Abstract
Colonic hydrogen (H2) protects against inflammation-induced oxidative stress. We examined the effect of a new highly branched α-glucan, isomaltodextrin (IMD), on colonic H2 production in rats. Rats were fed a 16.7% IMD, 8.8% indigestible dextrin (ID), or 10.4% high amylose cornstarch diet (Expt. 1), were fed diets containing 3.3–16.7% IMD (Expt. 2), or were fed diets containing 16.7% IMD or 5.2% fructooligosaccharide (FOS) (Expt. 3), for 14 days. Compared with the control group, feeding IMD or other α-glucans dose dependently and significantly increased H2 excretion and portal H2 concentration. The ability of IMD to increase H2 production was not inferior to that of FOS. The cecal Firmicutes/Bacteroidetes ratio in the IMD group was 5–14% of that in the control group. The cecal abundance of bifidobacteria was significantly greater in the IMD group than in the control group. Taken together, IMD, as well as other α-glucans, significantly increased colonic H2 production in a dose-dependent manner.
Graphical abstract
Isomaltodextrin(IMD), a highly branched α-glucan, dose dependently increases colonic H2 generation and decrease cecal Firmicutes(F)/Bacteroidetes(B) ratio.
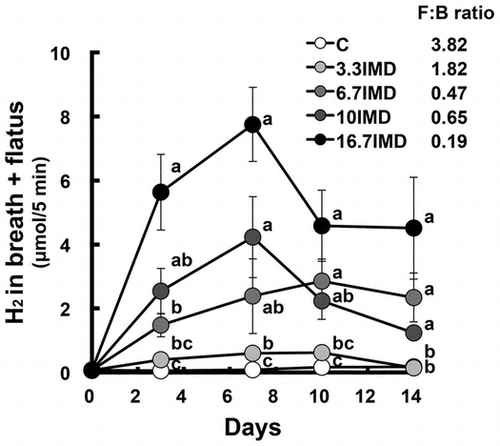
Nondigestible saccharides (NDSs), such as oligosaccharides, dietary fiber, and resistant starch promote colonic fermentation, which then generates large amounts of gases and short-chain fatty acids (SCFA).Citation1,2) Many investigators have studied various physiological effects of fermentation products. Colonic fermentation with low gas production has been considered to be useful because excessive gas production in the large intestine by administration of NDSs, such as oligosaccharides, dietary fiber, and resistant starch, causes flatulence and bloating.Citation3–5) However, we previously found that molecular hydrogen (H2) generated by colonic fermentation of high amylose cornstarch (HAS) and pectin increased portal H2 concentration and then reduced hepatic oxidative stress.Citation6) Also, we demonstrated the localization of colonic H2 to the adipose tissue due to diffusion into the abdominal cavity and suggested the reduced mRNA abundance of adipocytokine due to colonic H2.Citation7) Therefore, NDSs that can enhance colonic H2 generation would be of significant benefit because excessive oxidative stress can lead to the onset and progression of various metabolic syndromes.
The physiological effect of only a few α-glucans, such as indigestible dextrin (ID) and resistant starch through colonic fermentation, has been examined because most α-glucans are generally almost completely digested by α-amylase in saliva and pancreatic juice and digestive enzymes in brush border membranes such as maltase, isomaltase, trehalase, glucoamylase, and limit dextrinase. The digestibility of saccharides is influenced by its structure including the degree of branch linkage and crystallinity. Therefore, α-glucans that have a unique structure could be partially nondigestible. Also, the utilization of NDSs in the large intestine is affected by bacterial enzyme activity and composition of microbiota as well as solubility of NDSs.
Isomaltodextrin (IMD) is a novel, highly branched, soluble α-glucan with a relatively low degree of polymerization (DP); it is enzymatically produced from maltodextrin and is resistant to amylase (Supplemental Fig. 1).Citation8) This glucan partially escapes digestion in the small intestine and then could undergo fermentation by bacteria in the large intestine, and therefore, it may play a role as a prebiotic. However, no study has demonstrated its ability as a fermentation substrate in the large intestine. ID, which is chemically made from starch and is also a soluble glucan with low DP, promotes fermentation in the large intestine. However, strictly speaking, ID is not a pure α-glucan because ID has a small proportion of β-glycosidic linkage.Citation9) It has been reported that breath H2 excretion increased upon oral ingestion of ID in healthy subjects, although breath H2 excretion increased by a greater extent upon ingestion of fructooligosaccharide (FOS), which is one of the fructans.Citation10) Unlike IMD and ID, HAS is an insoluble α-glucan with a high DP.
The fermentation rate of NDSs in the large intestine is likely influenced by the monosaccharide composition, glycosidic linkages between the monosaccharide residues, and the DP of the particular NDS.Citation11,12) Most bacteria preferentially utilize glucose as a source of carbohydrates; therefore, we assumed that a large supply of α-glucan to the large intestine could induce high production of H2 in the large intestine. In the present study, we examined whether the pure, soluble, branched α-glucan, IMD, enhances colonic H2 production and whether IMD feeding dose dependently increases colonic H2 production.
Materials and methods
Samples
Isomaltodextrin, indigestible dextrin (Fibersol-2), HAS (Hi-maize 1043), and FOS (Meioligo-P) were kindly supplied by Hayashibara Co., Ltd. (Okayama, Japan), Matsutani Chemical Industry Co., Ltd. (Hyogo, Japan), Nippon NSC Ltd. (Tokyo, Japan) and Meiji Food Materia Co., Ltd. (Tokyo, Japan), respectively.
Measurement of properties of isomaltodextrin and indigestible dextrin
The number average molecular weight (Mn), weight average molecular weight (Mw), molecular weight distribution (Mw/Mn) and average DP in IMD and ID were determined using an HPLC apparatus equipped with a TSKgel α-M (13 μm, 7.8 × 300 mm, Tosoh, Tokyo, Japan) column with a refractive index detector.
Animals and diets
The study was approved by Nayoro City University Animal Care and Use Committee, and the animals were maintained in accordance with guidelines for the care and use of laboratory animals, Nayoro City University (approval numbers: 13–02 and 14–02). Male Sprague-Dawley rats weighing 260–280 g (8-week old) were obtained from Japan SLC (Haruno colony; Shizuoka, Japan). They were housed in individual cages made of screen bottoms of stainless steel in a room maintained at 23 ± 1 °C with humidity ranging from 50 to 70%, under lighting conditions of 12-h light/12-h darkness (07.00–19.00 h) daily. The rats were acclimatized by feeding a 25% casein control (C) diet for 3 days. The C diet was composed of 250 g/kg casein, 482.5 g/kg cornstarch, 100 g/kg sucrose, 70 g/kg soybean oil, 35 g/kg AIN-93G mineral mix,Citation13) 10 g/kg AIN-93 vitamin mix,Citation13) 2.5 g/kg choline bitartrate, and 50 g/kg cellulose.
Experiment 1
To determine the amount of hydrogen generated from various glucans in the large intestine, we examined the changes in H2 production in rats fed a diet containing glucans. After the acclimation period, (breath + flatus) H2 excretion over a 5-min period was measured by placing the rat inside a sealed polypropylene chamber for 5 min. After 5 min, an appropriate volume sample of the gaseous phase was withdrawn using a gas-tight syringe, and gas chromatography (GC) (Biogas analyzer BAS-1000; Mitleben, Osaka, Japan) was used to determine the amount of (breath + flatus) H2 excretion. The H2 excretion per 5 min was calculated by using the standard curve produced from peak height of standard H2 gas. These rats were further assigned into four groups (n = 8 per group) based on (breath + flatus) H2 excretion and body weight. Each group was administered the C diet supplemented with or without NDS for 10 d. Considering the digestibility of NDSs in vivo, which is 70%, 43%, and 52% for IMD, ID, and HAS, respectively (unpublished data), NDS was added to the C diet in such a way as to be able to deliver equal amounts to the large intestine; 16.7% IMD, 8.8% ID, or 10.4% HAS was supplemented to the C diet. It was not confirmed that equal amounts of NDSs were delivered to the large intestine in this study because rats with the large intestine were used. The supplementation of NDS was achieved by replacement of an equal weight of cornstarch in the C diet. The determination of the H2 excretion was carried out on day 3, 7, 10 and 14.
Experiment 2
To determine the dose response of IMD on H2 production in the large intestine, we examined the changes in (breath + flatus) H2 excretion and portal H2 concentration in rats fed a diet containing different amounts of IMD. After the acclimation period, (breath + flatus) H2 excretion over a 5-min period was measured using the same method as in Experiment 1. The rats were assigned into five groups (n = 8 per group) based on (breath + flatus) H2 excretion and body weight. Each group was administered the C diet supplemented with or without 3.3%, 6.7%, 10.0%, or 16.7% IMD, which is equal to 1%, 2%, 3%, or 5% as amounts of the nondigestible fraction to be supplied to the large intestine, respectively, for 14 days. IMD supplementation was achieved by the replacement of an equal weight of cornstarch in the C diet. The determination of the H2 excretion was carried out on day 3, 7, 10, and 14.
Experiment 3
To compare H2 generation in the large intestine by IMD feeding with that by FOS feeding, the latter of which leads to high generation of H2, we examined the differences in (breath + flatus) H2 excretion and portal H2 concentration in rats administered IMD or FOS. After the acclimation period, (breath + flatus) H2 excretion over a 5-min period was measured using the same method as earlier. The rats were assigned into three groups (n = 8 per group) based on (breath + flatus) H2 excretion and body weight. Each group was administered the C diet supplemented with or without 16.7% IMD or 5.2% FOS for 10 days. As Experiment 1, IMD and FOS were added to the C diet in such a way as to be able to deliver equal amounts to the large intestine. IMD or FOS supplementation was achieved by the replacement of an equal weight of cornstarch in the C diet. The determination of the H2 excretion was carried out on day 3, 7, 10, and 14.
Sampling
On the end of the experiment, rats were anesthetized with sodium penthobarbital (50 mg/kg body weight). After immediate laparotomy, 1 ml of blood was removed from the portal vein into sealed heparin vials for H2 analysis. Also, ca. 1 g of perirenal adipose tissue was immediately removed into sealed vials for H2 analysis. An appropriate volume sample of the gaseous phase was withdrawn using a gas-tight syringe, and H2 concentration was determined using GC (Biogas analyzer BAS-1000; Mitleben). The cecum was removed and weighed, and the cecal content was collected and stored at −80 °C in airtight tubes until SCFA, bacterial DNA and bacterial enzyme analysis. The cecal wall was blotted and weighed after rinsing in an ice-cold saline solution.
Quantitative PCR of microbial cecal content
Bacterial DNA was isolated from frozen cecal contents using a DNA extraction kit (ISOFECAL for Beads Beating kit, Nippon Gene Co., Ltd., Tokyo, Japan) according to the manufacturer’s protocol, except for sample disruption. Frozen cecal contents (200 mg) were disrupted at 2700 rpm for 90 s using a Multi-Beads shocker (MB601U, Yasui Kikai Co, Osaka, Japan). Extracted DNA samples were dissolved in DNase-free, TE buffer (pH 8.0; 10 mM Tris-HCl, 1 mM EDTA) and stored at −30 °C until quantitative PCR analyses. The PCR was performed in a total volume of 20 μl. All bacteria were detected using the SYBR Premix Ex Taq II (Takara), with 200 nmol/l each of respective primers and <1 ng DNA for each reaction. The primers used to detect the bacteria were based on 16S rRNA gene sequences.Citation7,14) The PCR amplification reactions were carried out as follows: 2 min at 50 °C, 3 min at 95 °C, followed by 40 cycles of 15 s at 95 °C and 1 min at 60 °C. Melting curve analysis was performed after amplification. Detection was carried out on an Agilent Mx3000P (Agilent Technologies). Tenfold serial dilutions of known quantities of TA cloning vector (pCR2.1-TOPO, Life Technologies) with inserted 16S rDNA gene sequences from Lactobacillus acidophilus (JCM1132) and Bacteroides vulgatus (JCM5826) were used to generate standard curves in order to count copies of total bacteria and Firmicutes, and Bacteroidetes, respectively. Absolute rDNA abundance was calculated based on the standard curves. Each assay was performed in duplicate in the same analysis.
SCFA analysis
After homogenization of cecal contents, cecal organic acids (acetate, propionate, and n-butyrate) were measured using an HPLC system (LC-10A, Shimadzu, Kyoto, Japan) equipped with a Shim-pack SCR-102H column (8 mm i.d. × 30 cm long, Shimadzu) and an electroconductivity detector (CDD-6A, Shimadzu).Citation15) Briefly, ~300 mg cecal contents was homogenized in 2 ml of 10 mmol/L NaOH and then centrifuged at 10,000 × g for 15 min. The supernatant obtained was applied to HPLC analysis.
Bacterial enzyme activity
Bacterial enzyme activity in the cecal content was measured by the release rate of 4-nitrophenol from nitrophenylglucosides according to the method of Komiyama et al.Citation16) The following substrates were used: for α-glucosidase, 4-nitrophenyl-α-D-glucopyranoside; for β-glucosidase, p-nitrophenyl-β-D-glucopyranoside; for α-galactosidase, p-nitrophenyl-α-D-galactopyranoside; and for β-galactosidase, o-nitrophenyl-β-D-galactopyranoside. The cecal content (0.15 g) was diluted with 0.5 mL of ice-chilled PBS (pH 7.4) and then was homogenized at 2700 rpm at 4 °C for 90 s using a Multi-Beads shocker (MB601U, Yasui Kikai Co, Osaka, Japan). The homogenate was centrifuged (21,880 × g, 10 min, 4 °C), and the supernatant was immediately filtered using an Ultra free-MC (Merck Millipore, Tokyo, Japan). The reaction mixture contained 0.05 mL of substrate solution (5 mM) and 0.1 mL of a 1:4 (v/v) dilution of the above filtrate in 0.1 mol/L phosphate buffer (pH 6.4). Incubation was carried out at 37 °C for 30 min, and after the addition of 0.8 mL of 0.25 mol/L cold sodium carbonate, 4-nitrophenol was quantified at 400 nm. Enzymatic activity was expressed as micromoles of product formed per minute (unit) per gram of cecal content.
Statistical analysis
Values obtained from the experiments were expressed as mean ± SEM. Data were subjected to Bartlett’s test for the homogeneity of variances, and unequal variances were stabilized by log transformation. For samples with equal variances, one-way analysis of variance (ANOVA) was used, followed by Tukey–Kramer post hoc test for multiple comparisons between individual group means. If sample variances were still unequal after log transformation, we used the Steel–Dwass test. Repeated-measures two-way ANOVA was used to analyze the change in H2 excretion among the NDS groups across time. H2 excretion within an individual group was compared with repeated-measures one-way ANOVA. The Tukey–Kramer test and Steel–Dwass test were performed using SAS JMP software (version 9.0.2; Tokyo, Japan). Significance was defined as a p < 0.05.
Results
The molecular weight and DP of IMD were higher than those of ID. That is, the Mn, Mw, Mw/Mn and DP of IMD were 2330, 4710 g/mol, 2.0 and 29, respectively, while those of ID were 1420, 2900 g/mol, 2.0 and 18, respectively.
Experiment 1
Food intake was significantly lower in the IMD and ID groups than in the C group, but a significant difference in body weight gain was not observed (ANOVA p = 0.1091; Table ). The AUC for 0–14 days in (breath + flatus) H2 excretion (AUC0–14d) and portal and perirenal H2 concentrations were significantly greater in all groups administered glucan, especially the ID group, than in the C group (Fig. , Table ). However, (breath + flatus) H2 excretion in the IMD and ID groups on d 14 was 11% and 9% of that on the day that it peaked in the respective group (Fig. ). The weights of cecal contents and tissue were significantly higher in the three glucan groups than in the C group, and the pH of cecal contents was significantly lower in the three glucan groups (Table ). Only the cecal succinate concentration, but not the cecal concentration of short-chain fatty acids, was significantly higher in the IMD group than in the C group, while n-butyrate and succinate concentrations were significantly higher in the ID and HAS groups than in the C group. Compared with the C group, cecal α-D-glucosidase activity was significantly higher in the ID group and was significantly lower in the HAS group, and β-D-glucosidase activity was significantly weaker in the IMD and HAS groups and was stronger in the ID group. The total number of bacteria was significantly higher in the three glucan groups compared with the C group. The abundance of Firmicutes was significantly greater in the ID and HAS groups than in the C group. In addition, the abundance of Bacteroidetes was significantly higher in the IMD group compared with the C group. Compared with the C group, the abundance of Bacteroides–Prevotella was significantly greater in the three glucan groups, while that of Clostridium cluster XIVa was higher in the glucan groups except for the IMD group. The numbers of Bacteroides–Prevotella and Bifidobacterium were higher in the IMD and HAS groups, but not the ID group, compared with those in the C group. No significant difference in the abundance of Lactobacillus was observed.
Table 1. Food intake, bodyweight gain, breath and flatus H2 excretion, portal and abdominal H2 concentrations in rats fed nondigestible α-glucan for 14 days (Expt. 1).
Fig. 1. Change in (breath + flatus) H2 excretion in rats fed the (C), IMD, ID and HAS diets for 14 days (Expt. 1).
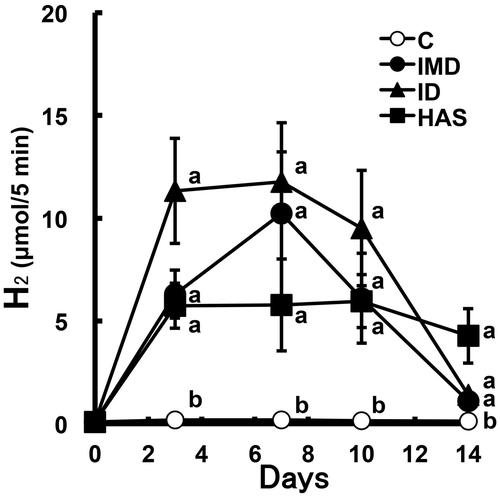
Table 2. Weight of cecal tissue and content, cecal organic acid concentration, and bacterial enzyme activity, counts and ratio in rats fed nondigestible α-glucan for 14 days (Expt. 1).
Experiment 2
Food intake and body weight gain were dose dependently lower in rats receiving dietary IMD supplementation than in the C group (Table ). The AUC0–14d and portal and perirenal H2 concentrations were dose dependently increased by IMD feeding compared with the C group and those in the 16.7% IMD group were 66-, 26-, and 28-fold of the respective value in the C group (Fig. and Table ). IMD feeding dose dependently reduced the weight of perirenal adipose tissue. IMD feeding dose dependently increased the weights of cecal contents and tissue (Table ). The pH of cecal contents was dose dependently reduced by IMD feeding. As in Experiment 1, the cecal acetate concentration did not differ among the five groups. The succinate concentration dose-dependently increased by IMD feeding and that in the 16.7% IMD group was 14-fold of the value in the C group. Cecal α-D-glucosidase activity in all IMD groups was about 3-fold of the value in the C group, while IMD feeding dose dependently decreased β-D-glucosidase activity. IMD feeding dose dependently increased the total number of bacteria. The abundance of Bacteroidetes was dose dependently increased by IMD feeding compared with that in the C group. In addition, the Firmicutes/Bacteroidetes ratio in the 3.3%, 6.7%, and 16.7% IMD groups was 48%, 12%, and 5% of the value in the C group, respectively. The abundance of Bacteroides–Prevotella and Bifidobacterium increased and that of Clostridium cluster XIVa decreased in a dose-dependent manner.
Table 3. Dose response of isomaltodextrin on food intake, bodyweight gain, breath and flatus H2 excretion, portal and abdominal H2 concentrations in rats.
Fig. 2. Dose response of IMD on (breath + flatus) H2 excretion in rats (Expt. 2).
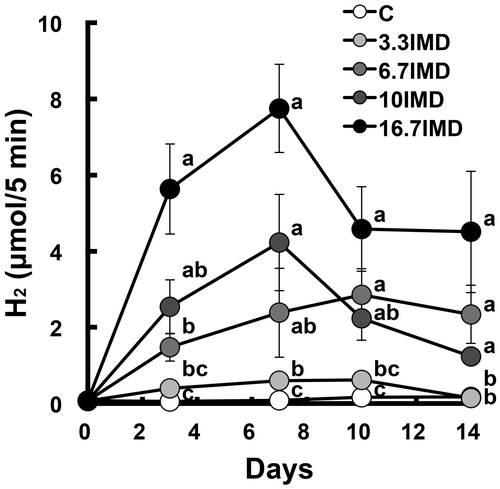
Table 4. Dose response of isomaltodextrin on weight of cecal tissue and content, cecal organic acid concentration, and bacterial enzyme activity, counts and ratio in rats.
Experiment 3
Food intake and body weight gain were significantly lower in the 16.7% IMD group and tended to be lower in the 5.2% FOS group than in the C group (Table ). The AUC0–14d was significantly greater to the same degree in the 16.7% IMD and 5.2% FOS groups than in the C group (Fig. and Table ). Portal and perirenal H2 concentrations were also significantly greater in both groups than in the C group, and the portal H2 concentration in the 5.2% FOS group was 2.7-fold of that in the 16.7% IMD group. Feeding IMD or FOS significantly reduced the weight of the perirenal adipose tissue compared with that in the C group. The weights of cecal contents and tissue were significantly higher in the 16.7% IMD and 5.2% FOS groups than in the C group, and the pH of cecal contents was significantly lower in both groups (Table ). Cecal acetate concentration was significantly higher in the 5.2% FOS group, but not in the 16.7% IMD, compared with that in the C group. The propionate concentration was significantly higher or lower in the 16.7% IMD and 5.2% FOS groups, respectively, compared with that in the C group. The butyrate concentration was significantly higher in both groups, and the butyrate concentration in the 5.2% FOS group was 3.9-fold of that in the 16.7% IMD group. Cecal α-D-glucosidase activity was significantly higher in the 16.7% IMD group, but was significantly lower in the 5.2% FOS group compared with that in the C group. Compared with the C group, the total number of bacteria was significantly higher in both groups. The abundance of Firmicutes was significantly greater in the 5.2% FOS group and was significantly lower in the 16.7% IMD group than in the C group. In addition, the abundance of Bacteroidetes was significantly higher in the IMD group only. The Firmicutes/Bacteroidetes ratio in the 16.7% IMD and 5.2% FOS groups was 5% and 424% of the value in the C group, respectively. Compared with the C group, the abundance of Bacteroides–Prevotella was significantly greater in both groups, and that in the 16.7% IMD and 5.2% FOS groups was 1400 and 230% of the value in the C group, respectively. The abundance of Bifidobacterium in the 16.7% IMD and 5.2% FOS groups was 50,200 and 81,2000% of the value in the C group. The abundance of Clostridium cluster XIVa was significantly lower or higher in the 16.7% IMD and 5.2% FOS groups, respectively, compared with that in the C group.
Table 5. Comparison between isomaltodextrin and fructooligosaccharide on food intake, bodyweight gain, breath and flatus H2 excretion, portal and abdominal H2 concentrations in rats.
Fig. 3. Comparison between IMD and FOS on (breath + flatus) H2 excretion in rats (Expt. 3).
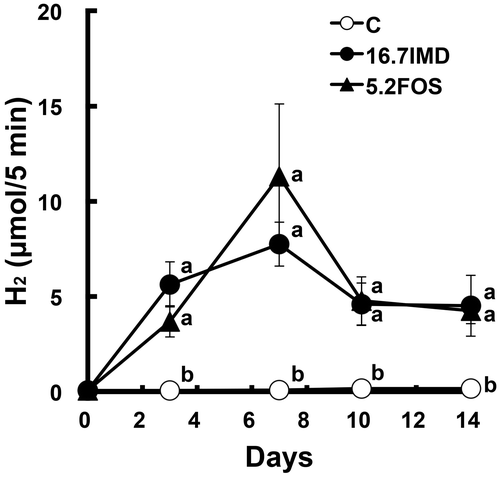
Table 6. Comparison between isomaltodextrin and fructooligosaccharide on weight of cecal tissue and content, cecal organic acid concentration, and bacterial enzyme activity, counts and ratio in rats.
Discussion
The fermentation rate of NDSs which includes the gas production rate and SCFA production rate is likely influenced by the monosaccharide composition, the glycosidic linkages between the monosaccharide residues, and the DP of the particular NDS.Citation11,12) In the case of fructans, the lower the DP, the more rapid its fermentation rate, resulting in increased production of gases.Citation17) NDSs with low DP would be utilized easily by bacteria because solubility increases as the DP decreases. Breath H2 excretion can be used as an indicator of colonic H2 production.Citation18) In the present study, considering the AUC0–14d, although the extent of colonic H2 production in the ID group (DP, 18) was 200% of the value in the IMD group (DP, 29), this difference was not statistically significant. Also, increased H2 excretion by HAS feeding was almost as much as that by IMD feeding, although HAS is an insoluble α-glucan with a high DP unlike IMD. Resistant starches, such as HAS, have been reported to be relatively rapidly fermented in the large intestine.Citation19,20) These findings could support our results. Therefore, HAS, as well as IMD and ID, is also suggested to increase colonic H2 production. Unlike fructan, α-glucans that reach the large intestine would be easily utilized by colonic bacteria and the fermentation rate would be immune to the differences in DP and solubility. The present study demonstrated that IMD, a new indigestible α-glucan that is enzymatically made from maltodextrin, as well as ID and HAS, partially at least reached the large intestine and induced colonic H2 production. However, further investigation is required to verify that this α-glucan could increase colonic H2 production independent of its DP and solubility.
In the present study, high α-glucosidase activity in cecal contents was observed in rats fed NDSs that have α-glycoside linkage, except for HAS, and the increase in the activity reached a plateau by administration of the 3.3% IMD diet. Also, only ID which has a small proportion of β-glycoside linkage (<3% of all glycosidic linkage) as well as α-glycoside linkage, amplified β-glucosidase activity in cecal contents. These results are understandable because bacteria are exposed to a limited carbon source. However, the effect of ID on β-glucosidase activity should be compared with that of β-glucan by further study. The shift in glycosidic enzyme activity in cecal contents would be attributed to dynamic changes in the composition of colonic microbiota as well as altered gene expression of the enzyme in colonic bacteria.
NDSs potentially shift the microbiota composition in the large intestine because the preferred fermentation substrate differs depending on the genus of bacteria. Most (90%) of the phylotypes of gut bacteria are members of two phyla: the Bacteroidetes (Gram-negative) and the Firmicutes (Gram-positive).Citation21) The Firmicutes account for about 70% of the total bacterial population within the human colonic microbiotaCitation21) and the population corresponded to the Firmicutes/Bacteroidetes (F/B) ratio of the C group (3.5) in the present study. The F/B ratio after IMD feeding decreased to 0.5 and Bacteroidetes were predominant, although the F/B ratio after ID and HAS feedings was 1.7 and 2.6, respectively (Table ). Also, the effect of IMD was dose dependent. Unlike the bacterial phylum Firmicutes, Bacteroides spp., which belong to the bacterial phylum Bacteroidetes, can take soluble polysaccharides into the periplasmic space and degrade them.Citation22,23) Bacteria belonging to the genus Bacteroides may preferentially utilize soluble NDSs compared with other bacteria. Holscher et al.Citation24) reported that polydextrose and soluble corn fiber decreased the F/B ratio in feces in humans. Therefore, these reports support the present results in that the abundance of Bacteroidetes increased and the F/B ratio decreased by administration of IMD and ID, which are soluble. A high F/B ratio has been reported to be related to increased adipose tissue and the development of obesity.Citation25) Our results that adipose tissue decreased in the IMD group, which showed a low F/B ratio (0.19 vs. 3.82 in the C group), appear to be significant. However, the weight of adipose tissue decreased in the FOS group even though the F/B ratio (16.2) was higher than that in the C group. It would be simplistic to assume that the shift in the composition of colonic microbiota directly contributes to the change in adipose tissue weight.
Bifidobacteria are thought to confer health benefits to the host.Citation26) The bifidogenic effect of FOS, inulin, galactooligosaccharide, and resistant starch has been well established in various rat and human studies.Citation27,28) Isomaltooligosaccharide was reported not to be bifidogenic in humans in a controlled human study.Citation28) To the best of our knowledge, there have been few studies demonstrating the bifidogenic effect of α-glucan except resistant starch. In this study, IMD feeding increased the number of cecal bifidobacteria in a dose-dependent manner, suggesting that IMD is a bifidogenic α-glucan.
In our previous study, oxidative stress induced by hepatic ischemia-reperfusion was suppressed when H2 excretion and portal H2 concentration in rats fed NDSs were more than 5 μmol/5 min and in the range of 3–9 μmol/L, respectively.Citation6,29) FOS feeding generates considerable amounts of colonic H2 in rats and humans compared with feeding of other NDSs.Citation7,10) Therefore, FOS would be a useful NDS that could alleviate oxidative stress and oxidative damage. In the present study, the H2 excretion and portal H2 concentration in rats fed ID or IMD on day 3–10 crossed these benchmarks. In the present study, IMD dose dependently increased colonic H2 production and its ability to increase the H2 production was not inferior to that of FOS. However, compared with rats fed FOS, adipose H2 concentration in rats fed IMD was lower. In our previous study, high adipose H2 concentration (9 μmol/kg) in dietary-induced obese rats fed FOS decreased adipose mRNA abundance of inflammatory cytokine. Therefore, unlike FOS, IMD may not increase adipose H2 concentration even though (breath + flatus) H2 excretion and portal H2 concentration are high. H2 production rate in the large intestine could change the movement of colonic H2. Further study is required to determine why adipose H2 concentration is different in rats with high colonic H2 generation.
Taken together, IMD, which is a highly branched α-glucan and partially escapes digestion in the small intestine, significantly increased colonic H2 production as well as ID and HAS. IMD feeding dose dependently increased colonic H2 production. Furthermore, the ability of IMD to increase colonic H2 production was almost equal to that of FOS. Therefore, IMD would be a new potential food source for colonic H2 production. Moreover, IMD shifts the composition of colonic microbiota to a low F/B ratio and a high level of bifidobacteria.
Author contributions
NN designed the study; NN, HT, and TY conducted the study; NN analyzed the dataset; NN wrote the manuscript; and NN had primary responsibility for the final content. All authors were involved in designing the study, reviewing and interpreting the results, and drafting the manuscript. All authors read and approved the final manuscript.
Disclosure statement
The study was proposed, designed and all analysis performed solely by the authors.
Funding
This work was supported by Hayashibara Co., Ltd. (Okayama, Japan).
Supplemental material
The supplemental material for this paper is available at http://dx.doi.org/10.1080/09168451.2015.1104237.
Supplemental_Fig1-final.jpg
Download JPEG Image (1.8 MB)References
- Macfarlane S, Macfarlane GT, Cummings JH. Review article: prebiotics in the gastrointestinal tract. Aliment Pharmacol Ther. 2006;24:701–714.10.1111/apt.2006.24.issue-5
- Topping DL, Clifton PM. Short-chain fatty acids and human colonic function: roles of resistant starch and nonstarch polysaccharides. Physiol. Rev. 2001;81:1031–1064.
- Behall KM, Scholfield DJ, van der Sluijs AM, et al. Breath hydrogen and methane expiration in men and women after oat extract consumption. J. Nutr. 1998;128:79–84.
- Kajs TM, Fitzgerald JA, Buckner RY, et al. Influence of a methanogenic flora on the breath H2 and symptom response to ingestion of sorbitol or oat fiber. Am. J. Gastroenterol. 1997;92:89–94.
- Wagner JR, Becker R, Gumbmann MR, et al. Hydrogen production in the rat following ingestion of raffinose, stachyose and oligosaccharide-free bean residue. J. Nutr. 1976;106:466–470.
- Nishimura N, Tanabe H, Sasaki Y, et al. Pectin and high-amylose maize starch increase caecal hydrogen production and relieve hepatic ischaemia-reperfusion injury in rats. Br. J. Nutr. 2012;107:485–492.10.1017/S0007114511003229
- Nishimura N, Tanabe H, Adachi M, et al. Colonic hydrogen generated from fructan diffuses into the abdominal cavity and reduces adipose mRNA abundance of cytokines in rats. J. Nutr. 2013;143:1943–1949.10.3945/jn.113.183004
- Tsusaki K, Watanabe H, Nishimoto T, et al. Structure of a novel highly branched alpha-glucan enzymatically produced from maltodextrin. Carbohydr. Res. 2009;344:2151–2156.10.1016/j.carres.2009.08.016
- Okuma K. What is resistant maltodextrin? Process, structure, and properties. In: Gordon DT, Goda T, editors. Dietary fiber: an international perspective for harmonization of health benefits and energy values. MN: AACC International; p. 151–160.
- Oku T, Nakamura S. Evaluation of the relative available energy of several dietary fiber preparations using breath hydrogen evolution in healthy humans. J. Nutr. Sci. Vitaminol. 2014;60:246–254.10.3177/jnsv.60.246
- Cummings JH, Macfarlane GT, Englyst HN. Prebiotic digestion and fermentation. Am. J. Clin. Nutr. 2001;73:415S–420S.
- de Preter V, Vanhoutte T, Huys G, et al. Baseline microbiota activity and initial bifidobacteria counts influence responses to prebiotic dosing in healthy subjects. Aliment Pharmacol. Ther. 2008;27:504–513.
- Reeves PG, Nielsen FH, Fahey GC Jr. AIN-93 purified diets for laboratory rodents: final report of the American Institute of Nutrition ad hoc writing committee on the reformulation of the AIN-76A rodent diet. J. Nutr. 1993;123:1939–1951.
- Guo X, Xia X, Tang R, et al. Development of a real-time PCR method for Firmicutes and Bacteroidetes in faeces and its application to quantify intestinal population of obese and lean pigs. Lett. Appl. Microbiol. 2008;47:367–373.10.1111/lam.2008.47.issue-5
- Hoshi S, Sakata T, Mikuni K, et al. Galactosylsucrose and xylosylfructoside alter digestive tract size and concentrations of cecal organic acids in rats fed diets containing cholesterol and cholic acid. J. Nutr. 1994;124:52–60.
- Komiyama Y, Mitsuyama K, Masuda J, et al. Prebiotic treatment in experimental colitis reduces the risk of colitic cancer. J. Gastroenterol. Hepatol. 2011;26:1298–1308.10.1111/jgh.2011.26.issue-8
- Hernot DC, Boileau TW, Bauer LL, et al. In vitro fermentation profiles, gas production rates, and microbiota modulation as affected by certain fructans, galactooligosaccharides, and polydextrose. J. Agric. Food Chem. 2009;57:1354–1361.10.1021/jf802484j
- Levitt MD. Production and excretion of hydrogen gas in man. N. Engl. J. Med. 1969;281:122–127.10.1056/NEJM196907172810303
- Tomlin J, Read NW. The effect of resistant starch on colon function in humans. Br. J. Nutr. 1990;64:589–595.10.1079/BJN19900058
- Topping DL, Gooden JM, Brown IL, et al. A high amylose (amylomaize) starch raises proximal large bowel starch and increases colon length in pigs. J. Nutr. 1997;127:615–622.
- Eckburg PB, Bik EM, Bernstein CN, et al. Diversity of the human intestinal microbial flora. Science. 2005;308:1635–1638.10.1126/science.1110591
- Martens EC, Koropatkin NM, Smith TJ, et al. Complex glycan catabolism by the human gut microbiota: the bacteroidetes sus-like paradigm. J. Biol. Chem. 2009;284:24673–24677.10.1074/jbc.R109.022848
- Flint HJ, Duncan SH, Scott KP, et al. Links between diet, gut microbiota composition and gut metabolism. Proc. Nutr. Soc. 2015;74:13–22.10.1017/S0029665114001463
- Holscher HD, Caporaso JG, Hooda S, et al. Fiber supplementation influences phylogenetic structure and functional capacity of the human intestinal microbiome: follow-up of a randomized controlled trial. Am. J. Clin. Nutr. 2015;101:55–64.10.3945/ajcn.114.092064
- Turnbaugh PJ, Ley RE, Mahowald MA, et al. An obesity-associated gut microbiome with increased capacity for energy harvest. Nature. 2006;444:1027–1031.10.1038/nature05414
- Fontana L, Bermudez-Brito M, Plaza-Diaz J, et al. Sources, isolation, characterisation and evaluation of probiotics. Br. J. Nutr. 2013;109:S35–S50.10.1017/S0007114512004011
- Meyer D, Stasse-Wolthuis M. The bifidogenic effect of inulin and oligofructose and its consequences for gut health. Eur. J. Clin. Nutr. 2009;63:1277–1289.10.1038/ejcn.2009.64
- Bouhnik Y, Raskine L, Simoneau G, et al. The capacity of nondigestible carbohydrates to stimulate fecal bifidobacteria in healthy humans: a double-blind, randomized, placebo-controlled, parallel-group, dose-response relation study. Am. J. Clin. Nutr. 2004;80:1658–1664.
- Tanabe H, Sasaki Y, Yamamoto T, et al. Suppressive effect of high hydrogen generating high amylose cornstarch on subacute hepatic ischemia-reperfusion injury in rats. Biosci. Microbiota Food Health. 2012;31:103–108.10.12938/bmfh.31.103