Abstract
μ1-Adaptin of adaptor protein (AP) 1 complex, AP1M, is generally accepted to load cargo proteins into clathrin-coated vesicles (CCVs) at the trans-Golgi network through its binding to cargo-recognition sequences (CRSs). Plant vacuolar-sorting receptors (VSRs) function in sorting vacuolar proteins, which are reportedly mediated by CCV. We herein investigated the involvement of CRSs of Arabidopsis thaliana VSR4 in the sorting of VSR4. The results obtained showed the increased localization of VSR4 at the plasma membrane or vacuoles by mutations in CRSs including the tyrosine-sorting motif YMPL or acidic dileucine-like motif EIRAIM, respectively. Interaction analysis using the bimolecular fluorescence complementation (BiFC) system, V10-BiFC, which we developed, indicated an interaction between VSR4 and AP1M2, AP1M type 2, which was attenuated by a YMPL mutation, but not influenced by an EIRAIM mutation. These results demonstrated the significance of the recognition of YMPL in VSR4 by AP1M2 for the post-Golgi sorting of VSR4.
Graphical abstract
Clathrin AP1M2-recognizable tyrosine motif for plant VSR4 sorting.
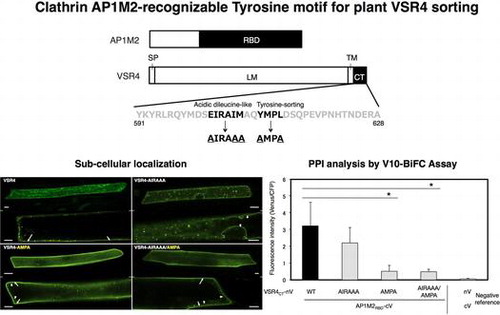
Plant clathrin-coated vesicles (CCVs) mainly function to sort proteins de novo synthesized in the endoplasmic reticulum (ER) from the trans-Golgi network (TGN) into late endosomes (LEs), leading to vacuoles, called the vacuolar pathway, and also internalize integral membrane proteins located on the plasma membrane (PM) into early endosomes (EEs) in a mode of endocytosis called the endocytic pathway.Citation1) The clathrin coat in animals is mainly composed of a heterotetrameric adaptor protein (AP) complex together with a microscopically visible structure with a three-legged shape, called the triskelion.Citation2) The triskelion comprises three clathrin heavy chains, each of which harbors a single clathrin light chain, which has been suggested to play a role in the interaction between triskelions.Citation3) On the other hand, the AP complex has five isoforms (AP1–5), each comprising heterotetrameric polypeptides including two large subunits (γβ1, αβ2, δβ3, εβ4, and ζβ5), a single medium one (μ1–μ5), and a single small one (σ1–σ5), and each AP complex has been shown to function in protein trafficking at a distinct location.Citation4) A previous study demonstrated that AP-1 localized at the TGN and was mainly involved in trafficking between the TGN and LEs.Citation5) AP-2 facilitates clathrin-mediated endocytosis from the PM. Each AP complex harbors subunits responsible for the packaging of cargo proteins into CCV via binding to the cargo-recognition sequences (CRSs) present in the cargo.Citation6) Some CRSs are known to serve as the binding site of AP complex subunits. A previous study showed that the tyrosine-sorting motif (YxxΦ; x means any amino acid, while Φ is a hydrophobic amino acid, including isoleucine, leucine, methionine, phenylalanine, or valine), is generally accepted to function as CRSs recognized by CCV in order to sort cargo into endosomes, and the dileucine (LL) or acidic LL motif ([D/E]xxxL[L/I/M]; the first amino acid is aspartic acid or glutamic acid and the final amino acid is leucine, isoleucine, or methionine) also reportedly participates in the interaction between clathrin cargo and a subunit of the clathrin AP complex in animals.Citation7) For example, the consensus tyrosine-sorting motif from a pea vacuolar-sorting receptor (VSR)-PS1 that recognizes the μ adaptin of the AP complex from Arabidopsis thaliana in vitro.Citation8) However, the mechanisms underlying the sorting of cargo into endosomes mediated by clathrin–AP complexes in plant cells have not yet been elucidated in detail.
Previous studies reported that plant clathrin–AP1 complex participates in sorting proteins at the TGN/EE.Citation5,9,10) Plant AP1 is considered to play a role in the vacuolar pathway based on similarities in its function to that of mammalian AP1. AP1 is known to be involved in the recycling of membrane proteins such as auxin efflux carrier PIN1 back to the PM.Citation10) Therefore, plant AP1 acts in dual trafficking, including vacuolar and endocytic pathways. However, the mechanism responsible for this dual function remains unclear. Plant VSRs have been shown to participate in the sorting of soluble vacuolar proteins from the ER to vacuoles via the Golgi through the action of coated vesicles.Citation1,11) Plant VSRs have been assumed to be transported by CCV since mannose-6 phosphate receptors for soluble lysosomal proteins in animal are trafficked from the TGN to the LEs by CCV through the recognition of their CRSs by AP1.Citation12) Previous studies investigated the involvement of AP1 in the sorting of VSRs.Citation13–15) The CRSs found in the cytosolic region of VSRs were found to play a role in the sorting of VSRs, with some being similar to AP1-recognizable CRSs such as the tyrosine-sorting motif and acidic LL-like motif. However, the roles of these CRSs in the sorting of VSRs are still unknown.
A recent study implicated plant CRSs in the endosomal sorting of the pea vacuolar sorting receptor BP80.Citation13) In that study, the BP80 mutant Y612 with the mutated tyrosine-sorting motif AMPL increased the localization of BP80 at the PM, while the mutant IMAA with the replacement of dipeptide I608M609, a part of the acidic LL-like motif EIRAIM, with AA caused the predominant accumulation of BP80 in vacuoles by using a transient expression system in tobacco through agroinfiltration. However, the abnormal localization of BP80 triggered by the mutation and the molecular machinery underlying changes in its endosomal sorting has yet to be elucidated in detail. A. thaliana has seven VSRs, which are reportedly involved in the post-Golgi sorting of soluble vacuolar proteins.Citation11) VSR4, which has the closest homology to BP80 among the seven VSRs, was shown to be colocalized with BP80 in tobacco epidermal cells using transient expression with agroinfiltration.Citation13) However, the precise subcellular localization of VSR4 and the relevance of CRSs and their interactors in the localization of VSR4 have yet to be established.
In the present study, we investigated the subcellular localization of a series of VSR4 variants with mutations in their CRSs in order to elucidate the molecular mechanism underlying the sorting of VSR4 through these CRSs. We also examined the involvement of the AP responsible for the post-Golgi sorting of VSR4 through the recognition of CRSs. The results obtained showed the involvement of the tyrosine-sorting motif and acidic LL-like motif in VSR4 sorting and suggested the significance of the tyrosine-sorting motif YMPL for governing the post-Golgi sorting of VSR4 mediated by AP1M2, a medium subunit type 2 of clathrin–AP complex 1.
Materials and methods
Materials
ReverTra Ace α® was purchased from Toyobo (Osaka, Japan). BP clonase II and LR clonase II were from Invitrogen (Carlsbad, CA, USA). Phusion Hot Start II High-Fidelity DNA Polymerase and fetal bovine serum were supplied by Thermo Fisher Scientific (Waltham, MA, USA). Dulbecco’s modified Eagle’s medium was obtained from PAA Laboratories (Dartmouth, MA, USA). The following antibodies were purchased: anti-GFP from Nacalai Tesque (Kyoto, Japan) and anti-FLAG from Sigma-Aldrich (St. Louis, MO, USA). All other chemicals were of reagent or tissue culture grade.
Plasmid construction
All plasmids used for subcellular localization, bimolecular fluorescence complementation (BiFC) and coimmunoprecipitation (co-IP) analyses were constructed using the Gateway cloning method. The probes of A. thaliana VSR4 and AP1M2 (At2g14720 and At1g60780, respectively) used for these analyses were shown in Fig. . cDNAs used for PCR were synthesized by ReverTra Ace α® using total RNA from A. thaliana leaves as a template according to the manufacturer’s instructions (Toyobo). In PCR, Phusion Hot Start II High-Fidelity DNA Polymerase was used to make amplified products with blunt ends. All primers used to construct plasmids were listed in Table S1. The corresponding CDSs to the CDS entry clones of VSR4, VSR4CT, AP1M2, AP1M2RBD, and AP1M2ΔRBD were amplified from the cDNAs of VSR4 and AP1M2, respectively, using adaptor PCR with a pair of attB1-F and attB2-R primers after PCR with the following primers: VSR4-F and VSR4-R (for VSR4), VSR4CT-F and VSR4-R (for VSR4CT), AP1M2-F and AP1M2-R (for AP1M2), AP1M2RBD-F and AP1M2-R (for AP1M2RBD), and AP1M2-F and AP1M2∆RBD-R (for AP1M2∆RBD) (Table S1). The amplified DNA fragments were cloned into pDONR201 (Invitrogen) by a BP reaction according to the manufacturer’s instructions (Invitrogen) in order to construct the corresponding pDONR201/VSR4, pDONR201/VSR4CT, pDONR201/AP1M2, pDONR201/AP1M2RBD, and pDONR201/AP1M2∆RBD entry clones. The entry clones for VSR4CT-AMPA and VSR4CT-AIRAAA were constructed by a BP reaction following the amplification of the corresponding mutated CDSs from pDONR201/VSR4CT by overlapping PCR using VSR4CT-F, VSR4CT-AMPA-F, VSR4CT-AIRAAA-F, and VSR4-R primers (Table S1). In order to construct the entry clones of VSR4CT-AIRAAA/AMPA, pDONR201/VSR4CT-AIRAAA was used as a template for PCR with VSR4CT-AIRAAA/AMPA-IF and VSR4CT-AIRAAA/AMPA-IR primers (Table S1). The entry clones for VSR4-AMPA, VSR4-AIRAAA, and VSR4-AIRAAA/AMPA were constructed by a BP reaction, following the amplification of the corresponding CDSs by PCR using a VSR4-F primer and the DNA fragment amplified from pDONR201/VSR4CT-AMPA, pDONR201/VSR4CT-AIRAAA, or pDONR201/VSR4CT-AIRAAA/AMPA by PCR with the VSR4CT-AIRAAA-F or VSR4CT-AMPA-F primer with VSR4-R primers (Table S1). The resultant plasmids were subjected to an LR reaction with destination vectors for subcellular localization, BiFC, and co-IP analyses in order to construct the corresponding expression vectors. In the subcellular localization analysis, a series of organellar markers such as SYP32 (At3g24350, for the Golgi), SYP61 (At1g28490, for the TGN), PEP12 (At5g16830, for LEs), VAM3 (At5g46860, for the vacuolar membrane (VM)), and SYP132 (At5g08080, for the PM) were also generated in order to determine the subcellular localization of VSR4 and its mutants.Citation16) These marker genes were amplified from the corresponding cDNAs using gene-specific primers, followed by cloning into pDONR201 (Invitrogen) by a BP reaction (Table S1). The resultant plasmids were subjected to an LR reaction with red fluorescent protein (RFP)-tagged destination vectors, such as pB5tdGW (for tdTomato fusion) and pB5mCGW (for mCherry fusion), to construct the corresponding expression vectors. Regarding the BiFC analysis, we recently made improved Gateway BiFC vectors for the high-throughput protein–protein interaction (PPI) analysis, named the V10-BiFC system.Citation3) Furthermore, we slightly modified the V10-BiFC vectors in this study as follows: pGWnV4 and pGWcV2 vectors were newly created by replacing the 3′-terminal T of attR2 sequences with C in pGWnV3 and pGWcV by PCR in order to remove the predicted tyrosine-sorting motif responsible for the interaction between μ-adaptin and a cargo protein, respectively.Citation6) These BiFC vectors were available for the expression of a protein (named X) fused with a split monomeric Venus fragment driven by the cauliflower mosaic virus (CaMV) 35S promoter, pGWnV4 for X-nV, and pGWcV2 for X-cV. These BiFC vectors were used to construct plasmids for a plant PPI analysis by BiFC in the transient expression system through biolistic bombardment. The complete nucleotide sequences of the BiFC vectors, such as pGWnV4 and pGWcV2, were registered in GenBank/EMBL/DDBJ as LC030200 and LC030201, respectively. The V10-BiFC plasmids including pnVGW3, pcVGW, pGWnV4, and pGWcV2 were used to construct plant expression vectors, such as pGWnV4/VSR4CT, pGWcV2/AP1M2RBD, and pGWcV2/AP1M2∆RBD, for the BiFC analysis. Furthermore, a pUGW2C vector was used as a Gateway destination vector in competitive BiFC in order to create expression vectors for competitors including VSR4CT, AP1M2, AP1M2RBD, or AP1M2∆RBD.Citation3) Mammalian Gateway-compatible vectors, such as pEGFPGW and p3xFLAGGW, for fusion with a green fluorescent protein (GFP) and 3x FLAG were used to construct mammalian expression vectors including pEGFPGW/VSR4CT, pEGFPGW/VSR4CT-AIRAAA, pEGFPGW/VSR4CT-AMPA, pEGFPGW/VSR4CT-AIRAAA/AMPA, or p3xFLAGGW/AP1M2RBD for co-IP and immunoblotting analyses.Citation3)
Fig. 1. Mapping probes used in the subcellular localization of Arabidopsis VSR4, and BiFC and co-IP analyses on the Arabidopsis VSR4 and AP1M2 interaction.
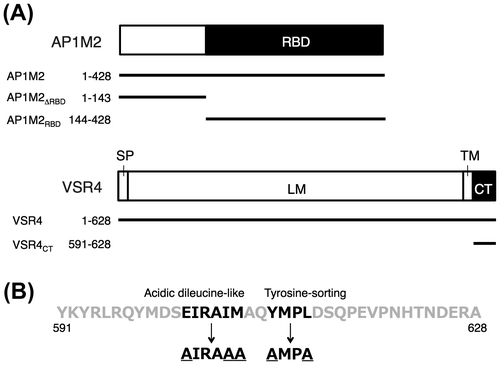
Microscopic analysis
Plasmids encoding AtVSR4 or its mutants fused to sGFP together with or without RFP fusion of an organellar marker were transiently transformed into A. thaliana leaf cells or Japanese leek epidermal cells by biolistic bombardment in order to determine subcellular localization.Citation3) A total of 0.5 μg of the plasmids encoding the AtVSR4 variants fused to sGFP driven by the CaMV 35S promoter was mixed with or without equal amounts of each organellar marker plasmid and was absorbed onto tungsten particles (1.0 μm in diameter) according to the manufacturer′s instructions (TANAKA, Sapporo, Japan). A BiFC assay was performed to analyze PPI by biolistic bombardment as previously reported. Following bombardment, the cells were incubated on the same plate overnight at 22 °C in darkness and the fluorescence proteins transformed were viewed with a TCS SP5 confocal laser scanning microscope (Leica Microsystems, Wetzler, Germany) using a HCX IRAPO L 25.0 × 0.95 WATER objective lens for the subcellular localization analysis or a HCX PL APO CS 20.0 × 0.70 IMM UV lens for the BiFC assay. sGFP fusions were excited with an argon laser line (488 nm) to detect fluorescence at 500–530 nm, while the RFP fusions were excited with a He/Ne laser line (543 nm) to view fluorescence at 565–615 nm. BiFC of the split Venus fused with proteins of interest was excited with an argon laser line (514 nm) to detect fluorescence at 520–555 nm, while ECFP was excited with the same argon laser line (458 nm) to capture fluorescence at 465–500 nm. Dual-fluorescence imaging was performed using the sequential scanning mode. The determination of cellular BiFC levels and colocalization between VSR4 series and organellar markers was quantified using Leica LAS AF software according to the manufacturer′s instructions (Leica Microsystems).
Protein extraction and immunoblotting following immunoprecipitation (IP)
293T cells were lysed in lysis buffer (50 mM HEPES-NaOH, pH 7.5, 20 mM MgCl2, 150 mM NaCl, 1 mM dithiothreitol, 1 mM phenylmethane sulfonylfluoride, 1 mg/ml leupeptin, 1 mM EDTA, 1 mM Na3VO4, and 10 mM NaF) containing detergents (0.5% NP-40 and 1% CHAPS). Unless otherwise indicated, all steps were performed at 4 °C. Proteins in centrifuged cell supernatants or immunoprecipitates, which were collected with a Santa Cruz ImmunoCruz kit (Santa Cruz, CA, USA), were heat-denatured. These samples were immunoblotted using the Bio-Rad Turbo Blot Transfer System (Hercules, CA, USA) with Turbo Blot reagents (Bio-Rad). The transferred membranes were blocked with a Blocking One reagent (Nacalai Tesque, Kyoto, Japan) and immunoblotted using a primary antibody, followed by a peroxidase-conjugated secondary antibody (Nacalai Tesque). The bound antibodies were detected using an ImmunoStar Zeta detection kit (Wako, Osaka, Japan).
Statistical analysis
Each experiment was performed 2–4 times under conditions in which the bombardments tested showed no detectable cytotoxicity. The significance of differences between two groups was determined by the Student′s t-test.
Results
Effects of mutations in predicted CRSs of VSR4 on its subcellular localization in A. thaliana leaf epidermal cells
Plant VSRs are reported to capture vacuolar proteins at the ER, transporting them to vacuoles by means of vesicle-trafficking machinery.Citation1,11) However, the molecular mechanism underlying the sorting of VSRs by coated vesicles remains unclear in plant cells. A. thaliana VSRs have at least two types of predicted sorting sequences in its cytosolic tail region (VSR4CT), and sequences such as the tyrosine-sorting motif and acidic LL-like motif were previously shown to be involved in vesicle trafficking mediated by CCVs in animals (Fig. (B)).Citation13) The tyrosine-sorting motif (YxxΦ) in a clathrin cargo protein has been shown to play a role in its recognition by μ-adaptin of the clathrin AP complex, while LL or the acidic LL motif ([D/E]xxxL[L/I/M]) of a cargo protein was also implicated in the interaction between clathrin cargo and a subunit of the clathrin–AP complex in animals.Citation6,7) These findings prompted us to investigate the involvement of these CRSs in the subcellular localization of A. thaliana VSR4, which was previously reported to localize at cellular punctate structures and the PM.Citation13) We initially examined the effects of mutations in CRSs such as the tyrosine-sorting motif or acidic LL-like motif on the subcellular localization of A. thaliana VSR4. VSR4 with the replacement of E, I, and M at 602, 606, and 607 of the LL-like motif EIRAIM with A (VSR4-AIRAAA) appeared to be localized at punctate structures and around the PM in Arabidopsis leaf epidermal cells, similar to the localization of VSR4 without mutations (Fig. ). In contrast, VSR4 with the substitution of Y and L at 610 and 613 of the tyrosine-sorting motif YMPL with A (VSR4-AMPA) or mutations to both motifs (VSR4-AIRAAA/AMPA) appeared to be markedly localized in the PM, and less in cellular punctate structures. These results suggested the involvement of the predicted CRSs of VSR4 in its subcellular localization in Arabidopsis leaf cells.
Fig. 2. Transient expression of VSR4 and their mutants fused to sGFP in Arabidopsis leaf epidermal cells.
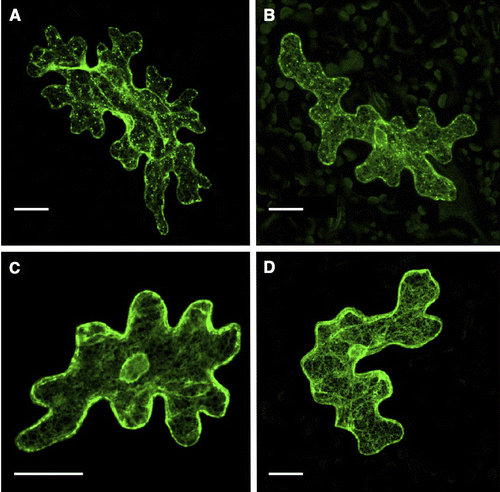
Determination of the subcellular localization of VSR4 variants in Japanese leek epidermal cells
In order to more precisely determine the localization of VSR4 with or without CRS mutations, we analyzed the colocalization of VSR4 variants with typical organellar markers through biolistic bombardment. We attempted to perform an analysis in which the VSR4 variants were transiently expressed in Japanese leek epidermal cells instead of Arabidopsis leaf epidermal cells by bombardment because the shape of leek cells was morphologically simpler than that of leaf pavement cells. Furthermore, we successfully characterized the assembly of A. thaliana SEC13 and SEC31 proteins, coat subunits of the COPII vesicle machinery, using this system.Citation17) We initially observed marked differences in localization between VSR4 and its mutants in Japanese leek epidermal cells (Fig. ). Although differences in localization between VSR4 and VSR4-AIRAAA were less in Arabidopsis leaf epidermal cells, VSR4 expressed in Japanese leek epidermal cells localized at punctate structures and occasionally at the PM, while VSR4-AIRAAA localized in punctate structures and also appeared to be predominantly present at the VM, but rarely at the PM. On the other hand, VSR4-AMPA and VSR4-AIRAAA/AMPA in the leek cells both apparently localized at the PM with a subpopulation of cellular punctate structures, similar to that in Arabidopsis leaf cells. These mutants also slightly localized at the VM. These results suggested the relevance of the CRSs of VSR4 to the subcellular localization of VSR4. We then attempted to confirm the subcellular localization of VSR4 variants using organellar markers in order to further clarify the effects of the mutation of CRSs on the subcellular localization of VSR4. In the PM, VSR4, VSR4-AMPA, and VSR4-AIRAAA/AMPA fused to sGFP colocalized with the PM marker, SYP132 fused to mCherry, at a wide range of levels, while the VSR4-AIRAAA fusion was rarely detected at the PM (Fig. I). Furthermore, VSR4-AIRAAA, VSR4-AMPA, and VSR4-AIRAAA/AMPA colocalized with the VM marker, VAM3 fused to mCherry while VSR4 without substitution was hardly detected at the VM (Fig. ). Among the VSR4 variants tested, VSR4-AIRAAA most predominantly localized at the VM. These results were supported by the quantitative analysis of the colocalization between VSR variants and each organellar marker (Figs. II and II). Regarding cellular punctate structures, any VSR4 variants were rarely colocalized with the Golgi marker, SYP32 fused to tdTomato, while each VSR4 variant with or without a mutation had a population of punctate structures colocalized with the TGN marker, tdTomato-SYP61 fusion, or LE marker, tdTomato-PEP12 fusion with a broad range of colocalization frequencies (Supplemental Figures S1–S3). The qualitative localization analysis of these cellular punctuate structures was strengthened by the quantitative colocalization analysis based on the values of Pearson′s correlation between VSR variants and the organellar markers (Figs. S1II, S2II, and S3II). Therefore, the results obtained suggested the involvement of the tyrosine-sorting motif and acidic LL-like motif in the post-Golgi transport of VSR4.
Fig. 3. Transient expression of VSR4 and their mutants fused to sGFP in Japanese leek epidermal cells.
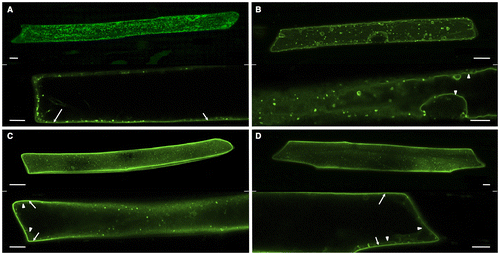
Fig. 4. Colocalization analysis of VSR4 and their mutants fused to sGFP with a PM marker transiently expressed in leek epidermal cells.
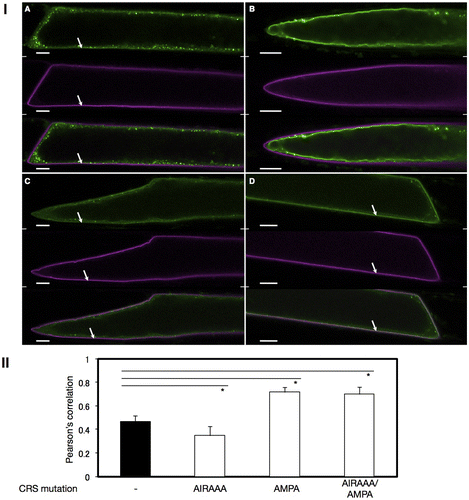
Fig. 5. Colocalization analysis of VSR4 and their mutants fused to sGFP with a VM marker transiently expressed in leek epidermal cells.
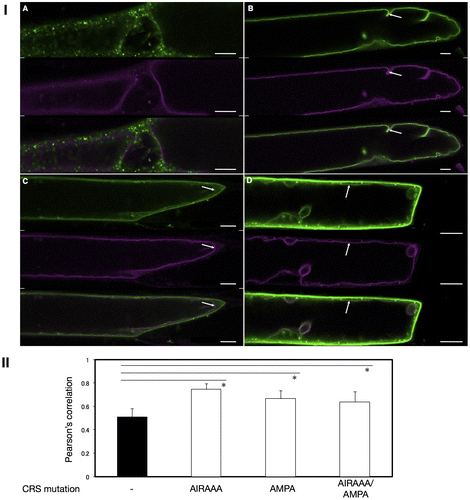
PPI analysis of AP1M2 and VSR4CT by the V10-BiFC system
We investigated the involvement of the cargo-recognition protein responsible for the post-Golgi sorting of VSR4. A medium subunit of clathrin–AP complex 1 (AP1), μ1-adaptin AP1M, is generally accepted to load VSRs into CCV at the TGN in plant cells.Citation5) However, the mechanisms responsible for the AP1M-mediated sorting of VSRs have not yet been elucidated in detail. Therefore, we herein attempted to investigate the interaction between AP1M and VSR4 in living plant cells. We recently generated improved Gateway-compatible BiFC vectors for a high-throughput PPI analysis, named the V10-BiFC system.Citation3) In the present study, we slightly modified V10-BiFC vectors and newly created pGWnV4 and pGWcV2 vectors in order to remove the predicted tyrosine-sorting motif from the vectors, which is reportedly responsible for the interaction between μ-adaptin and a clathrin cargo protein.Citation6) As for AP1M, A. thaliana has two types of AP1M, type 2, which is reported to be expressed ubiquitously in cells.Citation5) Therefore, we investigated the interaction between AP1M2 and VSR4 from A. thaliana using the V10-BiFC system. The cytosolic tail region of VSR4 (VSR4CT) harbored the predicted CRSs including the tyrosine-sorting motif and acidic LL-like motif, which was accessed by cytosolic AP1M2 at the TGN. We performed a BiFC analysis to examine the interaction between AP1M2 and VSR4CT (amino acids at 591–628) in living plant cells using the V10-BiFC system. We initially performed the BiFC assay using all combinations of the split Venus fusions of AP1M2 and VSR4CT. The results obtained indicated that a significant interaction existed between several combinations of split Venus fusions used as BiFC probes (Fig. (A)). In order to evaluate the specificity of the interaction between AP1M2 and VSR4CT, we performed a competitive BiFC assay by the cobombardment of a plasmid harboring VSR4CT without a BiFC tag at 10-fold amounts of split Venus fusion vectors as a competitor.Citation3) As shown in Fig. (B), the competitor led to significant decreases in BiFC levels due to the presence of the pair of nV-AP1M2 and VSR4CT-cV or AP1M2-nV and VSR4CT-cV. Competitive BiFC clearly demonstrated the specific interaction between AP1M2 and VSR4CT in living plant cells, which was not caused by the nonspecific interaction between split Venus tags.
Fig. 6. Interaction between AP1M2 and VSR4CT by a BiFC assay.
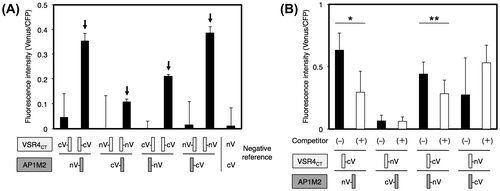
Interaction between AP1M2 and VSR4CT through recognition of the tyrosine-sorting motif YMPL of VSR4CT by AP1M2
VSR4CT has at least two types of predicted CRSs such as the tyrosine-sorting motif and acidic LL-like motif (Fig. (B)).Citation6) Therefore, we attempted to examine the contribution of these motifs to the AP1M2-VSR4CT interaction using the V10-BiFC system. A point mutation analysis was performed to determine whether the tyrosine-sorting motif, acidic LL-like motif, or both in VSR4CT were involved in the interaction with AP1M2. The replacement of E602, I606, and M607 of the acidic LL-like motif EIRAIM of VSR4CT with A (VSR4CT-AIRAAA) did not have an inhibitory effect on the AP1M2-VSR4CT interaction (Fig. ). However, the substitution of Y610 and L613 of the tyrosine-sorting motif YMPL in VSR4CT with A (VSR4CT-AMPA) or a mutation in both motifs (VSR4CT-AIRAAA/AMPA) caused lower BiFC levels in the interaction than that with the nonsubstitution, indicating the significant contribution of the tyrosine-sorting motif YMPL responsible for the interaction between AP1M2 and VSR4CT.
Fig. 7. Effects of mutated amino acids in predicted CRSs on the interaction between AP1M2 and VSR4CT by a BiFC assay.
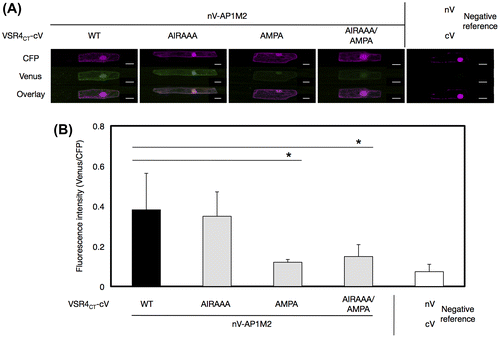
Involvement of the receptor-binding domain (RBD) of AP1M2 (AP1M2RBD) in the recognition of the tyrosine-sorting motif YMPL in VSR4CT
A. thaliana AP1M2 harbors a typical receptor-binding domain (RBD), which is responsible for the binding of a clathrin cargo protein in mammalian cells (Fig. (A)).Citation8) We examined the contribution of the RBD of AP1M2 (amino acids at 144–428), AP1M2RBD, to the interaction between AP1M2 and VSR4CT by the V10-BiFC system. The results obtained showed that AP1M2RBD strongly interacted with VSR4CT, and the specificity of this interaction was confirmed by a competitive BiFC assay, indicating the involvement of AP1M2RBD in the AP1M2RBD–VSR4CT interaction (Fig. (A)). Alternatively, we expressed AP1M2RBD and VSR4CT in mammalian 293T cells to investigate the molecular interaction of AP1M2RBD and VSR4CT using a co-IP assay. The results obtained demonstrated that AP1M2RBD formed an immune complex with VSR4CT, which revealed the involvement of AP1M2RBD in the AP1M2–VSR4CT interaction, thereby supporting the results of the BiFC assay (Supplemental Fig. S4). Furthermore, a mutation in the tyrosine-sorting motif YMPL, but not in that of the acidic LL-like motif EIRAIM, markedly decreased BiFC levels in the AP1M2RBD–VSR4CT interaction, indicating the significant contribution of the tyrosine-sorting motif YMPL, which is responsible for the interaction between AP1M2RBD and VSR4CT (Fig. (A)). As for the rest region of AP1M2, AP1M2∆RBD (amino acids at 1–143) still retained the potential to specifically interact with VSR4CT, and this was analyzed by a competitive BiFC assay (Fig. (A)). In addition, neither of the mutated motifs had an inhibitory effect on the AP1M2∆RBD–VSR4CT interaction (Fig. (B)). A mutation in the acidic LL-like motif with or without the mutated tyrosine-sorting motif led to a stronger interaction with AP1M2∆RBD than that with nonsubstitution and the AMPA mutant, suggesting other CRSs, besides the tyrosine-sorting motif, which are involved in the AP1M2–VSR4CT interaction. Taken together, these results suggested the dominant contribution of the recognition of the tyrosine-sorting motif YMPL of VSR4 by the RBD of AP1M2 to the AP1M2–VSR4 interaction.
Fig. 8. Effects of mutated amino acids in predicted CRSs on the interaction between a part of AP1M2RBD with VSR4CT by a BiFC assay.
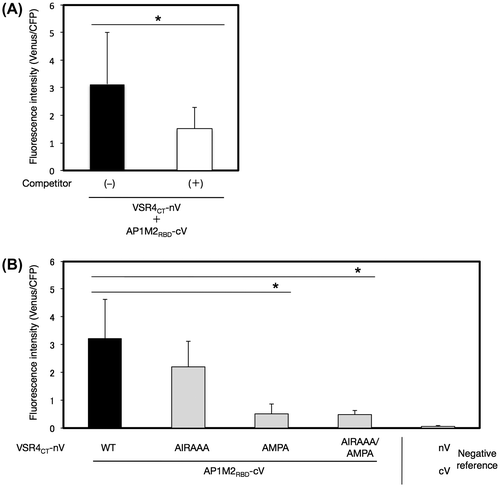
Fig. 9. Effects of mutated amino acids in predicted CRSs on the interaction between a part of AP1M2∆RBD with VSR4CT by a BiFC assay.
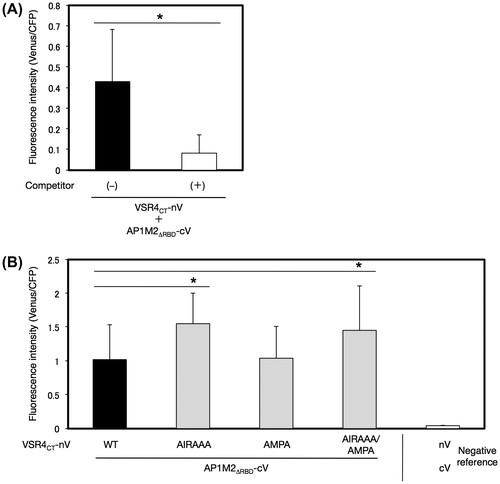
Discussion
Plant VSRs function to transport soluble vacuolar-localized proteins from the ER to the PM or vacuoles.Citation1,11) A. thaliana has seven VSRs, all of which have at least two typical CRSs including the tyrosine-sorting motif and acidic LL-like motif in the cytosolic tail region.Citation13) Several mutations in amino acid sequences including CRSs in the cytosolic regions of plant VSRs were previously shown to lead to the missorting of VSRs.Citation13,14) However, the function of CRSs in the sorting of A. thaliana VSRs remained unclear. We herein demonstrated that mutations including the tyrosine-sorting motif YMPL and LL-like motif EIRAIM of VSR4 led to the abnormal localization of VSR4, suggesting the involvement of these CRSs in the post-Golgi sorting of VSR4 (Figs. and ). Furthermore, the results obtained suggested that the tyrosine-sorting motif of VSR4 was involved in its sorting through recognition by the AP1M2 of the clathrin coatomer. Regarding plant VSRs, the CRSs in pea BP80, an ortholog of A. thaliana VSRs, were found to function in its sorting in tobacco leaf epidermal cells by using a transient expression system through an agroinfiltration analysis.Citation13) In that study, a mutation in the tyrosine-sorting motif increased the localization of BP80 at the PM, while the substitution of IM to AA in the cytosolic region led to a markedly increased localization of BP80 at vacuoles with the small population of BP80 at PM, in comparison with nonsubstitution. However, difficulties were associated with performing a detailed localization analysis in order to further determine the contribution of such sequences in the sorting of BP80 due to the complex shape of this type of tobacco leaf cell. In the present study, we analyzed the subcellular localization of A. thaliana VSR4 mutants in detail using transient expression in Japanese leek epidermal cells through bombardment and revealed the subcellular localization of VSR4 mutants more clearly and quantitatively, which demonstrated the involvement of CRSs, such as the tyrosine-sorting motif and acidic LL-like motif in the post-Golgi sorting of VSR4. Transgenic plants stably expressing VSR4 or its mutants may help in determining their precise localization and the involvement of CRSs in post-Golgi sorting in living Arabidopsis cells.
This study demonstrated that a mutation in the tyrosine-sorting motif YMPL of VSR4 increased its localization at the PM with a subpopulation of VSR4 at the VM (Figs. ). In addition, the YMPL of VSR4 had the potential to be recognized by AP1M2 (Figs. and ). Therefore, YMPL mutations may attenuate the transport of VSR4-AMPA or VSR4-AIRAAA/AMPA from the TGN to LEs through AP1M2 and enhance their accumulation at the TGN, leading to an increase in their delivery to the PM via a default secretory pathway.Citation13) These findings may explain the increased localization of VSR4-AMPA or VSR4-AIRAAA/AMPA at the PM. Regarding the subpopulation of VSR4-AMPA or VSR4-AIRAAA/AMPA at the VM, VSR4 at the PM was previously reported to have the potential to be endocytosed.Citation13) Membrane-bound receptors at the PM, such as BRASSINOSTEROID-INSENSITIVE 1 and FLAGELLIN-INSENSITIVE 2, are generally subjected to internalization by ligand-triggered endocytosis, leading to vacuoles for protein degradation via passage through EE and LEs.Citation18,19) Therefore, the subpopulation of VSR4-AMPA and VSR4-AIRAAA/AMPA at the VM may have been due to the receptor-mediated endocytosis (RME) through the involvement of other CRSs besides the tyrosine-sorting motif or acidic LL-like motif. Further analyses are needed in order to understand the molecular mechanisms underlying the RME of VSR4 mutants.
Concerning the AIRAAA mutation, a mutation in the acidic LL-like motif EIRAIM of VSR4 caused the predominant localization of VSR4 at the VM, suggesting the anterograde transport of VSR4-AIRAAA from the TGN to LEs, followed by the attenuation of its retrograde transport from LEs to the TGN, which led to the sorting to vacuoles (Figs. , , and ). The acidic LL-like motif and LL motif generally serve as CRSs for a subunit of the clathrin–AP complex in animals.Citation6) The dipeptide IM in the LL-like motif EIRAIM is known to be similar to CRSs such as WLM for retromers in animals, as previously reported.Citation20) Therefore, a defect in the recognition of VSR4-AIRAAA by retromers due to a mutation in EIRAIM may attenuate the transport of VSR4-AIRAAA from LEs to the TGN, followed by the sorting of the VSR4-AIRAAA to vacuoles, thereby potentially explaining the predominant localization of VSR4-AIRAAA at the VM. However, the plant retrograde pathway from LEs to the TGN by retromers remains a matter of debate. VSR1 was previously reported to form an immunocomplex with VSP35, a cargo-recognition subunit of retromers, which is located at LEs or the VM.Citation21) However, some subunits of retromers have been detected at the TGN, not at LEs.Citation22,23) Furthermore, the functional depletion of either a retromer subunit or VSR1/VSR3 caused the accumulation of soluble cargo of VSR1 at the ER, but not the TGN, suggesting the loading of cargo by VSRs at the ER, not the TGN.Citation11) Taken together, these findings indicate that the involvement of the retrograde pathway of VSRs by retromers at LEs to the TGN, as operated in animals, requires further study. Therefore, a PPI analysis of plant retromers with VSR4 with or without mutation may assist in elucidating the molecular mechanism governing the vacuolar sorting of VSR4-AIRAAA.
All VSR4 mutants have a subpopulation at the TGN and LEs, in contrast to VSR4, which has a major population at these organelles (Fig. S2–4). Therefore, a mutation in CRSs may affect their localization at these organelles, but not abolish it completely. The attenuation of retrograde transport from the Golgi to the ER by COP1 using disruption in the activity of Arf1 was previously reported to inhibit the anterograde pathway from the ER to the Golgi by COPII, suggesting that the anterograde and retrograde pathways influence each other.Citation24) Therefore, the retained localization of VSR4 mutants at the TGN and LEs by mutated CRSs may be explained by an interaction between the opposite pathway by CCV and retromers, as the interference of COPI and COPII pathways against the sorting of cargo proteins between the ER and Golgi.
Some AP subunits were recently shown to interact with A. thaliana VSR2 in a yeast two hybrid assay; however, the specificity of this type of PPI assay remains controversial.Citation15) Furthermore, the involvement of CRSs in the interaction between VSR2 and the AP subunit remains unclear. In the present study, the V10-BiFC system showed that AP1M2 interacted with VSR4CT, and the tyrosine-sorting motif, but not the acidic LL-like motif of VSR4CT had the potential to be recognized by the RBD of AP1M2 in living plant cells (Figs. and ). The AIRAAA mutation also enhanced the interaction between VSR4CT and AP1M2∆RBD, suggesting the involvement of other CRSs besides the tyrosine-sorting motif and acidic LL-like motif as well as the participation of other adaptor molecules in addition to the adaptin of the clathrin–AP complex in the VSR4CT–AP1M2∆RBD interaction. A PPI analysis using the gateway V10-BiFC system developed by us, along with the competitive BiFC assay and subcellular localization analysis through transient expression in Japanese leek epidermal cells are together expected to provide useful high-throughput tools for elucidating the interaction between proteins of interest, thereby rapidly presenting valuable information on PPI and subcellular localization in living cells. Therefore, a PPI analysis between other types of VSR4CT mutants and AP1M2 as well as high-throughput screening of interactors of VSR4CT by using the V10-BiFC system may elucidate the molecular mechanisms underlying the post-Golgi sorting of VSR4. Further analyses are required to uncover the post-Golgi sorting of VSR4.
Author contributions
This study is designed by Nishimura, K., Matsunami, E., Yoshida, S., Kohata, S., Yamauchi, J., Jisaka, M., Nagaya, T., Yokota, K., and Nakagawa, T. designed research; Nishimura, K., Matsunami, E., Yoshida, S., Kohata, S., and Yamauchi, J. performed research; Nishimura, K. and Matsunami, E. contributed analytical tools; Nishimura, K., Matsunami, E., and Yamauchi, J carried out data analysis; and Nishimura, K., Yamauchi, J., and Nakagawa T. wrote the paper.
Funding
This work was supported by the Japan Society for the Promotion of Science under the Japan–UK Research Cooperative Program from the Ministry of Education, Culture, Sports, Science and Technology of Japan (to KN).
Supplemental material
Supplemental material for this article can be accessed at http://dx.doi.org/10.1080/09168451.2015.1116925.
Supplementarty_material.zip
Download Zip (2.3 MB)Acknowledgments
We thank Dr. Takashi Ueda at the University of Tokyo, Dr. Roger Tsien at the University of California, and Dr. Shoji Mano at the National Institute for Basic Biology for the gift of cDNAs of organellar marker genes, red fluorescence proteins, such as tdTomato and mCherry, and destination vectors, including pB5tdGW and pB5mCGW, respectively. We are also grateful to Dr. Hideyuki Yamaguchi of Leica Microsystems for the quantification of fluorescence images.
Disclosure statement
No potential conflict of interest was reported by the authors.
Notes
Abbreviations: Ampr, ampicillin resistance marker; AP, adaptor protein; AP1M, μ1-adaptin of AP1 complex; AP1M2, AP1M type 2; BiFC, bimolecular fluorescence complementation; BP, a recombination between an att B-flanking DNA fragment and an att P-containing donor vector; CaMV, cauliflower mosaic virus; CDS, coding sequence; CCV, clathrin-coated vesicle; CLSM, confocal laser scanning microscope; COPI, coat protein I; COPII, coat protein II; CRS, cargo-recognition sequence; cV, C-terminal region (211–239) of Venus; ECFP, enhanced cyan fluorescent protein; EE, early endosome; ER, endoplasmic reticulum; GFP, green fluorescent protein; IP, immunoprecipitation; Kmr, kanamycin resistance marker; mCherry, monomeric Cherry; nV, N-terminal region (1–210) of Venus; ORF, open reading frame; P35S, 35S promoter; LR, a recombination between an att L-containing entry clone and an att R-containing destination vector; PCR, polymerase chain reaction; PM, plasma membrane; PPI, protein–protein interaction; PVDF, polyvinylidene fluoride; RFP, red fluorescent protein; RME, receptor-mediated endocytosis; SD, standard deviation; SDS-PAGE, SDS‐polyacrylamide gel electrophoresis; sGFP, synthetic GFP with S65T; SYP, syntaxin of plants; tdTomato, tandem dimer Tomato; TGN, trans-Golgi network; VM, vacuolar membrane; VSR, vacuolar-sorting receptor; YFP, yellow fluorescent protein.
References
- Robinson DG, Pimpl P. Receptor-mediated transport of vacuolar proteins: a critical analysis and a new model. Protoplasma. 2014;251:247–264.10.1007/s00709-013-0542-7
- Kirchhausen T, Owen D, Harrison SC. Molecular structure, function, and dynamics of clathrin-mediated membrane traffic. Cold Spring Harb. Perspect. Biol. 2014;6:a016725.10.1101/cshperspect.a016725
- Nishimura K, Ishikawa S, Matsunami E, et al. New Gateway-compatible vectors for a high-throughput protein-protein interaction analysis by a bimolecular fluorescence complementation (BiFC) assay in plants and their application to a plant clathrin structure analysis. Biosci. Biotechnol. Biochem. 2015;79:1995–2006.
- Hirst J, Barlow LD, Francisco GC, et al. The fifth adaptor protein complex. PLoS Biol. 2011;9:e1001170.10.1371/journal.pbio.1001170
- Park M, Song K, Reichardt I, et al. Arabidopsis μ-adaptin subunit AP1M of adaptor protein complex 1 mediates late secretory and vacuolar traffic and is required for growth. Proc. Natl. Acad. Sci. USA. 2013;110:10318–10323.10.1073/pnas.1300460110
- Bonifacino JS, Traub LM. Signals for sorting of transmembrane proteins to endosomes and lysosomes. Annu. Rev. Biochem. 2003;72:395–447.10.1146/annurev.biochem.72.121801.161800
- Traub LM. Common principles in clathrin-mediated sorting at the Golgi and the plasma membrane. Biochim. Biophys. Acta. 2005;1744:415–437.10.1016/j.bbamcr.2005.04.005
- Happel N, Höning S, Neuhaus JM, et al. Arabidopsis mu A-adaptin interacts with the tyrosine motif of the vacuolar sorting receptor VSR-PS1. Plant J. 2004;37:678–693.10.1111/tpj.2004.37.issue-5
- Teh OK, Shimono Y, Shirakawa M, et al. The AP-1 μ adaptin is required for KNOLLE localization at the cell plate to mediate cytokinesis in Arabidopsis. Plant Cell Physiol. 2013;54:838–847.10.1093/pcp/pct048
- Wang JG, Li S, Zhao XY, et al. HAPLESS13, the Arabidopsis μ1 adaptin, is essential for protein sorting at the trans-Golgi network/early endosome. Plant Physiol. 2013;162:1897–1910.10.1104/pp.113.221051
- Lee Y, Jang M, Song K, et al. Functional identification of sorting receptors involved in trafficking of soluble lytic vacuolar proteins in vegetative cells of Arabidopsis. Plant Physiol. 2013;161:121–133.10.1104/pp.112.210914
- Li YB, Rogers SW, Tse YC, et al. BP-80 and homologs are concentrated on post-Golgi, probable lytic prevacuolar compartments. Plant Cell Physiol. 2002;43:726–742.10.1093/pcp/pcf085
- Saint-Jean B, Seveno-Carpentier E, Alcon C, et al. The cytosolic tail dipeptide Ile-Met of the pea receptor BP80 is required for recycling from the prevacuole and for endocytosis. Plant Cell. 2010;22:2825–2837.10.1105/tpc.109.072215
- Foresti O, Gershlick DC, Bottanelli F, et al. A recycling-defective vacuolar sorting receptor reveals an intermediate compartment situated between prevacuoles and vacuoles in tobacco. Plant Cell. 2010;22:3992–4008.10.1105/tpc.110.078436
- Gershlick DC, Lousa Cde M., Foresti O, et al. Golgi-dependent transport of vacuolar sorting receptors is regulated by COPII, AP1, and AP4 protein complexes in tobacco. Plant Cell. 2014;26:1308–1329.10.1105/tpc.113.122226
- Uemura T, Ueda T, Ohniwa RL, et al. Systematic analysis of SNARE molecules in Arabidopsis: dissection of the post-Golgi network in plant cells. Cell Struct. Funct. 2004;29:49–65.10.1247/csf.29.49
- Hino T, Tanaka Y, Kawamukai M, et al. Two Sec13p homologs, AtSec13A and AtSec13B, redundantly contribute to the formation of COPII transport vesicles in Arabidopsis thaliana. Biosci. Biotechnol. Biochem. 2011;75:1848–1852.10.1271/bbb.110331
- Irani NG, Di Rubbo S, Mylle E, et al. Fluorescent castasterone reveals BRI1 signaling from the plasma membrane. Nat. Chem. Biol. 2012;8:583–589.10.1038/nchembio.958
- Robatzek S, Chinchilla D, Boller T. Ligand-induced endocytosis of the pattern recognition receptor FLS2 in Arabidopsis. Genes Dev. 2006;20:537–542.10.1101/gad.366506
- Seaman MN. Identification of a novel conserved sorting motif required for retromer-mediated endosome-to-TGN retrieval. J. Cell Sci. 2007;120:2378–2389.10.1242/jcs.009654
- Oliviusson P, Heinzerling O, Hillmer S, et al. Plant retromer, localized to the prevacuolar compartment and microvesicles in Arabidopsis, may interact with vacuolar sorting receptors. Plant Cell. 2006;18:1239–1252.10.1105/tpc.105.035907
- Niemes S, Langhans M, Viotti C, et al. Retromer recycles vacuolar sorting receptors from the trans-Golgi network. Plant J. 2010;61:107–121.10.1111/tpj.2009.61.issue-1
- Stierhof YD, Viotti C, Scheuring D, et al. Sorting nexins 1 and 2a locate mainly to the TGN. Protoplasma. 2013;250:235–240.10.1007/s00709-012-0399-1
- Stefano G, Renna L, Chatre L, et al. In tobacco leaf epidermal cells, the integrity of protein export from the endoplasmic reticulum and of ER export sites depends on active COPI machinery. Plant J. 2006;46:95–110.10.1111/tpj.2006.46.issue-1