Abstract
Dried spaghetti was rehydrated to its optimal cooking state, known as al dente, at 60, 80, and 100 °C, in distilled water or 0.1, 1.0, and 2.0 mol/L sodium chloride solutions. Then, the moisture distributions and stress–strain curves were examined to identify the major factors governing the texture of rehydrated spaghetti. The difference in moisture content between the inner and peripheral regions of rehydrated spaghetti and its breaking stress were greater at higher rehydration temperatures; however, rehydration temperature did not affect breaking strain. The sodium chloride concentration of the immersion solution did not affect moisture distribution or breaking stress, while breaking strain was decreased by rehydration at higher sodium chloride concentrations. The results obtained in this study suggest that moisture distribution within spaghetti and its material properties govern its breaking stress and strain, respectively.
Graphical abstract
Moisture distribution of spaghetti rehydrated to the al dente state at 60, 80, and 100 °C.
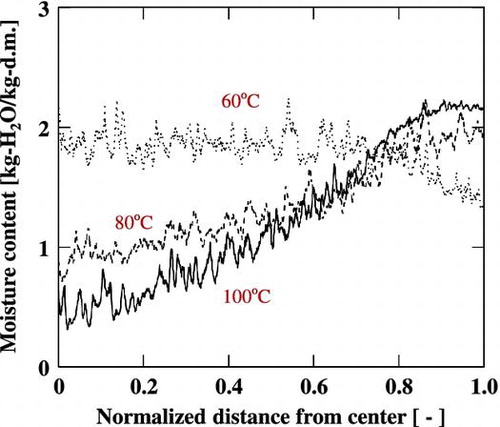
Pasta has its origins in traditional Italian cuisine. It is now eaten worldwide and is one of the most popular noodles in Japan alongside Udon and Soba, which are traditional Japanese noodles. The palatability of food is affected by its flavor, taste, and texture. Texture plays an especially important role in the palatability of noodles.
Raw materials, manufacturing conditions, drying conditions, and rehydration conditions affect the material properties of pasta, such as its starch characteristics,Citation1) degree of starch damage,Citation2) gelatinization temperature of the starch,Citation3,4) network structure of the gluten,Citation5) and the extent of protein denaturation.Citation6,7) Many researchers have reported on the effects of these material properties on pasta texture. Decreases in stickiness and increases in firmness are ascribed to the formation of large and insoluble protein aggregatesCitation8); protein content greatly affects the firmness and compressibility of pasta.Citation9)
The optimal cooked state of dried pasta is called al dente, in which the consistency of the hairsbreadth core in the center of pasta is maintained. In the al dente state, moisture distribution is not uniform: the moisture content is high and low near the surface and at the center of pasta, respectively. Thus, the texture of al dente pasta is characterized by a soft external zone and a firm core. The firm core provides a mouthfeel of resistance while biting. Irie et al. examined the relationship between the moisture distribution of pasta, as measured using magnetic resonance imaging (MRI), and its mechanical properties, as measured using a texture analyzer. They concluded that moisture distribution is the primary factor affecting the texture of rehydrated pasta.Citation10)
This indicates that both the material properties of pasta and the moisture distribution within pasta play important roles in creating the texture of rehydrated pasta. However, the specific effects of these factors on pasta texture remain unclear. One reason why the factors governing pasta texture have not been elucidated is the absence of an adequate method of measuring the precise moisture distribution with a high spatial resolution, especially when the moisture content is low. As mentioned above, MRI is a powerful method of measuring moisture distribution, but it cannot measure low moisture contents precisely. We developed a novel method of measuring the moisture distribution of pasta by an image processing procedure using a digital camera.Citation11) The method is based on the fact that the pasta is brighter in color at higher levels of moisture content and allowed for the precise quantification of low moisture content portions of the pasta with a high spatial resolution.
A change in the amount of water rehydrated relative to cooking time, that is, the relationship between the rehydration time and an overall moisture content of pasta, is known to be expressed via a hyperbolic curveCitation12) for any type of pasta,Citation13,14) at any rehydration temperatureCitation15) and sodium chloride concentration.Citation16) Although the overall moisture content is lower at lower rehydration temperaturesCitation15) and higher sodium chloride concentrations,Citation16) the effects of the rehydration temperature and sodium chloride concentration on the moisture distribution and texture of rehydrated pasta remain unclear.
In this context, pasta was rehydrated to the al dente state using various temperatures and sodium chloride concentrations. The moisture distribution and the stress–strain curve were examined to reveal the major factors governing the texture of rehydrated pasta. Although the rehydration of pasta at low temperatures or high sodium chloride concentrations is unrealistic in cooking, the results obtained under immoderate conditions provide good insight into the relationship between the moisture distribution and texture of pasta.
Materials and methods
Materials
Cylindrical pasta (spaghetti) was supplied by Nisshin Foods Co., Ltd. (Tokyo, Japan). Its initial diameter was about 1.6 mm. The sodium chloride was of an analytical grade (Wako Pure Chemical Industries, Osaka, Japan) and was dissolved in distilled water.
Rehydration
Culture tubes containing about 50 cm3 of distilled water or a sodium chloride solution of concentration 0.1, 1.0, or 2.0 mol/L were equilibrated at temperatures of 60 and 80 °C in an SD thermominder and Personal-11 water bath (Taitec, Saitama, Japan), and at 100 °C in a stainless-steel tray on a digital hot plate (DP-1S, As One, Osaka, Japan). A spaghetti sample cut into an 8-cm-long section was immersed into each culture tube. At a given time, the samples were removed from the culture tubes, immediately blotted to remove any excess solution, and weighed (W1). The samples were then dried in a convection-drying oven (DO-300FA, As One) at 135 °C for 5 h and weighed (W0). The overall moisture content of the sample, X, was calculated using Equation (1).(1)
Moisture distribution measurement
According to the method described in our previous paper,Citation11) the moisture distribution within spaghetti rehydrated to the al dente state, at which an overall moisture content was 1.70 ± 0.05 kg-H2O/kg-d.m., was measured. The method is based on the increase in sample color brightness that occurs with increasing moisture content.
Break test
The rehydrated spaghetti sample, having an overall moisture content of 1.70 ± 0.05 kg-H2O/kg-d.m., was cut with a wedge-shaped plunger (No. 49) at a rate of 0.5 mm/s using a creep meter (RE2–33005S Rheometer II; Yamaden, Tokyo, Japan) equipped with a 20 N load cell, in order to record the cutting strain and stress. The strain was normalized by a diameter of spaghetti. This test was repeated ten times for each sample.
Results and discussion
Effects of temperature on rehydration
Because the method for measuring moisture distribution requires the calibration curve to correlate the color and moisture content, the calibration curve that was prepared for the spaghetti rehydrated at 100 °C in our previous studyCitation11) was used for all spaghetti rehydrated at other temperatures. Fig. shows the moisture distribution in spaghetti rehydrated at 60, 80, and 100 °C to the overall moisture content of 1.70 ± 0.05 kg-H2O/kg-d.m. The moisture content of the spaghetti rehydrated at 80 and 100 °C was lower near center than in the peripheral region, with the moisture distribution exhibiting a concave shape. Conversely, the moisture content of the spaghetti rehydrated at 60 °C exhibited an almost flat distribution across the entire cross section, although it decreased slightly around the peripheral region and exhibited a weakly convex shape. Changes in the color of rehydrated spaghetti are affected by the gelatinization state of the starch, as well as by the moisture content. The gelatinization state of the starch is determined by the rehydration temperature. Moreover, the spaghetti material leaks into the immersion solution during rehydration, resulting in a loss of mass. This loss of spaghetti mass increases with time.Citation15) When the spaghetti was rehydrated at 60 °C, it took longer to reach the overall moisture content of 1.70 ± 0.05 kg-H2O/kg-d.m. than when rehydration was carried out at other temperatures. Therefore, in this treatment, the gelatinization state of the starch and/or increased loss of spaghetti mass during rehydration results in a slightly convex or almost flat moisture distribution. All spaghetti had almost the same overall moisture content, but the difference in the moisture content between the inner and peripheral regions was greater in spaghetti rehydrated at higher temperatures.
Fig. 1. Moisture distributions of spaghetti rehydrated at 60 °C (∙ ∙ ∙), 80 °C (‒ ‒), and 100 °C (―) in distilled water.
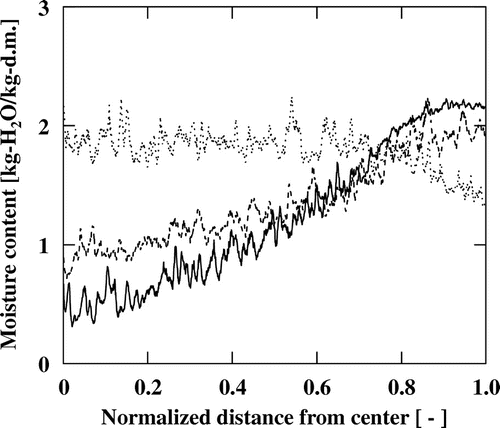
Fig. illustrates the stress–strain curves of spaghetti rehydrated at 60, 80, and 100 °C, with Fig. illustrating the temperature dependence of breaking stress and strain. The spaghetti rehydrated at higher temperatures exhibited smaller stress under low strain and larger stress when under greater strain. The spaghetti rehydrated at higher temperature also exhibited higher breaking stress, but the rehydration temperature did not affect the breaking strain. The stress–strain curve generated for low strain reflects the mechanical properties of the spaghetti surface, while the curve generated for greater strain is governed by the properties of the material near the spaghetti’s core. Because the spaghetti rehydrated at higher temperatures had higher and lower moisture contents near the surface and the center, respectively, it could be cut by lower and greater stresses under lower and greater strains, respectively. The similarity of the breaking strain of all experimental spaghetti with different moisture distributions indicates that the moisture distribution does not affect breaking strain, but instead controls the breaking stress only.
Effects of the salt concentration of the immersion solution
Fig. shows the moisture distributions of the spaghetti rehydrated at 100 °C in 0.1, 1.0, and 2.0 mol/L sodium chloride solutions to the overall moisture content of 1.70 ± 0.05 kg-H2O/kg-d.m. The calibration curve prepared for the spaghetti rehydrated in distilled water was used for the spaghetti rehydrated in the sodium chloride solutions. The moisture distribution of spaghetti rehydrated in distilled waterCitation11) is also shown in the figure. The salt concentration had little effect on the moisture distribution of al dente spaghetti. The overall moisture content at any rehydration time was more heavily suppressed in the same manner by rehydration at lower temperatures and higher salt concentrations.Citation15,16) However, the moisture distribution in the rehydrated al dente spaghetti was nearly unaffected by salt concentration, while the rehydration temperature had a much greater effect. Water migration in the spaghetti is determined by starch gelatinization, diffusion of water, and the relaxation of the gluten matrix.Citation11,17) All of these factors are affected by rehydration temperature. However, the salt concentration alters the gelatinization temperature, but does not directly affect the diffusion coefficient of the water. This difference in the sensitivity of the salt concentration to starch gelatinization and diffusion of water would bring about the difference in the moisture distribution seen in the spaghetti rehydrated in solutions of different salt concentrations.
Fig. 4. Moisture distributions of spaghetti rehydrated at 100 °C in distilled water (―), 0.1 mol/L NaCl solution (‒ ‒), 1.0 mol/L NaCl solution (∙ ∙ ∙), and 2.0 mol/L NaCl solution (‒ ∙).
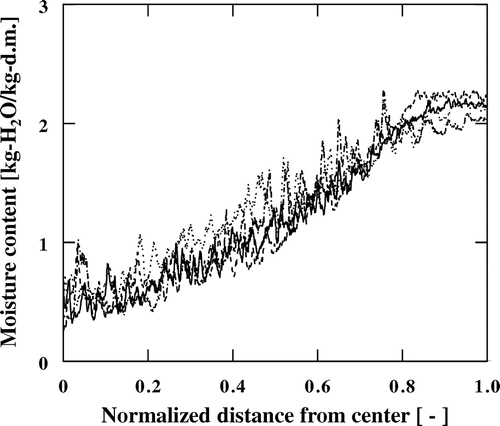
Fig. shows the stress–strain curves of spaghetti rehydrated at 100 °C in the various sodium chloride solutions, and Fig. shows the effect of the salt concentration on its breaking stress and strain. The slope of the curve generated during tests of small strains was larger at higher salt concentrations. This indicates that the spaghetti rehydrated at higher salt concentrations is more elastic. The salt concentration had no significant effect on the breaking stress, while the breaking strain of the spaghetti was smaller in pasta rehydrated at higher salt concentrations. This small strain indicates a reduction in a property equivalent to the stickiness of the spaghetti. No difference in the breaking stress can be ascribed to differences in the moisture distribution among the spaghetti treatments, as there were no significant differences in moisture distribution. Spaghetti possesses a gluten framework in which starch granules are embedded.Citation18,19) It has been reported that salt changes the molecular conformation of the gluten to fibrous network during dough preparation, owing to the addition of water and kneading.Citation20) Ukai et al. reported that both the interactions and distances between the glutenin and gliadin in gluten were altered by the addition of sodium chloride during dough preparation: the distance was shortened.Citation21) Ultracentrifugation measurements revealed the reduction in gluten volume caused by addition of sodium chloride.Citation22) Although all phenomena caused by addition of sodium chloride were observed during dough preparation, it is possible that similar phenomena occur during rehydration in the presence of sodium chloride. Rehydration at higher salt concentrations would contract the gluten network further, lowering the breaking strain. That is, the material properties of spaghetti affect its breaking strain.
Fig. 5. Representative examples of stress–strain curves for spaghetti rehydrated at 100 °C in distilled water (―), 0.1 mol/L NaCl solution (‒ ‒), 1.0 mol/L NaCl solution (∙ ∙ ∙), and 2.0 mol/L NaCl solution (‒ ∙).
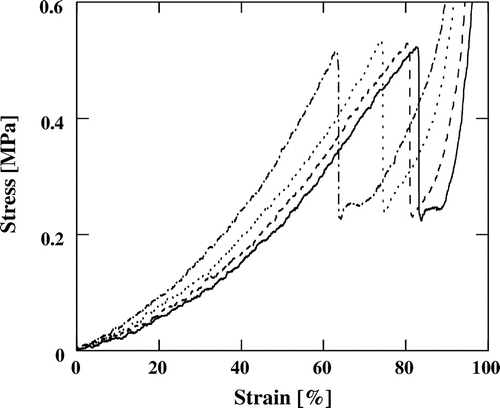
Fig. 6. Relationship between the concentration of the NaCl solution and breaking stress (○) or breaking strain (□).
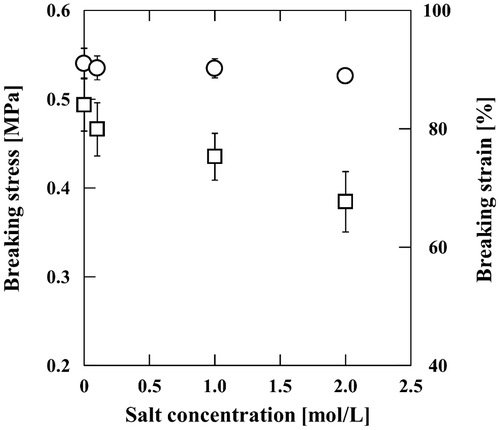
The results obtained in this study suggest that the moisture distribution within spaghetti and its material properties govern its cutting stress and strain, respectively.
Authors Contribution
T.O. and S.A. conceived and designed the experiments, discussed the results, and wrote the manuscript. T.O. performed the experiments and analyzed the data.
Funding
This work was supported by the Grant-in-Aid for JSPS Fellows (T.O.) [grant number 11J02488].
Acknowledgment
This study was carried out during the project study of “The Cereal Science Consortium by the Graduate School of Agriculture, Kyoto University and the Nisshin Seifun Group, Inc.”
Disclosure statement
No potential conflict of interest was reported by the authors.
References
- Yue P, Rayas-Duarte P, Elias E. Effect of drying temperature on physicochemical properties of starch isolated from pasta. Cereal Chem. 1999;76:541–547.10.1094/CCHEM.1999.76.4.541
- Lintas C, D’Appolonia BL. Effect of spaghetti processing on semolina carbohydrates. Cereal Chem. 1973;50:563–570.
- Vansteelandt J, Delcour JA. Physical behavior of durum wheat starch (Triticum durum) during industrial pasta processing. J. Agric. Food Chem. 1998;46:2499–2503.10.1021/jf9710692
- Zweifel C, Conde-Petit B, Escher F. Thermal modifications of starch during high-temperature drying of pasta. Cereal Chem. 2000;77:645–651.10.1094/CCHEM.2000.77.5.645
- Schofield JD, Bottomley RC, Timms MF, et al. The effect of heat on wheat gluten and the involvement of sulphydryl-disulphide interchange reactions. J. Cereal Sci. 1983;1:241–253.10.1016/S0733-5210(83)80012-5
- Zweifel C, Handschin S, Escher F, et al. Influence of high-temperature drying on structural and textural properties of Durum wheat pasta. Cereal Chem. 2003;80:159–167.10.1094/CCHEM.2003.80.2.159
- Aktan B, Khan K. Effect of high-temperature drying of pasta on quality parameters and on solubility, gel electrophoresis, and reversed-phase high-performance liquid chromatography of protein components. Cereal Chem. 1992;69:288–295.
- Lamacchia C, Di Luccia A, Baiano A, et al. Changes in pasta proteins induced by drying cycles and their relationship to cooking behaviour. J. Cereal Sci. 2007;46:58–63.10.1016/j.jcs.2006.12.004
- Malcolmson LJ, Matsuo RR, Balshaw R. Textural optimization of spaghetti using response surface methodology: effects of drying temperature and durum protein level. Cereal Chem. 1993;70:417–423.
- Irie K, Horigane AK, Naito S, et al. Moisture distribution and texture of various types of cooked spaghetti. Cereal Chem. 2004;81:350–355.10.1094/CCHEM.2004.81.3.350
- Ogawa T, Adachi S. Measurement of moisture profiles in pasta during rehydration based on image processing. Food Bioprocess Technol. 2014;7:1465–1471.10.1007/s11947-013-1156-y
- Cunningham SE, McMinn WAM, Magee TRA, et al. Modelling water absorption of pasta during soaking. J. Food Eng. 2007;82:600–607.10.1016/j.jfoodeng.2007.03.018
- Yoshino M, Ogawa T, Adachi S. Properties and water sorption characteristics of spaghetti prepared using various dies. J. Food Sci. 2013;78:E520–E525.10.1111/jfds.2013.78.issue-4
- Aimoto U, Ogawa T, Adachi S. Water sorption kinetics of spaghetti prepared under different drying conditions. Food Sci. Technol. Res. 2013;19:17–22.10.3136/fstr.19.17
- Ogawa T, Kobayashi T, Adachi S. Water sorption kinetics of spaghetti at different temperatures. Food Bioprod. Process. 2011;89:135–141.10.1016/j.fbp.2010.04.004
- Ogawa T, Adachi S. Effect of salts on the water sorption kinetics of dried pasta. Biosci. Biotechnol. Biochem. 2013;77:249–252.10.1271/bbb.120639
- Ogawa T, Hasegawa A, Adachi S. Effects of relaxation of gluten network on rehydration kinetics of pasta. Biosci. Biotechnol. Biochem. 2014;78:1930–1934.10.1080/09168451.2014.925784
- Cunina C, Handschina S, Waltherb P, et al. Structural changes of starch during cooking of durum wheat pasta. LWT – Food Sci. Technol. 1995;28:323–328.10.1016/S0023-6438(95)94552-0
- Dexter JE, Dronzek BL, Matsuo RR. Scanning electron microscopy of cooked spaghetti. Cereal Chem. 1978;55:23–30.
- Tuhumury HCD, Small DM, Day L. The effect of sodium chloride on gluten network formation and rheology. J. Cereal Sci. 2014;60:229–237.10.1016/j.jcs.2014.03.004
- Ukai T, Matsumura Y, Urade R. Disaggregation and reaggregation of gluten proteins by sodium chloride. J. Agric. Food Chem. 2008;56:1122–1130.10.1021/jf0725676
- Larsson H. Effect of pH and sodium chloride on wheat flour dough properties: ultracentrifugation and rheological measurements. Cereal Chem. 2002;79:544–545.10.1094/CCHEM.2002.79.4.544