Abstract
dl-Penicillamine, a copper-specific metal chelator, remarkably suppressed the growth of Bacillus subtilis 168 when added to a synthetic medium under Cu2+ limitation. DNA microarray and screening of 2,602 knockout mutants showed that the zosA gene was de-repressed in the presence of 0.1% dl-penicillamine, and that the zosA mutant was sensitive to dl-penicillamine medium. The zosA mutant delayed the growth under Cu-limitation even without the chelator, and the sensitivity to dl-penicillamine was reversed by induction using 0.3 mM IPTG and the Pspac promoter inserted directly upstream of the zosA gene. Furthermore, the zosA mutant showed elevated tolerance of excessive Cu2+ but not of excessive Zn2+ added to LB and synthetic media. Homology modeling of the ZosA protein suggested that the protein can fold itself into essential domains for constituting a metal transporting ATPase. Our study suggests that zosA is a candidate gene involved in copper uptake.
Graphical abstract
Modeling of ZosA protein by molecular dynamics simulation.
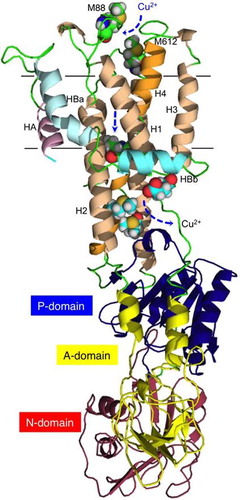
Organisms essentially require copper as a cofactor for many proteins and enzymes that play key biological roles such as respiration and protection from oxidative stress. As a redox-active transition metal, copper may also have toxic effects by generating reactive oxygen species or, more likely, by causing extensive thiol depletion in aerobically growing cells.Citation1–3) Regulation of intracellular copper activity is thus crucial, and a mechanism must exist for the safe management of copper activity. Microbial copper homeostasis has been studied most thoroughly in the copYZAB operon of Enterococcus hirae.Citation4) In the presence of >50 μM copper (I), the repressor CopY allows expression of copA and copB in E. hirae cells. CopA and copB encode transmembrane ATPases that are involved in copper import and export, respectively.Citation5) CopZ is an intracellular metal chaperone that is involved in copper delivery.Citation2)
Pathogenic streptococci, such as Streptococcus pyogenes,Citation1,6) S. agalactiae,Citation7) and the cariogenic dental pathogen, S. mutans,Citation8) commonly have a copper-regulating operon, copYAZ, encoding a repressor (CopY), a Cu-exporting ATPase (CopA), and a copper chaperone (copZ). A gene encoding a Cu-uptake ATPase has not been identified. The dairy bacterium S. thermophilus, which is phylogenetically close to the pathogenic streptococci, also has the copYAZ-type operon in its genome.Citation2) The copYAZ operon was found in clinically isolated, vancomycin-resistant Enterococcus faecalis, and the copper-importing gene was not identified in its genome.Citation9) Interestingly, a copper-uptake ATPase has been identified among the virulence-related genes that are up-regulated during the pathogenesis of Staphylococcus aureus, suggesting that copper might be a limiting nutritional requirement in the event of pathogenesis.Citation10) Bacillus subtilis 168 and the closely related B. licheniformisCitation11) have another type of copper operon, csoR-copZA, in which the copper chaperone gene, copZ, and the Cu-exporting ATPase gene, copA, are regulated by the copper-sensing transcriptional repressor encoded by csoR.Citation12) A gene encoding a Cu-uptake ATPase is not included in these copper operons, nor has it been identified anywhere in the genome.
A previous study reported the involvement of the ycnKJI operon in copper homeostasis in B. subtilis 168; the ycnKJI operon includes the copper-binding repressor YcnK, which regulates the downstream ycnJ gene.Citation13) The ycnJ gene encodes a transmembrane metal-permease whose amino acid sequence shares high homology with a Cu-efflux protein encoded by copCD in P. fluorescens SBW25, a plant pathogen characterized as highly resistant to the Bordeaux mixture, a fungicidal CuSO4 solution.Citation14) The physiological role of the ycnKJI operon in B. subtilis was elucidated by a more recent report, which elegantly revealed the involvement of ycnJ gene in copper import when a reversible metal chelator, bathocuproine sulfonate (BCS), was added to the medium.Citation15) The effect of reversible copper chelator was substantiated by the previous observation that B. subtilis grown in the presence of BCS accumulated twice as much copper (3.58 ppb) as cells grown without the BCS (1.84 ppb). This controversy may be understood by the peculiar behavior of BCS,Citation16) which deplete copper from media, whereas BCS-bound copper can also be taken up in the cells under the concomitant induction of ycnJ gene.
Penicillamine (3,3-dimethyl-cysteine) is a heavy metal chelator that can bind copper, lead, and nickel. The metal specificity of penicillamine is high for copper, forming an adduct with Cu irreversibly,Citation17) but other metals are bound reversibly with varied affinity (relative affinities: Hg2+ > Pb2+ > Ni2+ > Zn2+ > Co2+).Citation18) The present study aimed to exploit dl-penicillamine for imposing copper-limiting conditions on growing cells of B. subtilis 168. A P-type transporter, zosA, formerly referred to as ykvW, was identified both in the microarray study and screening of the gene knockout library. The zosA gene was proposed to be a zinc-uptake ATPase under oxidative stress.Citation19) We report that the zosA mutant showed a remarkable growth delay when copper was omitted from the synthetic medium and conversely, an elevated tolerance to CuSO4. Homology modeling and molecular dynamics simulation of the ZosA protein suggested that the gene product should have a ternary structure with domains and scaffolds necessary for function as a metal-uptake ATPase.
Materials and methods
Bacterial strains and culture conditions
The gene knockout library was constructed by inserting the Pspac promoter upstream of each target gene.Citation20) The synthetic medium containing 0.5% (w/v) D-glucose, 0.1% ammonium sulfate, 0.05% yeast extract, 0.1% K2HPO4, 0.1% KH2PO4, 0.1% NaCl, and 0.05% MgSO4·7H2O, pH 7.0, was prepared in 18 MΩ-water and supplemented with metals such as 5.5 μg/mL CaCl2, 1.7 μg/mL ZnCl2, 0.43 μg/mL CuCl2·2H2O, 0.6 μg/mL CoCl2·6H2O, and 0.6 μg/mL Na2MoO4·2H2O. FeCl3 and MnSO4 were also added to the medium at final concentrations of 4.0 or 0.2 μg/mL, respectively. Metal-limiting media were prepared by omitting one of the metal ingredients from the synthetic medium. An aqueous solution of dl-penicillamine was freshly prepared and filter sterilized when added to the medium. l-Glutamate was substituted for D-glucose as a carbon source when the effect of (isopropyl-β-D-thiogalactopyranoside) IPTG induction was tested.
Effects of metals on growth
The effect of dl-penicillamine to alleviate metal stress was assayed by counting viable cells in growing cultures of B. subtilis 168. Synthetic media containing 0.1 mM CuSO4, 0.5 mM ZnSO4, or 5 mM MnSO4 were prepared with or without 0.1% (w/v) (6.7 mM) dl-penicillamine. B. subtilis 168 was inoculated and grown at 37 °C under aeration. Cells collected at 9, 12, 15, and 17 h were prepared in a series of 102- to 107-fold dilutions and 5-μL aliquots of these cell suspensions were spotted in triplicate on the synthetic agar plate medium. The number of viable cells was counted after incubation at 30 °C for 12 h.
Microarray analysis
B. subtilis 168 was grown overnight on the synthetic medium, washed twice with saline buffer, and inoculated in 100 mL of the synthetic media at an optical density (OD600) of 0.02. The cultures were grown with 0.1% (6.7 mM) dl-penicillamine at 37 °C, and total RNA was isolated from the cells in the early logarithmic phase at 3 h. Fluorescence-labeled cDNA probes were prepared as described previously.Citation21) Hybridization and microarray analysis were also performed as described previously.Citation22) The expression ratios were calculated in relation to the fluorescence intensities of Cy3 and Cy5 for each spot.Citation23) The results were deposited in the KEGG Expression database (http://www.genome.jp/kegg/expression/) as ex0001763 and ex0001764.
Growth assay under metal limitation
A library of gene knockout strains of B. subtilis 168 were screened for a mutant hypersensitive to copper starvation induced by 0.1% (6.7 mM) dl-penicillamine. Each frozen stock mutant was grown overnight at 30 °C in 5 mL of the normal synthetic medium containing 0.5 μg/mL erythromycin and 1.5 μg/mL lincomycin to maintain the inserted gene fragment. The cells were washed and suspended in saline at approximately 109 cells/mL. A series of 102- to 107-fold dilutions were prepared with sterile saline and 5 μL of the cell suspensions was spotted on copper-free synthetic media with or without 0.1% dl-penicillamine. Cells of the wild-type strain were also spotted along with the mutant cells for reference. When colony formation on the 0.1% dl-penicillamine medium was less than that observed on the control medium, the mutant strain was considered to be sensitive to 0.1% dl-penicillamine. The spotting assay was also used to measure the sensitivity to CuSO4 or ZnSO4 using the wild-type strain and the zosA mutant. Diluted cell suspensions were spotted in triplicate on the synthetic agar plate medium containing either 0.1 mM CuSO4 or 0.5 mM ZnSO4, and the plates were incubated at 30 °C for 12 h to count the number of colonies.
Double-knockout mutant
The csoR-copZA operon was deleted from the genomes of the wild-type strain and the zosA mutant. The deletion mutants were constructed as described previouslyCitation24) using four primers cop-F1 (5′-TGTATGCATGCCATA GTAT-3′), cop-R1 (5′-GTTATCCGCTCACAATTCAATCCGCTTAAGCCGGTTTG -3′), cop-F2 (5′-CGTCGTGACTGGGAAAAC CAGCTCCGTTTCCGTTGTTC-3′), and cop-R2 (5′-TTCTG ATCAG CATAT TGACC-3′). The chloramphenicol acetyl transferase gene, cat, was amplified by PCR from plasmid pCBB31, using the primers pUC-F (5′-GTTTT CCCAG TCACG ACG-3′) and pUC-R (5′-GAATT GTGAG CGGAT AAC-3′). The 18 bases at the 5′ ends of cop-R1 and cop-F2, designated in italics, are complementary to the sequence of pUC-R and pUC-F, respectively. The mixture of the three PCR products was used as the template for the second round of PCR using the primers cop-F1 and cop-R2, and the resulting PCR fragment, amplified via overlap extension, was used for transformation of the wild-type and zosA mutant strains. The deletion was confirmed by DNA sequencing.
Homology modeling and molecular dynamics
The full-length sequence of ZosA was aligned with 11 type IB P-type transporting ATPases with confirmed copper specificity (O32220, A9CJP7, Q9VYT4, P32113, Q1D6W1, Q57S66, B9DFX7, Q7A3E6, O29777, Q04656, P35670, Q5ZWR1). The ternary structure model for the ZosA protein was constructed on the Molecular Operating Environment (Chemical Computing Group Inc., Montreal, Canada) implemented with the 3D protonation matrixCitation25) in the implicit solvent model.Citation26) The crystal structure of L. pneumophila Lp-CopACitation27) was used as the template for modeling. Molecular dynamics simulations using the AMBER12 program were used to further optimize the geometry of the homology model in the isotherm–isobar thermodynamic ensemble at 300 K. The generalized Born model augmented with the hydrophobic solvent accessible surface area model was used as the solvent model. Simulations were carried out with the sander MPI module using the SHAKE algorithm on bonds involving hydrogen atoms. Accordingly, 500,000 steps of minimization in 11 ns of MD simulation were performed to reach the final geometry of the ZosA protein. The stereochemistry of the model structure was checked by Ramachandran plot. The accuracy of the computation model was assessed by the 3D–1D scoring using the Verify3D Structure Evaluation Server.Citation28) Energy on each residue was checked by ProSA using the web service ProSA-web.Citation29)
Results
Effects of dl-penicillamine on growth of B. subtilis 168
dl-Penicillamine was added to synthetic media supplemented with metals, and the growth rate of B. subtilis 168 was examined. The generation time in the early logarithmic phase was increased from 35 min in 0% dl-penicillamine to 46 min in 0.3% (20.1 mM) and 71 min in 0.5% (33.5 mM) dl-penicillamine (Table ). Then, different media were prepared by omitting one of the metals from each, and growth in the early log phase was examined as a function of generation time vs. the concentration of dl-penicillamine. The generation time was remarkably prolonged when the synthetic medium was prepared without CuCl2. Omitting other metals such as zinc did not enhance the growth retardation by dl-penicillamine.
Table 1. Effects of dl-penicillamine on generation time in log phase B. subtilis 168.
The binding specificity of dl-penicillamine was also assessed by its ability to alleviate the toxicity of excess metals added to the synthetic media. Cells of B. subtilis 168 grown in 5 mL of synthetic medium at 30 °C overnight were washed twice with saline, and approximately 103 cells/mL were inoculated in 5 mL of the synthetic media containing 5 mM HEPES at pH 7.0 (Fig. ). The growth of B. subtilis 168 was sensitive to 0.1 mM CuSO4, but the cells propagated as rapidly as they did on the normal medium when 0.1% (6.7 mM) dl-penicillamine was added to the culture. Interestingly, B. subtilis 168 was sensitive to 0.5 mM ZnSO4 but the culture reached the late log phase at 17 h, whereas addition of 0.1% dl-penicillamine allowed the bacteria to grow as rapidly as in the normal medium in the early log phase. It has been reported that penicillamine can also bind zinc whereas the complex is not as stable as it is with copper.Citation30) Manganese delayed the growth of B. subtilis 168 at a concentration of 5 mM, and the addition of dl-penicillamine had no effect.
Fig. 1. Effects of metals and 0.1% dl-penicillamine on growth of B. subtilis 168.
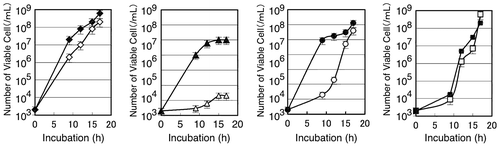
Microarray analysis
DNA microarray analysis was performed using pairs of cultures grown with or without dl-penicillamine. In the presence of 0.1% (6.7 mM) dl-penicillamine, the growth was slightly slower than that without the chelator. The two cultures were grown for three hours, and total RNA was extracted for reverse transcription. The intensities of gene expression obtained from the culture with dl-penicillamine were divided by those obtained from the one without the metal chelator. The ratios summarized in Table were obtained in duplicate experiments in which we adopted the criterion of 10-fold up-regulation to identify the candidate genes that responded significantly to 0.1% dl-penicillamine.
Table 2. B. subtilis 168 genes responding to 0.1% dl-penicillamineTable Footnotea.
B. subtilis 168 expressed several metal transporter genes more than 10-fold in the presence of 0.1% dl-penicillamine; some members of the PerR and Zur regulons showed remarkable de-repression in response to 0.1% dl-penicillamine. The most dramatic increase was observed for the yciC gene, which exhibited >170-fold de-repression. The yciC promoter is a target of the Zur repressor. It has been reported that another Zur target, the yciAB operon,Citation19) can be transcribed independently but co-directionally from yciC, and transcription of yciAB was also enhanced by 12- to 34-fold in our microarray experiments. Three other strongly up-regulated genes comprise the ycdHI-yceA operon, which has also been reported to be under Zur control. YciABC encodes a putative zinc(II)-uptake system,Citation19) and yceA-ycdHI encodes a putative ABC transporter for Zn(II).Citation31)
The transcription of mntABC genes, encoding bacterial ABC importers, was also increased by 15- to 20-fold in the dl-penicillamine medium. These genes, under the regulation of the MntR repressor, have been characterized to be essential for the import of manganese.Citation32)
The zosA gene encodes a P-type metal transporter, which has been proposed to be a zinc transporter.Citation19) The zosA gene is under the regulation by PerR repressor, which senses the intracellular Fe/Mn ratio.Citation33) In our experiment, expression of other members of the PerR regulon, including mrgA (Dps-like DNA-binding protein), katA (catalase), and ahpCF (alkylhydroperoxide reductase), was also enhanced 7- to 15-fold.
Screening of the mutant library
B. subtilis 168 has 4106 ORFs, including over 2600 genes whose functions are not fully understood. We screened for mutant strains that are highly sensitive to 0.1% (6.7 mM) dl-penicillamine in the Cu-omitted synthetic medium. In the first screening, 80 strains showed growth defects on the synthetic plate medium containing 0.1% dl-penicillamine. The 80 candidates were then screened by spotting a suspension of 102 cells in 5 μL of saline on the penicillamine medium; 15 strains reproduced the growth defects. The 15 candidate strains and the wild-type strain for comparison were spotted in a series of diluted cell suspensions and grown on synthetic media with or without 0.1% dl-penicillamine. The number of cells required for colony formation indicated the growth inhibition in comparison with the wild-type strain. Three of the mutant strains, zosA, mntD, and ycdD, remained as candidates that were highly sensitive to dl-penicillamine. YcdD is homologous to L-alanoyl-D-glutamate peptidase. The mntD gene (295 bp) is located within the mntABCD operon, which reportedly encodes components of the ABC transporter for manganese.Citation32) Although mntABC genes were de-repressed in our microarray study, mutant strains with disrupted mntA, B, or C genes did not show growth defects on the penicillamine medium. Genes belonging to the Zur regulon (Table ) were not essential for B. subtilis to survive on the 0.1% dl-penicillamine medium. Only the zosA gene, highly homologous to the E. hirae copA, was identified in our DNA microarray investigation and in the screening of the gene knockout library.
Characterization of the zosA mutant
In the zinc-limited medium, the zosA mutant propagated as rapidly as in the synthetic medium when grown at 37 °C, and the mutant was sensitive to Cu-limitation, showing growth retardation in the copper-omitted medium even without dl-penicillamine. The growth delay was not observed for wild-type strain under the same growth conditions (Fig. (A)). The zosA mutant also showed normal growth in synthetic media from which other metals, such as Mn, Mo, Co, and Ca, were omitted (data not shown). Since the zosA mutant was constructed by inserting the Pspac promoter at the upstream of the target gene,Citation20) the function may be recovered to some extent by an appropriate concentration of IPTG. The effect of IPTG was assayed in the Cu2+-omitted synthetic medium in the presence or absence of 0.1% dl-penicillamine when grown at 30 °C. In the presence of 0.1% dl-penicillamine, the growth delay of the zosA mutant was observed at 10 h. When 0.3 mM IPTG was added, the growth of the zosA mutant was restored to a level comparable to that of the wild-type (Fig. (B)). These growth studies demonstrated that the loss of function phenotype is a rather subtle growth delay and that this delay may be recovered at the end of overnight culture.
Fig. 2. Characterization of the zosA mutant.
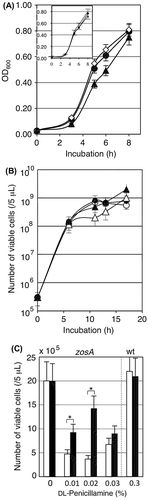
Complementation of the zosA gene by IPTG-induction was also assayed in the final yield of viable cells when dl-penicillamine was added to the copper-omitted synthetic media (Fig. (C)). The growth after overnight was 20 × 105 /5 μL with or without 0.3 mM IPTG. The growth of zosA mutant was suppressed <10 × 105/5 μL when dl-penicillamine was added at concentrations from 0.01 to 0.03%. Induction of zosA by 0.3 mM IPTG recovered the growth when dl-penicillamine was added at 0.01 and 0.02%, but the recovery effect was not obvious when dl-penicillamine was added at 0.03%, suggesting that strong induction of the Pspac promoter by IPTG was not optimal for expressing this membrane protein. The growth of wild-type strain was high in the presence of 0.3% dl-penicillamine, and IPTG had no adverse effect on the growth of B. subtilis 168.
Sensitivity of the zosA mutant to copper and zinc
Typically, if a transporter works in the influx of a certain ion, mutation of the gene will increase tolerance to the metal ion(s) in the culture medium.Citation19) Accordingly, the sensitivity to copper and zinc was tested at higher concentrations in LB medium. Naturally, but interestingly enough, copper and zinc significantly differed in their toxic effects on B. subtilis when added to the HEPES-buffered LB medium. The growth of the wild-type strain was reduced by over 50% when CuSO4 was added at 2 mM, while the zosA mutant showed much higher tolerance to CuSO4 under the same conditions (Fig. ). In contrast, the zosA mutant and wild-type strains showed similar sensitivities to ZnSO4. The zosA mutant was slightly less sensitive to 1.4 mM ZnSO4, but the wild-type and mutant strains were completely inhibited by 1.6 mM ZnSO4 in LB medium.
Fig. 3. The wild-type strain (open symbols) and zosA mutant (closed symbols) were grown overnight on LB media containing either CuSO4 (triangles) or ZnSO4 (circles) at the concentrations designated on the x-axis.
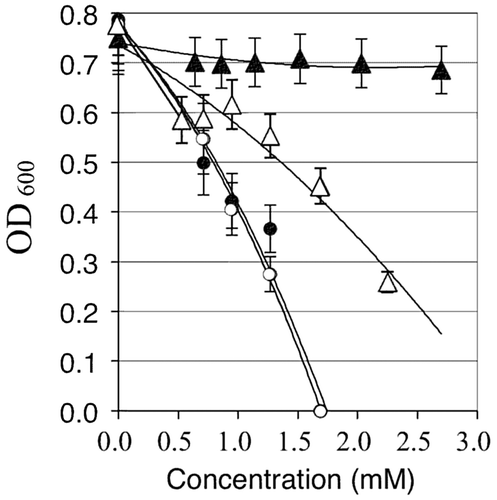
The metal sensitivity was also assayed by a colony spot assay with the synthetic media in which the effect of copper was more intense than that of zinc. After repeated assays using a series of three-quarter dilutions of ZnSO4 or CuSO4, the IC50 for CuSO4 and ZnSO4 were determined to be 0.1 and 0.5 mM, respectively, in the synthetic medium. The IC50 represents the conditions under which bacterial cells can respond to varied concentrations of metals with the highest sensitivity. Therefore, the metal tolerances of the wild-type and zosA mutant were assayed by their ability to form colonies on media containing either 0.1 mM CuSO4 or 0.5 mM ZnSO4. When cell suspensions were spotted on the medium containing 0.1 mM CuSO4, the zosA mutant formed colonies up to the 106-fold dilution but not over the 107-fold dilution. In contrast, the wild-type strain showed the dilution limit at the 104-fold dilution (Fig. (A)). The results again clearly demonstrated that the bacterial cells acquired significantly higher tolerance to CuSO4 by zosA mutation. Assays on agar plates containing 0.5 mM ZnSO4 (Fig. (B)) and without excess metal (Fig. (C)) were also tested with identical cell suspensions, and the results revealed that the two strains showed similar sensitivities to ZnCl2.
Effects of deleting the csoR-copZA operon on Cu sensitivity
It was unclear whether the Cu-regulating proteins encoded in the csoR-copZA operon interact physiologically with the zosA gene product. We compared the Cu-sensitivity of four strains, WT, zosA, ΔcsoR-copZA, and zosA/ΔcsoR-copZA, by spotting the cell suspensions in 10-fold dilutions on media with different concentrations of CuSO4. Remarkably, the ΔcsoR-copZA mutant strain was highly sensitive to copper even at concentrations of CuSO4 as low as <40 μM (Fig. ). As observed in the zosA mutation that elevated the CuSO4 tolerance to >100 μM, repression of the zosA gene had a similar but lesser effect on the ΔcsoR-copZA mutant. The addition of the zosA mutation in the ΔcsoR-copZA background slightly alleviated the copper sensitivity.
Fig. 5. Effect of the csoR-copZA deletion on Cu-sensitivity of B. subtilis 168.
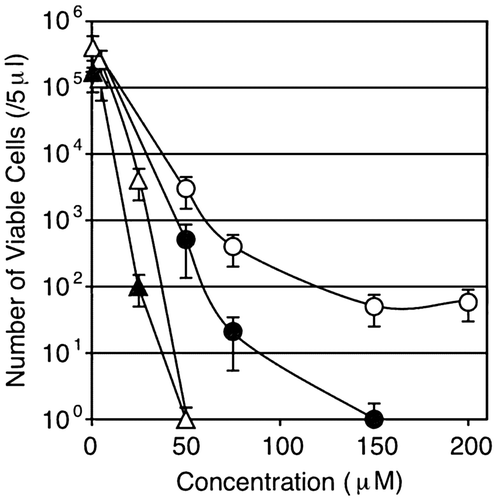
Homology modeling and molecular dynamics simulation
The ZosA protein has a significant sequence identity to copper-transporting ATPases, including the copper exporting CopA from Legionella pneumophila (Lp-CopA), of which X-ray crystallographic structures for the transmembrane domains have been solved at 3.2 Å.Citation27) The N-terminal heavy-metal binding domain (HMBD) of Lp-CopA was not modeled because of poor electron density. The amino acid sequence of ZosA lacks domains corresponding to the HMBD in Lp-CopA, and, therefore, homology modeling of ZosA was performed using the crystal structure of Lp-CopA (3rfu.pdb) in the region from Val74 to the C-terminal Leu736. The geometry of the homology model was further optimized by molecular dynamics simulation.
The quality of the resulting theoretical model was first evaluated by Ramachandran plot, which revealed 67.1% of residues in most favored regions, 27.17% in additional allowed regions, 4.26% in generously allowed regions, and only 1.47% (Glu136, Ala457, Lys569) were off the borderline of generously allowed regions. Then, the ProSA web service was used to evaluate the Z-score values for model structures collected at every nanosecond interval, all of which fell in a narrow range between −7.0 and −6.0, which was within the values for experimentally determined protein chains in the current PDB. Finally, the Verify 3D program was used to assess the compatibility of the theoretical ZosA model at the ultimate conformation after the 11 ns MD simulation. The 3D–1D scoresCitation28) were between −0.30 and 0.72 for the amino acid residues in the predicted ZosA structure, similar to the scores for the template structure, 3rfu.pdb, that were between −0.14 and 0.72.
The cytosolic part of ZosA was predicted to have three domains that are characteristic of P-type ATPases (Fig. (A)),Citation34) comprising the A-domain (actuator), P-domain (phosphorylation), and the N-domain (nucleotide-binding). The transmembrane domain consists of eight helical segments: the six core helices that constitute the principal organization commonly found in other P-type ATPases,Citation35) and two extra helices HA and HBa-HBb. The N-terminal HA interacts with H2 (helix 2) and H6. The long and curved helix HB is divided into two transmembrane helices, HBa and HBb, which are tightly associated with H2, H1, and H3.
Fig. 6 Modeling of ZosA.
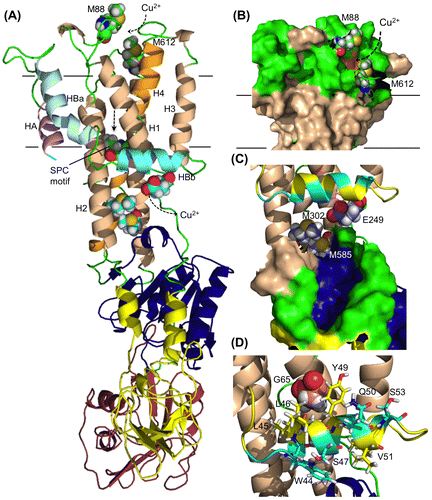
Our theoretical model suggested the location of Met88 and Met612 residues at the copper entrance on top of the bundle of helices (Fig. (B)). The Cu-exporting Lp-CopA does not have corresponding residues on the extracellular site. Behind the wall of the highly conserved SCP motif are Met residues M302 and M585 along with E249, which would make the final trap for copper that departs from the wide-open cavity (Fig. (C)).Citation27) These candidate amino acid residues provide a target for site-directed mutation and are useful for further study to elucidate the cellular function of the zosA gene product. HBb and H1 helices in the Lp-CopA model are proposed to provide a platform from which Cu2+ is transferred to a Cu-chaperone protein. LP-CopA has positively charged residues on the cytosolic face of HBb, while the HBb of ZosA has a hydrophilic array of residues W44, S47, and S53. Residues L45, L46, and Y49 attach themselves to the hydrophobic face of the H1 and H3 helices and this putative hydrophobic interaction contributes to the stability of the intramembranous structure (Fig. (D)).
Discussion
B. subtilis 168 is a model bacterium that has a wealth of known genome research tools, such as the genome sequence, DNA microarrays, and a gene knockout library. In this study, we used dl-penicillamine, a highly specific and irreversible Cu2+-chelator, in order to produce copper deficient conditions in culture.Citation18) Our microarray study identified several genes belonging to the Zur-, MntR-, and PerR-regulons that are de-repressed over 10-fold in response to 0.1% dl-penicillamine. The zosA gene has been identified as a member of the PerR regulon, which is specifically induced by the PerR metalloregulatory protein that binds Zn and either Fe or Mn as a cofactor for repression,Citation33) suggesting that the de-repression of PerR regulon was caused by limitation of zinc, iron or manganese besides copper.
The zosA gene has not been recognized as a Cu-uptake transporter due to the following reasons. First, the deduced amino acid sequence lacks an N-terminal metal-binding domain with the consensus motif CxxC, which is conserved in E. hirae CopA and other copper transporters such as MNK and WND in human.Citation36,37) It is unclear whether the CxxC motif is required for the metal-binding function or is dispensable. The E. hirae CopB, like some other CPx-type ATPases, does not have the N-terminal CxxC motif, but instead features a histidine-rich N-terminus that most likely constitutes a metal-binding domain. However, ZosA does not have a sequence corresponding to the histidine-rich region. Second, heavy metal ATPases have an intramembranous motif that begins with CPC or CPH (also sometimes SPC). Tottey et al. suggested that copper-transporting ATPases tend to feature the membrane channel motif CPCALGLA, while this motif is CPCALVIS in Zn2+-, Cd2+-, Pb2+-, and Hg2+-translocating ATPases.Citation38) ZosA has a SPCALVAS sequence, featuring the latter motif, consistent with a zinc transporter. Homology modeling and further optimization by MD simulation suggests that the zosA gene product can fold itself into a ternary structure that is highly similar to that of Lp-CopA as a whole, but it also has distinctive characteristic features, for example, the presence of Met residues at the entrance to the extracellular cavity and at the putative docking port for the copper-chaperone on the intracellular side. The thioether moiety of Met88 and Met612 may provide high affinity for copper that enters the channel cavity, and the SPC motif at the bottom of the cavity would have even higher affinity due to the thiol group, which swings by the mechanical motion induced by phosphorylation and hydrolysis in the cytosolic P and N domains.
Although the copYABZ operon in E. hirae has served as a paradigm for elucidating the mechanism for the safe management of copper,Citation4) the model is not applicable to some human pathogenic bacteria that have a copYAZ-type operon. A gene for copper import is rarely included in those copper operons. The zosA mutant of B. subtilis 168 may serve as a complementation host for identifying a copper-uptake gene from other bacteria. This expectation is based on our observation that the phenotypic effects of the zosA mutation were clearly specific to copper among other metals. In fact, searching for genes homologous to zosA in those pathogenic bacteria suggested the presence of several candidate CPx-ATPase genes: SPs0772 in S. pyogenes, AAN59655.1 in S. mutans, and NP_814505 in the vancomycin-resistant E. faecalis. These genes are located far from their copYAZ operons and some of them are considered to be Cd2+ or other heavy metal transporters.
Our conclusion that the zosA gene is involved in copper uptake in B. subtilis is based on the following lines of evidence: (i) the zosA gene was among the genes that were >10-fold up-regulated in response to 0.1% dl-penicillamine, (ii) the zosA mutant was sensitive to dl-penicillamine, (iii) the zosA mutant was sensitive to copper limitation even in the absence of dl-penicillamine but not sensitive to the limitation of other metals such as zinc and manganese, and (iv) the zosA mutation remarkably elevated the tolerance to CuSO4 but not to ZnSO4 both in synthetic and LB media. Homology modeling and further optimization by the molecular dynamics simulation elucidated the ternary model of ZosA and predicted the position of several candidate amino acid residues that possibly contribute to copper uptake. The hypothetical model will be useful for investigating the molecular function of the metal transporter. Our study does not necessarily exclude the original findings that the gene plays a role as a zinc-uptake ATPase under oxidative stress, nor does oppose to a recent report on possible involvement in Fe(II) efflux,Citation39) and further study is still required to conclude the molecular function of this metal transporter.
Author contributions
Takahiro Fukuhara, Yousuke Kanayama, and Taeko Kondo performed experiments. Naoki Tsunekawa wrote script files and programs for MD simulation. Shu-ichi Enomoto, Michiko Nemoto, Naotake Ogasawara, and Kenji Ingaki contributed analysis and discussion. Kazuo Kobayashi and Takashi Tamura designed the project and wrote the manuscript.
Funding
The authors are grateful to PRESTO, JST, and the Research Core for Interdisciplinary Sciences (RCIS), Okayama University for financial support. The present study was also supported in part by the Center for Information Technology and Management, Okayama University.
Disclosure statement
No potential conflict of interest was reported by the authors.
References
- Ferretti JJ, McShan WM, Ajdic D, et al. Complete genome sequence of an M1 strain of Streptococcus pyogenes. Proc. Natl. Acad. Sci. 2001;98:4658–4663.10.1073/pnas.071559398
- Bolotin A, Quinquis B, Renault P, et al. Complete sequence and comparative genome analysis of the dairy bacterium Streptococcus thermophilus. Nat. Biotechnol. 2004;22:1554–1558.10.1038/nbt1034
- Klotz L-O, Weser U. Biological chemistry of copper compounds. In: Rainsford KD, editor. Copper and Zinc in inflammatory and degenerative diseases. Dordrecht: Kluwer Academic Pubrishers; 1998. p. 19–46.10.1007/978-94-011-3963-2
- Solioz M, Stoyanov JV. Copper homeostasis in Enterococcus hirae. FEMS Microbiol. Rev. 2003;27:183–195.10.1016/S0168-6445(03)00053-6
- Odermatt A, Krapf R, Solioz M. Induction of the putative copper ATPases, CopA and Copb, of Enterococcus hirae by Ag+ and Cu2+, and Ag+ extrusion by CopB. Biochem. Biophys. Res. Commun. 1994;202:44–48.10.1006/bbrc.1994.1891
- Beres SB, Sylva GL, Barbian KD, et al. Genome sequence of a serotype M3 strain of group A Streptococcus: phage-encoded toxins, the high-virulence phenotype, and clone emergence. Proc. Natl. Acad. Sci. 2002;99:10078–10083.10.1073/pnas.152298499
- Tettelin H, Masignani V, Cieslewicz MJ, et al. Complete genome sequence and comparative genomic analysis of an emerging human pathogen, serotype V Streptococcus agalactiae. Proc. Natl. Acad. Sci. 2002;99:12391–12396.10.1073/pnas.182380799
- Ajdic D, McShan WM, McLaughlin RE, et al. Genome sequence of Streptococcus mutans UA159, a cariogenic dental pathogen. Proc. Natl. Acad. Sci. 2002;99:14434–14439.10.1073/pnas.172501299
- Paulsen IT, Banerjei L, Myers GSA, et al. Role of mobile DNA in the evolution of vancomycin-resistant Enterococcus faecalis. Science. 2003;299:2071–2074.10.1126/science.1080613
- Lowe AM, Beattie DT, Deresiewicz RL. Identification of novel staphylococcal virulence genes by in vivo expression technology. Mol. Microbiol. 1998;27:967–976.10.1046/j.1365-2958.1998.00741.x
- Rey MW, Ramaiya P, Nelson BA, et al. Complete genome sequence of the industrial bacterium Bacillus licheniformis and comparisons with closely related Bacillus species. Genome Biol. 2004;5:R77.10.1186/gb-2004-5-10-r77
- Smaldone GT, Helmann JD. CsoR regulates the copper efflux operon copZA in Bacillus subtilis. Microbiology. 2007;153:4123–4128.10.1099/mic.0.2007/011742-0
- Chillappagari S, Miethke M, Trip H, et al. Copper acquisition is mediated by YcnJ and regulated by YcnK and CsoR in Bacillus subtilis. J. Bacteriol. 2009;191:2362–2370.10.1128/JB.01616-08
- Zhang XX, Rainey PB. Regulation of copper homeostasis in Pseudomonas fluorescens SBW25. Environ. Microbiol. 2008;10:3284–3294.10.1111/emi.2008.10.issue-12
- Hirooka K, Edahiro T, Kimura K, et al. Direct and indirect regulation of the ycnKJI operon involved in copper uptake through two transcriptional repressors, YcnK and CsoR, in Bacillus subtilis. J. Bacteriol. 2012;194:5675–5687.10.1128/JB.00919-12
- Zhou L, Singleton C, Le Brun NE. High Cu(I) and low proton affinities of the CXXC motif of Bacillus subtilis CopZ. Biochem. J. 2008;413:459–465.10.1042/BJ20080467
- Walshe JM. Penicillamine: a new oral therapy of Wilson’s disease. Am. J. Med. 1956;21:487–495.10.1016/0002-9343(56)90066-3
- Doornbos DA, Faber JS. Studies on metal complexes of drugs; D-penicillamine and N-acetyl-D-penicillamine. Pharm. Weekbl. 1964;99:289–309.
- Gaballa A, Helmann JD. A peroxide-induced zinc uptake system plays an important role in protection against oxidative stress in Bacillus subtilis. Mol. Microbiol. 2002;45:997–1005.10.1046/j.1365-2958.2002.03068.x
- Kobayashi K, Ehrlich SD, Albertini A, et al. Essential Bacillus subtilis genes. Proc. Natl. Acad. Sci. 2003;100:4678–4683.10.1073/pnas.0730515100
- Yoshida K, Kobayashi K, Miwa Y, et al. Combined transcriptome and proteome analysis as a powerful approach to study genes under glucose repression in Bacillus subtilis. Nucleic Acids Res. 2001;29:683–692.10.1093/nar/29.3.683
- Ogura M, Yamaguchi H, Yoshida K, et al. DNA microarray analysis of Bacillus subtilis DegU, ComA and PhoP regulons: an approach to comprehensive analysis of B. subtilis two-component regulatory systems. Nucleic Acids Res. 2001;29:3804–3813.10.1093/nar/29.18.3804
- Kobayashi K, Ogura M, Yamaguchi H, et al. Comprehensive DNA microarray analysis of Bacillus subtilis two-component regulatory systems. J. Bacteriol. 2001;183:7365–7370.10.1128/JB.183.24.7365-7370.2001
- Kobayashi K. Bacillus subtilis pellicle formation proceeds through genetically defined morphological changes. J. Bacteriol. 2007;189:4920–4931.10.1128/JB.00157-07
- Labute P. Protonate3D: assignment of ionization states and hydrogen coordinates to macromolecular structures. Proteins. 2009;75:187–205.10.1002/prot.22234
- Labute P. The generalized Born/volume integral implicit solvent model: Estimation of the free energy of hydration using London dispersion instead of atomic surface area. J. Comput. Chem. 2008;29:1693–1698.10.1002/jcc.v29:10
- Gourdon P, Liu XY, Skjørringe T, et al. Crystal structure of a copper-transporting PIB-type ATPase. Nature. 2011;475:59–64.10.1038/nature10191
- Lüthy R, Bowie JU, Eisenberg D. Assessment of protein models with three-dimensional profiles. Nature. 1992;356:83–85.10.1038/356083a0
- Wiederstein M, Sippl MJ. ProSA-web: interactive web service for the recognition of errors in three-dimensional structures of proteins. Nucleic Acids Res. 2007;35:W407–W410.10.1093/nar/gkm290
- Doornbos DA. Stability constants of metal complexes of L-cysteine, D-penicillamine, N-acetyl-D-penicillamine and some biguanides. Determination of stoichiometric stability constants by an accurate method for pH measurement. Pharm. Weekbl. 1968;103:1213–1227.
- Gaballa A, Helmann JD. Identification of a zinc-specific metalloregulatory protein, Zur, controlling zinc transport operons in Bacillus subtilis. J. Bacteriol. 1998;180:5815–5821.
- Que Q, Helmann JD. Manganese homeostasis in Bacillus subtilis is regulated by MntR, a bifunctional regulator related to the diphtheria toxin repressor family of proteins. Mol. Microbiol. 2000;35:1454–1468.
- Helmann JD. Specificity of metal sensing: iron and manganese homeostasis in Bacillus subtilis. J. Biol. Chem. 2014;289:28112–28120.10.1074/jbc.R114.587071
- Toyoshima C, Sasabe H, Stokes DL. Three-dimensional cryo-electron microscopy of the calcium ion pump in the sarcoplasmic reticulum membrane. Nature. 1993;362:467–471.
- Toyoshima C, Nakasako M, Nomura H, et al. Crystal structure of the calcium pump of sarcoplasmic reticulum at 2.6 Å resolution. Nature. 2000;405:647–655.10.1038/35015017
- Vulpe C, Levinson B, Whitney S, et al. Isolation of a candidate gene for Menkes disease and evident that it encodes a copper-transporting ATPase. Nat. Genet. 1993;3:7–13.
- Tanzi RE, Petrukhin K, Chernov I, et al. The Wilson disease gene is a copper transporting ATPase with homology to the Menkes disease gene. Nat. Genet. 1993;5:344–350.10.1038/ng1293-344
- Tottey S, Rich PR, Rondet SAM, et al. Two Menkes-type ATPases supply copper for photosynthesis insynechocystis PCC 6803. J. Biol. Chem. 2001;276:19999–20004.10.1074/jbc.M011243200
- Guan G, Pinochet-Barros A, Gaballa A, et al. PfeT, a P1B4 -type ATPase, effluxes ferrous iron and protects Bacillus subtilis against iron intoxication. Mol. Microbiol. 2015;98. doi: 10.1111/mmi.13158.