2b,Citation15,16) and detection of the enzymatic activity and purification of the enzyme solanapyrone synthase have been employed.Citation17–19) Recently, a biosynthetic gene cluster of solanapyrone has been identified.Citation20) This study showed involvement of four genes sol1 (PKS), sol2 (methyltransferase), sol6 (P450 monooxygenase), and sol5 (FAD-dependent oxidase, solanapyrone synthase), and functional analyses of two enzymes Sol1 and Sol5 were performed.Citation20) Based on this background, we started heterologous expression of four genes to produce solanapyrones.
Initial introduction using pTAex3 harboring sol1 in A. oryzae NSAR1 has successfully gave transformants AO-sol1. AO-sol1 produced desmethyl-prosolanapyrone I (5) in 120 mg/kg whose methylation product (Me2SO4, acetone) was identical to the synthetic prosolanapyrone I (6) (Fig. ).Citation21) To the AO-sol1, we introduced the second gene sol2 with a plasmid pPTRI-sol2. The resultant AO-sol1/2 produced 6 and significant amount of by-products 11a-11d (Fig. ). Based on their characteristic UV spectra, we speculated that all of them possessed 2-pyrone moiety derived from 6. Molecular formula C18H26O5 of a major product 11a suggested that 11a had the same carbon framework of 6. Compared its 1H NMR spectrum with that of 6, up-field shifted doublet methyl signal (1.13 ppm), and two oxymethine signals (3.85, 4.01 ppm) were newly observed in place of a terminal propenyl moiety in 6, suggesting that the terminal olefin in 6 was oxidized to a vicinal diol in 11a. Structure of 11a was further confirmed by several 2D-NMR data as shown in Fig. . Similarly, structures of three additional metabolites were determined in a similar manner as shown in the Fig. . Length of the side chain on the pyrone ring in 11b (C7), 11c (C5) and 11d (C3) suggested that putative C9-product derived by the oxidative cleavage of vic-diol in 11a further degraded by β-oxidation to give 11b-11d. Thus, we speculated that host monooxygenase oxidized terminal olefin in 6 to epoxide 10 in which hydrolysis underwent to give diol 11a as in the case of the total biosynthesis of paxilline.Citation4) Further oxidations were also likely catalyzed by oxidases in A. oryzae. Again, we faced a similar problem which occurred in the cytochalasin project.
To avoid the side reaction, we decided to introduce the downstream gene sol6. Our initial attempt to introduce sol6 to the AO-sol1/2 failed to produce prosolanapyrone II (7).Citation21) When we re-examined DNA sequence of gene cluster with a program 2ndFind which has recently developed for rapid the identification of natural product gene cluster, we found the previous prediction of sol6 intron was not correct.Citation20) Based on this observation, we prepared the plasmids pUSA-sol2 and pUSA2-sol2/6 which were subsequently introduced into AO-sol1 to yield AO-sol1/2 and AO-sol1/2/6. We gratifyingly found that the AO-sol1/2/6 gave the desired product 7 (12.5 mg/kg) as a single product that was identical to the authentic sample (Fig. ). AO-sol1/2 gave 6 and 11a (26 and 9.0 mg/kg) and prolonged fermentation increased the amount of 11a.
Finally, we introduce the last gene sol5 with pAdeA-sol5 and the resultant AO-sol1/2/6/5 afforded a new product (47.3 mg/kg) (Fig. ). Its molecular formula C19H25NO4 did not matched to those of 2a and 2b but solanapyrone C (9).Citation11,12) 1H NMR data of the new product were identical to 9 (Scheme ). Previous report showed treatment of 2a with ethanolamine gave 9 in quantitative yield.Citation12) Based on this result, we speculated relatively abundant ethanolamine present in A. oryzae reacted with 2a nonenzymatically to yield 9.
Our reconstitution study on solanapyrone biosynthetic genes in A. oryzae unambiguously confirmed the pathway proposed by the incorporation of isotopically labeled precursorsCitation15,16) and the subsequent gene cluster identification.Citation20) Previously, with the synthetic standard, we attempted to detect the intermediates 6–8 in the extracts from large-scale fermentation. We failed to detect any intermediate in the A. solani. This strongly suggested that the enzyme system for the solanapyrone biosynthesis extremely efficient to convert it to downstream endproduct solanapyrones. As in the case of A. solani, no intermediate was found in the all transformants of A. oryzae (Fig. ). Not like chemical synthesis, titer of intermediates increased in later steps (5:120 mg/kg; 6:26 mg/kg; 7:12.5 mg/kg; 9:47.3 mg/kg). The reason of this change is not obvious at this stage.
In this report, we described undesired oxidation of biosynthetic intermediate by the expression host A. oryzae in the biosynthesis of fungal polyketides cytochalasin and solanapyrone. Similar oxidation products 12A and 13A have been reported in the heterologous production in A. oryzae of desmethylbassianin (13)Citation22) and PKS-NRPS product 13 from Magnaporthe grisea.Citation23) In all cases including 1 and 2a/2b, oxidations occurred at the terminal of linear polyketide precursors 12 and 13 (Fig. ). Intriguingly, in the solanapyrone production, similar oxidation did not occur in structurally similar 5 and 7 but did in 6, suggesting that the corresponding monooxygenase in the formation of 11a showed strict substrate specificity in the pyrone moiety. In general, it is likely that several oxidation enzymes are responsible for the oxidations at terminal olefin of polyketide intermediates.
Regarding increasing importance of the A. oryzae expression system for producing the secondary metabolites,Citation24–26) the undesired oxidations found in our two projects could be a potential problem on expressing PKS-related genes. Therefore, we attempted to identify enzyme (most likely cytochrome P450) responsible for the oxidation in the transformant AO-sol1/2 using A. oryzae P450 library expressing in yeast which covered 121 out of putative 155 genes.Citation27,28) In these experiments, we found two yeast strains carrying CYP52H3 and CYP584G1 were able to give three new products from 6 in the LC-MS analysis (Fig. S1). Intriguingly, CYP52H3 and CYP584G1 showed significant sequence similarity. LC-MS analysis of the extracts from the transformants showed that molecular ion peaks of the new products did not match that of both epoxide and the corresponding diol 11a (Fig. S2). Based on these data, we speculated other monooxygenases in A. oryzae is responsible for the undesired oxidations.
In the solanapyrone biosynthesis, we faced a problem that accumulated intermediate 6 in AO-sol1/2 was oxidized into shunt products 11a–11d. This problem has been overcome by simply introducing the downstream gene sol6. Similar phenomena were observed in the biosynthesis of pyripyropenesCitation1) and paxillineCitation4) (Scheme ). In these cases, the epoxide intermediates 14/15 were readily converted into the corresponding diols 14A/15A by a host hydrolase. Introduction of downstream cyclase gene paxB/pyr4 completely suppressed the undesired diol formation. In case of conversion of intermediate 16 in the indole diterpene penitrem biosynthesis,Citation6) unidentified degradation of the putative intermediate 17 was suppressed in the transformant having downstream gene ptmI. Heterologous production of fungal metabolites sometimes suffered unexpected side reactions by host enzymes.Citation25,26) In this report, we have introduced a simple solution to avoid the undesired reaction by introducing downstream gene. We are currently working on the identification of downstream gene of ccsA/ccsC in the cytochalasin project, and its introduction to the AO-ccsAC for elucidating the biosynthetic pathway and the production of cytochalasin.
Experimental
Strains and culture condition
Alternaria solani ASP-2 was obtained from the Hokkaido National Agricultural Experimental Station, Sapporo. A. solani was cultivated in potato–glucose medium (200 g/L potato broth, 20 g/L of glucose) at 30 °C for the extraction of genomic DNA. Escherichia coli HST08 and DH5α were cultivated in Luria–Bertani (LB) medium for routine cloning and sequencing, while E. coli BL21-Gold (DE3) was utilized for the expression of recombinated proteins. Aspergillus oryzae NSAR1 (ΔArgB, sC−, niaD−, AdeA−) was cultivated in DPY medium (20 g/L of dexstrin monohydrate, 10 g/L of polypeptone, 5 g/L of yeast extract, and appropriate nutrients), MPY medium (30 g/L of maltose, 10 g/L of polypeptone, 5 g/L of yeast extract and appropriate nutrients) and rice medium (20–100 g polished rice, 0.1 g/kg of adenine) for transformation, detection of metabolites, and structure determination of new compounds, respectively. The appropriate nutrients are as follows: 0.6 g/L of arginine, 1.5 g/L of methionine, 9.25 g/L of ammonium sulfate, and 0.1 g/L of adenine were added to incubate the corresponding auxotrophic mutant.
Construction of expression plasmids of solanapyrone biosynthetic genes
The sol2, sol6, and sol6-rev genes were amplified from A. solani ASP-2 genomic DNA with the primer sets as shown in Table S1. Polymerase chain reaction (PCR) was performed with the KOD-Plus-Neo (TOYOBO). Each PCR product was inserted into appropriate restriction site to construct pTAex3-sol2, pUSA2-sol2, pUSA-sol6, pUSA2-sol6-rev, and pUSA2-sol2/sol6-rev.
The sol2 and sol5 gene flanked by PamyB and TamyB were amplified from pTAex3-sol2 and pTAex3-sol5 with primer sets as shown in Table S1. Each PCR product was inserted into appropriate restriction site to construct pPTR1-sol2 and pAdeA-sol5.
Purification of metabolites
The crude metabolites were purified by HPLC (Shimazu Class VP system) equipped with Wakopak navi C18-5 column (10 × 250 mm for preparation, 5 mm particular size) [flow rate of 3 mL/min, detection at 325 nm or 270 nm] using a linear gradient from 20 to 100% of CH3CN for 0–15 min and 100% CH3CN for 10 min.
Metabolite 4A from AO-ccsA/C (cytochalasin linear compound with terminal alcohol): 1.9 mg from the culture (1.2 kg). [α]D24.5 +1.0°(c 0.007, MeOH). 1H NMR (500 MHz, CD3OD) δH 7.37 (m, 1H), 7.28 (t, J = 6.5 Hz, 2H), 7.26 (d, J = 6.5 Hz, 2H), 7.12 (m, 1H), 6.28 (dd, J = 15.5, 9.6 Hz, 1H), 6.18 (d, J = 15.5 Hz, 1H), 6.10 (dd, J = 15.5, 9.6 Hz, 1H), 5.74 (dd, J = 15.5, 6.3 Hz, 1H), 5.61 (t, J = 6.4 Hz, 1H), 4.23 (d, J = 6.4 Hz, 2H), 4.16 (m, 1H), 3.55 (m, 2H), 3.32 (m, 2H), 2.95 (dd, J = 14.0, 6.5 Hz, 1H), 2.75 (dd, J = 14.0, 8.4 Hz, 1H), 2.45 (m, 2H), 2.08 (dd, J = 13.8, 6.3 Hz, 1H), 1.96 (dd, J = 13.8, 6.3 Hz, 1H), 1.81 (s, 3H), 1.62 (m, 1H), 1.50 (m, 1H), 1.47 (m, 1H), 1.31 (m, 1H), 1.11 (m, 2H), 0.86 (m, 3H), 0.84 (m, 3H); 13C NMR (125 MHz, CD3OD) δC 207.1, 169.1, 139.9, 136.0, 134.5, 133.5, 131.3, 130.5, 129.9, 129.6, 127.5, 64.2, 59.6, 54.5, 48.6, 45.5, 42.3, 41.4, 38.1, 32.3, 32.0, 31.0, 20.0, 19.8, 12.7. ESI–HR–MS (positive) calculated for C28H42NO4 [M + H]+ 456.3114, found 456.3161.
Metabolite 11a from AO-sol1/2: 9 mg from the culture (2 kg). [α]D24.2 −7.4°(c 0.32, CHCl3). 1H NMR (500 MHz, CDCl3) δH 6.70 (m, 1H), 5.98 (s, 1H), 5.97 (m, 1H), 5.74 (dt, J = 16.0, 6.9 Hz, 1H), 5.53 (dd, J = 16.0, 7.0 Hz, 1H), 4.01 (dd, J = 7.0, 4.0 Hz, 1H), 3.87 (s, 3H), 3.85 (m, 2H), 2.23 (dt, J = 13.0, 6.8 Hz, 2H), 2.09–2.38 (m, 2H), 1.93 (s, 3H), 1.46–1.67 (m, 2H), 1.46–1.54 (m, 2H), 1.13 (d, J = 7.0 Hz, 3H); 13C NMR (125 MHz, CDCl3) δC 157.4, 157.2, 139.3, 138.6, 134.8, 128.0, 122.1, 121.9, 94.3, 76.5, 70.1, 56.2, 32.5, 32.0, 28.5, 28.0, 17.7, 8.7. ESI–HR–MS (positive) calculated for C18H27O5 [M + H]+ 323.1853, found 323.1821.
Author contributions
R. F., A. M., and H.O. designed the research plan with assistance from I. F. and K. G. R. F., T. U., and T. K. performed research and analyzed data. M. H and H. I. designed and performed research for the P450 experiments. R. F., A. M., and H. O. prepared manuscript with assistance from all authors. All authors reviewed and approved the final manuscript.
Funding
This work was supported by the MEXT research grant on innovative area [grant number 22108002].
Supplemental materials
The supplemental material for this paper is available at http://dx.doi.org/10.1080/09168451.2015.1104234.
15BBB-solanapyrone-SI_final-925.pdf
Download PDF (2.7 MB)Disclosure statement
No potential conflict of interest was reported by the authors.
References
- Itoh T, Tokunaga K, Matsuda Y, et al. Reconstitution of a fungal meroterpenoid biosynthesis reveals the involvement of a novel family of terpene cyclases. Nat. Chem. 2010;2:858–864.
- Heneghan MN, Yakasai AA, Halo LM, et al. First Heterologous Reconstruction of a Complete Functional Fungal Biosynthetic Multigene Cluster. ChemBioChem. 2010;11:1508–1512.
- Fujii R, Minami A, Tsukagoshi T, et al. Total biosynthesis of diterpene aphidicolin, a specific inhibitor of dna polymerase α: heterologous expression of four biosynthetic genes in Aspergillus oryzae. Biosci. Biotecshnol. Biochem. 2011;75:1813–1817.
- Tagami K, Liu C, Minami A, et al. Reconstitution of biosynthetic machinery for indole-diterpene paxilline in Aspergillus oryzae. J. Am. Chem. Soc. 2013;135:1260–1263.
- Tagami K, Minami A, Fujii R, et al. Rapid reconstitution of biosynthetic machinery for fungal metabolites in Aspergillus oryzae: total biosynthesis of aflatrem. ChemBioChem. 2014;15:2076–2080.
- Liu C, Tagami K, Minami A, et al. Reconstitution of biosynthetic machinery for the synthesis of the highly elaborated indole diterpene penitrem. Angew. Chem. Int. Ed. 2015;54:5748–5752.
- Ugai T, Minami A, Fujii R, et al. Heterologous expression of highly reducing polyketide synthase involved in betaenone biosynthesis. Chem. Commun. 2015;51:1878–1881.
- Chiba R, Minami A, Gomi K, et al. Identification of ophiobolin f synthase by a genome mining approach: a sesterterpene synthase from Aspergillus clavatus. Org. Lett. 2013;15:594–597.
- Qiao kJ, Chooi YH, Tang Y. Identification and engineering of the cytochalasin gene cluster from Aspergillus clavatus NRRL 1. Metab. Eng. 2011;13:723–732.
- Fujii R, Minami A, Gomi K, et al. Biosynthetic assembly of cytochalasin backbone. Tetrahedron Lett. 2013;54:2999–3002.
- Ichihara A, Tazaki H, Sakamura S. Solanapyrones A, B and C, phytotoxic metabolites from the fungus Alternaria solani. Tetrahedron Lett. 1983;24:5373–5376.
- Oikawa H, Yokota T, Sakano C, et al. Solanapyrones, phytotoxins produced by Alternaria solani: biosynthesis and isolation of minor components. Biosci. Biotech. Biochem. 1998;62:2016–2022.
- Mizushina Y, Kamisuki S, Kasai N, et al. A plant phytotoxin, salanapyrone A, is an inhibitor of DNA polymerase β and λ. J. Biol. Chem. 2002;277:630–638.
- Oikawa H, Yokota T, Ichihara A, et al. Structure and absolute configuration of solanapyrone D: a new clue to the occurrence of biological Diels–Alder reactions. J. Chem. Soc. Chem. Commun. 1989;1284–1285.
- Oikawa H, Suzuki Y, Naya A, et al. First direct evidence in biological Diels–Alder reaction of incorporation of Diene-Dienophile precursors in the biosynthesis of solanapyrones. J. Am. Chem. Soc. 1994;116:3605–3606.
- Oikawa H, Suzuki Y, Katayama K, et al. Involvement of Diels–Alder reactions in the biosynthesis of secondary natural products: the late stage of the biosynthesis of the phytotoxins solanapyrones. J. Chem. Soc. Perkin Trans. 1999;1:1225–1232.
- Oikawa H, Katayama K, Suzuki Y, et al. Enzymatic activity catalysing exo-selective Diels–Alder reaction in solanapyrone biosynthesis. J. Chem. Soc., Chem. Commun. 1995;1321–1322.
- Katayama K, Kobayashi T, Oikawa H, et al. Enzymatic activity and partial purification of solanapyrone synthase: first enzyme catalyzing Diels–Alder reaction. Biochim. Biophys. Acta. 1998;1384:387–395.
- Katayama K, Kobayashi T, Chijimatsu M, et al. Purification and N-terminal amino acid sequence of solanapyrone synthase, a natural Diels–Alderase from Alternaria solani. Biosci. Biotechnol. Biochem. 2008;72:604–607.
- Kasahara K, Miyamoto T, Fujimoto T, et al. Solanapyrone synthase, a possible Diels–Alderase and iterative type I polyketide synthase encoded in a biosynthetic gene cluster from Alternaria solani. ChemBioChem. 2010;11:1245–1252.
- Oikawa H, Kobayashi T, Katayama K, et al. Total synthesis of (−)-solanapyrone a via enzymatic Diels−Alder reaction of prosolanapyrone. J. Org. Chem. 1998;63:8748–8756.
- Heneghan MN, Yakasai AA, Williams K, et al. The programming role of trans-acting enoyl reductases during the biosynthesis of highly reduced fungal polyketides. Chem. Sci. 2011;2:972–979.
- Song Z, Bakeer W, Marshall JW, et al. Heterologous expression of the avirulence gene ACE1 from the fungal rice pathogen Magnaporthe oryzae. Chem. Sci. 2015;6:4837–4845.
- Matsuda Y, Wakimoto T, Mori T, et al. Complete biosynthetic pathway of anditomin: nature’s sophisticated synthetic route to a complex fungal meroterpenoid. J. Am. Chem. Soc. 2014;136:15326–15336.
- Yakasai AA, Davison J, Wasil Z, et al. Nongenetic reprogramming of a fungal highly reducing polyketide synthase. J. Am. Chem. Soc. 2011;133:10990–10998.
- Wasil Z, Pahirulzaman KAK, Butts C, et al. One pathway, many compounds: heterologous expression of a fungal biosynthetic pathway reveals its intrinsic potential for diversity. Chem. Sci. 2013;4:3845–3856.
- Nazmul Hussain Nazir KH, Ichinose H, Wariishi H. Molecular characterization and isolation of cytochrome P450 genes from the filamentous fungus Aspergillus oryzae. Arch. Microbiol. 2010;192:395–408.
- Nazumul Hussain Nazir, Ichinose H, Wariishi H. Construction and application of a functional library of cytochrome P450 monooxygenases from the filamentous fungus Aspergillus oryzae. Appl. Environ. Microb. 2011;77:3147–3150.