Abstract
In crustaceans, various physiological events, such as molting, vitellogenesis, and sex differentiation, are regulated by peptide hormones. To understanding the functional sites of these hormones, many structure–activity relationship (SAR) studies have been published. In this review, the author focuses the SAR of crustacean hyperglycemic hormone-family peptides and androgenic gland hormone and describes the detailed results of our and other research groups. The future perspectives will be also discussed.
Graphical abstract
In this review, the author outlines the results of structure–activity relationship studies on crustacean peptide hormones by our and the other research groups.
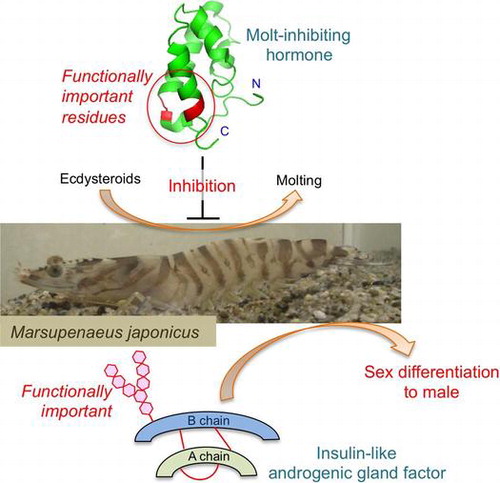
The growth, homeostasis, and development of animals are generally controlled by the endocrine systems. For example, the blood glucose level in mammals is regulated by insulin and glucagon; the former decreases the glucose level in blood stream and the latter shows the opposite activity.Citation1) In crustacean, similar to glucagon, crustacean hyperglycemic hormone (CHH) increases the glucose level in hemolymph.Citation2) CHH is synthesized in and secreted from the X-organ–sinus gland complex located in the eyestalksCitation2) (Fig. ). CHHs have been identified in many crustacean species including decapod,Citation2–4) isopod,Citation5) and stomatopodCitation6) animals, and these are peptide hormones consisting of 72–73 amino acid residues.
Fig. 1. Schematic figure of endocrine organs in the kuruma prawn Marsupenaeus japonicus.
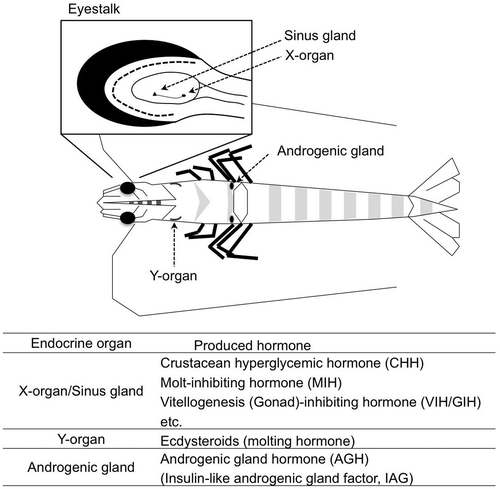
Arthropod animals grow by shedding the exoskeleton, and the molting is triggered by a molting hormone, ecdysteroids.Citation7) In crustacean, the synthesis and the secretion of ecdysteroids by the Y-organs are negatively regulated by a peptide hormone so-called molt-inhibiting hormone (MIH).Citation8) MIH is also secreted from X-organ–sinus gland complex. MIHs have been isolated and characterized from various decapod species and consist of 72–79 amino acid residues.Citation9) Amino acid sequences of MIHs are similar to those of CHHs, and therefore these peptide hormones form the CHH family.Citation2) Until now, CHH-family peptides have been found not only in crustaceans but also in insectsCitation10–12) and a spider.Citation13)
Sex differentiation in crustaceans has been shown to be under hormonal regulation by Charniaux-Cotton.Citation14) This intrinsic factor termed androgenic gland hormone (AGH) has been characterized from a terrestrial isopod Armadillidium vulgare as an insulin-like heterodimeric peptide in 1999.Citation15,16) After that, insulin-like genes have been cloned from several isopodsCitation17,18) and decapod crustaceans,Citation19–22) whereas the functional aspect of these peptides has not yet been analyzed well.
Thus, peptide hormones carry the significant roles for maintaining life not only in vertebrates but also in crustaceans. In this review, the author focuses on the structure and the functions of crustacean peptide hormones and describes the results obtained mainly in our researches on structure–activity relationship studies of CHH-family peptides and of insulin-family peptides.
I. CHH-family peptides
The chemical structure of CHH from the shore crab Carcinus maenas was firstly determined in 1989.Citation23) The CHH consisted of 72 amino acid residues, and no peptide/protein with significant sequence homology was found at that time. On the other hand, a peptide hormone which suppressed the synthesis and the secretion of molting hormone, ecdysteroids, in the Y-organs was characterized from C. maenas in 1991.Citation8) This peptide hormone termed MIH consisted of 78 amino acid residues and shared similar amino acid sequence to CHH. These peptides, therefore, form a peptide family so-called the CHH family.Citation2)
A peptide showing the vitellogenesis-inhibitory function was isolated and characterized from the American lobster Homarus americanus in 1991,Citation24) and this peptide designated as vitellogenesis (gonad)-inhibiting hormone (VIH/GIH) shared the similar amino acid sequence to the CHH-family peptides.Citation24) On the other hand, a CHH-family peptide isolated from the spider crab Libinia emarginata was demonstrated to show the inhibitory effect on the biosynthesis of methyl farnesoate, an insect juvenile hormone-like compound, in the mandibular organs.Citation25) This hormonal peptide was named mandibular organ-inhibiting hormone (MOIH). Thus, VIH and MOIH are now considered to be members of the CHH family.
CHH, MIH, VIH, and MOIH are generally expressed in the X-organ located in the eyestalks and stored in and secreted from the sinus glands.Citation4) Recently, it was reported that some of CHH-family peptide genes were also expressed in non-eyestalk tissues.Citation26–28)
CHH-family peptides could be divided into two groups based on the amino acid sequences; type II peptides have an insertion of Gly residue at the position 12, but type I peptides do not.Citation3,29) To date, CHHs have been characterized in many decapods, one isopod,Citation5) and in one stomatopod species.Citation6) All CHHs identified so far belong to type I and have an amidated C-terminus. On the other hand, the most of MIHs and VIHs belong to the type II, whereas there are some exceptions.
In the kuruma prawn Marsupenaeus japonicus, seven CHH-family peptides were found in the extract of sinus glands as major components, and these peptides are termed Pej-SGP-I to -VII based on the order of peaks in the reversed-phase HPLC chromatogram.Citation29–32) In addition, three minor components belonging to the CHH family were recently characterized,Citation33,34) and total of ten CHH-family peptides have been identified (Fig. ). Since the kuruma prawn is cultivated in the southern part of Japan and it could be purchased from commercial resources all year around, we use M. japonicus as an experimental animal on the structural and functional analyses of CHH-family peptides.
Fig. 2. Amino acid sequence alignment of CHH-family peptides characterized from the kuruma prawn Marsupenaeus japonicus.
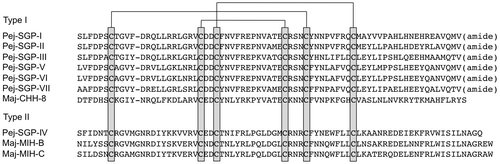
I.i. Crustacean hyperglycemic hormone
In the CHH-family peptides, six Cys residues are completely conserved and form three intrachain disulfide bonds. The disulfide bond arrangement in CHH was firstly determined using C. maenas CHHCitation23) to be Cys1-Cys5, Cys2-Cys4, and Cys3-Cys6, and this arrangement is identical within CHHs analyzed thus far.Citation5,29,30,35–37)
As stated above, the functional CHHs belong to the type I CHH family and have an amidated C-terminus. In M. japonicus, six type I CHH-family peptides, Pej-SGP-I, -II, -III, -V, -VI, and -VII, have been demonstrated to show the hyperglycemic activity in vivo.Citation29,30,32) In our previous study, we demonstrated that the C-terminal amide moiety in CHHs is significant for conferring the hormonal activity in vivo using M. japonicus CHHs, Pej-SGP-I,Citation38) and Pej-SGP-III.Citation39) The significance of C-terminal amide moiety was also demonstrated in CHH of the crayfish Astacus leptodactylus.Citation40)
In several decapod crustaceans, a CHH splicing variant was expressed in non-eyestalk tissues such as pericardial organ, gill, and so on.Citation27,28) The deduced amino acid sequence of the pericardial organ CHH (PO-CHH) is the same as the sinus gland CHH in the N-terminal half, whereas differed in the C-terminal part.Citation27,28) Since the precursors of PO-CHH have no amidation signal at the C-terminus, the mature PO-CHH peptides have a free C-terminus. PO-CHHs showed no or quite weak hyperglycemic activity in vivo,Citation27,41,42) which support the significance of C-terminal amide group on the CHH activity. Similar observation has been reported on the functional analysis of the insect ion transport peptide (ITP) and ITP-like peptide (ITP-L). ITPs have been identified in insects and belong to type I of the CHH family. ITP has an amidated C-terminus and stimulates chloride ion transport in ileum; ITP-L is a splicing variant of ITP having a free C-terminus, and it does not show the ion transport activity.Citation43)
The characteristic of type I of the CHH family is that there is no Gly insertion between the positions 11 and 12, and the all of type II CHH-family peptides show no hyperglycemic activity. Therefore, there is a possibility that the Gly insertion into the CHH at the position 12 abolished the hyperglycemic activity. In order to examine the importance of the absence of Gly insertion, we prepared a CHH mutant having Gly insertion, and its biological activity was assessed.Citation44) As a result, indeed the insertion of Gly residue reduced the hyperglycemic activity in approximately one order of magnitude, but the mutant still showed the activity in vivo.Citation44) Thus, the classification of types in CHH family may be meaningful in structurally but not in functionally.
The mutational analysis of CHH in the N- and C-terminal regions was recently accomplished by other research group.Citation45) The bioassay of substituted mutants revealed that Arg13 and Ile69 were quite important for the hyperglycemic activity in the CHH from the mud crab Scylla olivacea. These two positions are well conserved in CHHs as basic and hydrophobic resides, respectively, and therefore it is likely that these residues are also significant for CHHs in the other crustacean species.
In some CHHs from crabs and crayfishes, the N-terminal Gln residue is converted post-translationally to pyroglutamate,Citation2,23,35,36) and the CHHs of Astacidea have been reported to undergo the stereoisomerization post-translationally at Phe3 residue.Citation36,46,47) The pyroglutaminylation at the N-terminus has been clarified to be not significant for the hyperglycemic activity by means of chemical synthetic method.Citation48) On the other hand, the stereoconversion of l-Phe to d-Phe at the position 3 increases the hyperglycemic activity significantly.Citation48) It may be due to the elongation of half-life in the hemolymph by preventing the enzymatic proteolysis. The mutational analysis of CHH N-terminal region revealed that the substitution of Phe3 to Ala in S. olivacea CHH slightly decreased the biological activity in vivo, and the mutation at the position 2 or 4 did not.Citation45) These results suggest that the N-terminal region of CHH may be important not for the hyperglycemic activity, but for the stability of molecule.
The circular dichroism (CD) spectra of CHHs have been recorded using CHHs from the Mexican crayfish Procambarus bouvieri,Citation49) the kuruma prawn M. japonicus,Citation38,50) and from the mud crab S. olivacea,Citation45) and those gave the negative bands at 208 and 222 nm, demonstrating that CHHs have an α-helical structure. In the type I CHH-family peptides, low molecular weight protein isolated from the venom of the black widow spider Latrodectus mactans tredecimguttatus has been analyzed by CD measurement, and it also showed the spectral pattern typical for α-helical proteins.Citation13) Therefore, it is likely that the type I CHH-family peptides are generally α-helical. Interestingly, it has been demonstrated using M. japonicus CHH that the C-terminally amidated CHH was more helical than the CHH with a free C-terminus.Citation38) Since the amide moiety has a potential to form a hydrogen bond whereas a carboxyl group does not, it may be due in part that the C-terminal amide forms a hydrogen bond to maintain the helical structure. The helical conformation may be important for the hyperglycemic activity. The X-ray crystal structure analysis of CHH is now on progress, and it has been reported that the crystal of CHH precursor peptide was obtained.Citation51) In near future, the tertiary structure will be determined, and more information about the relationship between tertiary structure and the CHH activity might be obtained.
I.ii. Molt-inhibiting hormone
MIHs in the most decapod crustaceans belong to type II of the CHH family,Citation9) whereas there is an exception; MIH from the Mexican crayfish P. bouvieri belongs into type I.Citation52) In addition, some of CHHs have been reported to show the molt-inhibiting activity.Citation29,36,53,54) Therefore, it is likely that the structural characteristics in types of the CHH family are not strongly related to the molt-inhibiting activity. The C-terminal structure of MIHs is varied; the amidated C-terminus was found in some of MIHs,Citation55–57) although the others have a free C-terminus.
Pej-SGP-IV, a member of type II CHH-family peptides from M. japonicus, has been shown to suppress the synthesis and secretion of molting hormone, ecdysteroids, in the Y-organs in vitro, and therefore it is considered to be the M. japonicus MIH.Citation31) Pej-SGP-IV has six Cys residues at the conserved positions among the CHH-family peptides, and these Cys residues form three intrachain disulfide bonds. Its disulfide bond arrangement was the same as that in P. clarkii MIH,Citation58) and also the same as that in the type I CHH-family peptides determined thus far,Citation5,23,29,30,35–37) indicating that the disulfide pairing pattern in CHH-family peptides is identical regardless of the types.
Pej-SGP-V and Pej-SGP-VI, members of type I CHH family, also showed the weak but distinct molt-inhibiting activity in vitro.Citation29,31) Therefore, it seemed to be difficult to predict the biological activity of CHH family peptides from their primary structures. In order to understand the functional site of MIH, we have determined the tertiary structure of Pej-SGP-IV by the nuclear magnetic resonance (NMR) methodCitation59) using the recombinant Pej-SGP-IV expressed in the bacterial expression system.Citation50,60) Pej-SGP-IV consisted of five α-helices and no β-sheet structure (Fig. ),Citation59) which was consistent with that the CD spectral pattern of Pej-SGP-IV was typical for α-helical proteins.Citation50) Nine hydrophobic amino acid residues at the positions of 16, 20, 23, 46, 50, 54, 66, 70, and 73 formed a hydrophobic core inside, and these positions are mostly conserved as hydrophobic residues in CHH-family peptides. Since the CD spectral patterns of CHH-family peptides are similar to each other regardless of types, the type I CHH-family peptides might have the folding similar to that of Pej-SGP-IV.
Fig. 3. The tertiary structures of CHH-family peptides.
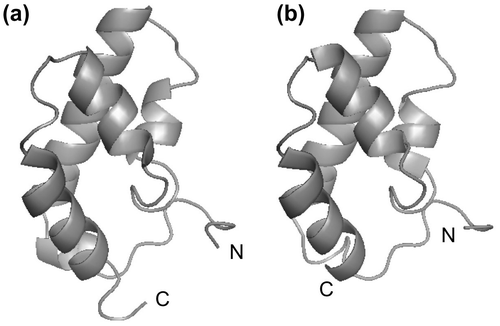
To identify the structural site important for the distinction of CHH and MIH functions, we presumed the tertiary structure of Pej-SGP-III, one of the M. japonicus CHHs, and compared it to the solution structure of Pej-SGP-IV.Citation59) The Gly12 residue of Pej-SGP-IV, characteristic in the type II, located at the N-terminal short α-helix, and this helix was not found in Pej-SGP-III due to the shortened peptide chain by lacking the Gly insertion. These results strongly suggest that the Gly residue at the position 12 in type II peptide is significant for maintaining the helical structure at the N-terminal side.
The C-terminus and the residues at 13 and 69, functionally important residues in CHHs, locate the sterically close position, and therefore this side of molecule is likely to be significant for the function of CHHs. The mutational analysis of Pej-SGP-IV revealed that the residues at the positions of 13, 14, 71, and 72 were quite important for conferring the MIH activity.Citation61) These results are coincident well with those in the mutational analysis of S. olivacea CHH.Citation45) On the other hand, deletion of the Gly12 from Pej-SGP-IV which was expected to lack the N-terminal helical structure did not affect to the hormonal activity, and the deletion mutant showed the MIH activity compatible to the native peptide.Citation61) Thus, the classification of types in the CHH family is not related to the MIH function.
As minor components in the sinus gland extract, MIH-B and MIH-C have been identified in M. japonicus.Citation33,34) A large amount of MIH-B peptide has been prepared by the recombinant protein expression system, and its function was analyzed.Citation33) As a result, MIH-B showed molt-inhibiting activity in vitro weaker than that of Pej-SGP-IV by approximately one order of magnitude. The residues important for MIH activity (Asn13, Arg14, Trp69, Ser71, and Ile72) were completely conserved in MIH-B, and the recombinant MIH-B had the disulfide bond arrangement identical to that in Pej-SGP-IV.Citation33) It is, therefore, difficult to understand the reason for low biological activity of the recombinant MIH-B. It may due to a difference in the local conformation of the receptor recognition site of MIH-B.
I.iii. Vitellogenesis (Gonad)-inhibiting hormone (VIH/GIH)
VIH has been firstly isolated and characterized from the lobster H. americanus.Citation24,62) Up to now, authentic VIHs have been identified from Homarus gammarus,Citation63) Penaeus monodon,Citation64) and A. vulgare.Citation65) In opposite, it has been reported that the CHH-family peptides stimulate the vitellogenin gene expression in some decapod crustaceans,Citation66–68) although the molecular mechanisms on this phenomenon is still unclear. Most CHH-family peptides with the VIH activity are classified into type II,Citation63–65) while only type I peptides showed the VIH activity in M. japonicus.
Seven CHH-family peptides of M. japonicus (Pej-SGP-I to -VII) have been applied to the in vitro bioassay in which suppression of the total protein expression amount in ovaries was analyzed, and it was demonstrated that six of seven peptides, Pej-SGP-I, -II, -III, -V, -VI, and -VII, belonging to type I CHH family showed the biological activity, whereas Pej-SGP-IV, a type II peptide, did not.Citation69) In the other bioassay system using the vitellogenin gene expression levels as the indicator, Pej-SGP-III also suppressed the expression level, although Pej-SGP-IV and MIH-B did not even at high concentration levels.Citation70) Thus, type I CHH-family peptides act as VIH in M. japonicus. Interestingly, H. americanus VIH which is classified in type II also suppressed the vitellogenin gene expression in M. japonicus ovaries at a high dosage level.Citation71) All these results indicate that the classification in types by the structural characteristics never agree with the functional characteristics of CHH family peptides.
II. Crustacean insulin-family peptides
II.i. AGH from isopod
The terrestrial isopod A. vulgare is distributed widely throughout the world and is easily collected, and therefore it had been used as a model animal for AGH studies. The chemical structure of AGH was firstly determined in A. vulgare. AGH is a heterodimeric peptide with an insulin-like sequence, and it has an Asn-linked glycan at the A chain (Fig. (a)).Citation15) The cDNA encoding AGH was also cloned,Citation16) and the recombinant AGH showing the biological activity has been prepared by a baculovirus expression system.Citation72) A. vulgare AGH has eight Cys residues, in which six Cys residues locate the positions conserved in the insulin superfamily peptides, and two additional Cys residues are found in the A chain forming an intrachain disulfide bond. Using the recombinant AGH, the disulfide bond pairing of the conserved six Cys residues was determined to be different from that in the vertebrate insulin.Citation72) From other isopod species, cDNAs encoding putative AGH precursor have been cloned.Citation17,18) These precursors consist of a signal peptide, B chain, C peptide, and A chain, and the eight Cys residues and the putative N-glycosylation site at the A chain are completely conserved among them.
Fig. 4. The primary structures of crustacean insulin-family peptides.
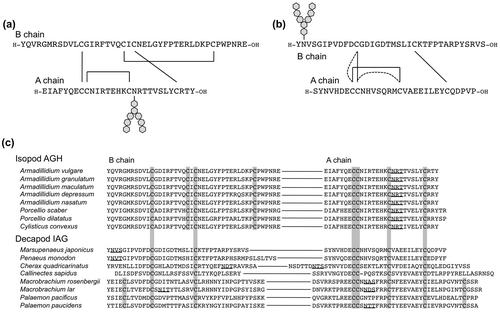
In order to clarify the structure–activity relationship on the carbohydrate structure attached at the A chain, we tried to synthesize A. vulgare AGH carrying a homogenous glycan structure by the expressed protein ligation (EPL) method.Citation73) To use EPL method, N-terminal segment was prepared as the recombinant protein with C-intein tag at the C-terminus by the bacterial expression system. This recombinant protein was converted into the N-terminal segment thioester by the cleavage of C-intein tag with a thiol compound, and then condensed by the native chemical ligation methodCitation74) with the C-terminal segment carrying a carbohydrate chain prepared by the solid-phase peptide synthesis, generating the full-length proAGH with a homogenous glycan structure. After the in vitro folding in a redox buffer and the processing by the protease treatment, the mature form of AGH was obtained.Citation75) However, this semi-synthetic AGH showed no biological activity in vivo regardless of the glycan structure, and it was shown to have the disulfide bond arrangement not with the native type but the same as that of insulin. These results indicated that the correct disulfide bond arrangement is essential for conferring AGH activity in vivo, and that the native folding of AGH is not the most stable form at least in vitro. On the other hand, the synthetic AGH carrying N-acetylglucosamine (GlcNAc) moiety prepared by the total chemical synthesis with regioselective disulfide formation reactions showed the AGH activity in vivo, although non-glycosylated synthetic AGH did not.Citation75) These results indicated that the glycosylation at the A chain is essential for conferring AGH activity. Since the N-glycosylation motif is completely conserved among the isopod AGHs, it is likely that the N-linked glycan attached at the A chain is essential for AGH activity also in the other isopod species.
In the CD spectral analyses of the insulin-family peptides, disulfide isomers of the native peptides generally showed the less α-helical spectral pattern,Citation76,77) and therefore the disulfide isomerization in insulin-family peptides was likely to disrupt the α-helical structure in part. Interestingly, in the comparison with CD spectra between disulfide isomers of AGH, no significant difference was observed,Citation75) indicating that the disulfide isomerization in AGH does not affect the conformation significantly. On the other hand, the glycosylation of A chain affected the CD spectral pattern; the glycosylated AGH showed the more helical pattern than that of non-glycosylated one. These results suggested that the N-linked glycan at the A chain was significant to form the conformation essential for the binding to its receptor.
II.ii. Insulin-like androgenic gland factor (IAG) from decapod
An insulin-like gene was cloned from the red-claw crayfish Cherax quadricarinatus in 2007, and this was the first report on the insulin-like gene from decapod crustaceans.Citation19) This gene was specifically expressed in the male androgenic glands, and therefore it termed insulin-like androgenic gland factor (IAG). Different from isopod AGHs, putative N-glycosylation sites in IAGs are not conserved among them; IAG from the kuruma prawn M. japonicus has one glycosylation site at the position 2 of B chain,Citation22) that of the freshwater prawn Macrobrachium rosenbergii has it at the position 14 of A chain,Citation20) and that of blue crab Callinectes sapidus has no putative glycosylation siteCitation21) (Fig. (c)). In addition, IAGs lack the additional two Cys residues found in the isopod AGHs, and it seems that the decapod IAGs are more related molecules to the vertebrate insulin. The native IAG peptides have not yet been isolated from any decapod crustaceans, and therefore it was difficult to presume the native form of disulfide bond arrangement in IAGs. The function of IAG was remained unclear, although it was presumed to be a decapod AGH due to the amino acid sequence similarity. Actually, it has been reported that the knock down of IAG gene by RNAi induces ferminization of male in C. quadricarinatusCitation78) and M. rosenbergii.Citation20)
In our previous study, in order to obtain the direct evidence for the function of IAG, we synthesized M. japonicus IAG carrying a GlcNAc moiety at the position 2 of B chain with AGH-type or insulin-type disulfides by the solid-phase peptide synthesis and the regioselective disulfide bond formation reactions as shown in Fig. .Citation79) If IAG had a biological activity as the sex determination factor for males, it should suppress the expression levels of female-specific genes, such as vitellogenin gene in ovary. The bioassay system using vitellogenin gene expression levels has been developed as the VIH bioassay system.Citation70) Using this in vitro bioassay, the biological activity of synthetic IAGs was assessed. As a result, the synthetic IAG with insulin-type disulfides showed the biological activity significantly in vitro, whereas that with AGH-type disulfides did not, strongly suggesting that the IAG with insulin-type disulfides is the decapod AGH. In addition, non-glycosylated IAG with the insulin-type disulfide bonds showed no biological activity in vitro, demonstrating that the glycan moiety at the position 2 of B chain was essential for conferring the biological activity.Citation79)
In the CD spectral analysis of the synthetic IAGs, the disulfide isomers showed the spectral patterns different from each other; IAG with the insulin-type disulfide bonds showed more α-helical patterns than that of IAG with the AGH-type disulfide bonds.Citation79) These observations are quite similar to those in the case of vertebrate insulin,Citation76) but not to those in the case of isopod AGH.Citation75) The N-linked glycan at the B chain of IAG also affected the CD spectral pattern,Citation79) suggesting that, similar to the isopod AGH, the N-linked glycan was significant to form the conformation essential for the binding to its receptor.
III. Concluding remarks and perspectives
We focused on the structure–activity relationship on the crustacean peptide hormones, such as CHH-family peptides and insulin-like peptides, and we could identify the functionally important sites on these molecules by the mutational analyses and the chemical synthetic strategy. On the other hand, the receptors for these peptide hormones have not yet been identified, and therefore structural basis of the receptor–ligand bindings cannot be analyzed. To understand the functional site of the peptide hormones in more detail, characterization of the receptors might be important. The receptor of insect ITP has been characterized from the silkworm Bombyx mori,Citation80) and it is expected that the receptors for CHH and MIH will be identified in near future. In crustacean, insulin receptor-like molecule has not yet been identified, and it is difficult to understand the structurally important site for AGH/IAG-receptor binding at present. To identify the AGH/IAG receptors, further experiments might be required.
Acknowledgments
I would like to express my sincere thanks to my supervisors, Prof. Hiromichi Nagasawa of the University of Tokyo, Prof. Saburo Aimoto of Osaka University, Prof. J. Sook Chung of University of Maryland Biotechnology Institute, and Prof. Hironobu Hojo of Osaka University. I am also grateful to my all collaborators, especially Profs. Katsumi Aida, Masaru Tanokura, Yoshiaki Nakahara, Haruyuki Sonobe, Osamu Nureki, Akemi Suzuki, Drs. Shohei Sakuda, Jiro Nakayama, Koji Nagata, Shinji Nagata, Toru Kawakami, Tsuyoshi Ohira, Naoaki Tsutsui, Yuko Nakahara, and Fumiaki Yumoto. Finally, I thank the committee members of The Japan Bioscience, Biotechnology, and Agrochemistry Society for their nomination for the Young Scientists Award in 2015.
Disclosure statement
No potential conflict of interest was reported by the author.
Funding
This work was supported in part by Grants-in-Aid from the Ministry of Education, Culture, Sports, Science and Technology of Japan, Grant-in-Aid from Kato Memorial Foundation; Research and Study Program of Tokai University Educational System General Research Organization.
Notes
This review was written in response to the author’s receipt of the JSBBA Award for Young Scientists in 2015.
References
- Takei Y, Ando H, Tsutsui K, editors. Handbook of hormones-comparative endocrinology for basic and clinical research. Oxford: Academic Press; 2016.
- Keller R. Crustacean neuropeptides: structures, functions and comparative aspects. Experientia. 1992;48:439–448.10.1007/BF01928162
- Lacombe C, Grève P, Martin G. Overview on the sub-grouping of the crustacean hyperglycemic hormone family. Neuropeptides. 1999;33:71–80.10.1054/npep.1999.0016
- Webster SG, Keller R, Dircksen H. The CHH-superfamily of multifunctional peptide hormones controlling crustacean metabolism, osmoregulation, moulting, and reproduction. Gen. Comp. Endocrinol. 2012;175:217–233.10.1016/j.ygcen.2011.11.035
- Martin G, Sorokine O, Van Dorsselaer A. Isolation and molecular characterization of a hyperglycemic neuropeptide from the sinus gland of the terrestrial isopod Armadillidium vulgare (Crustacea). Eur. J. Biochem. 1993;211:601–607.10.1111/ejb.1993.211.issue-3
- Ohira T, Makabe K, Suzuki M, et al. Purification and structural determination of crustacean hyperglycemic hormone (CHH) from mantis shrimp Oratosquilla oratoria. Sci. J. Kanagawa Univ. 2009;20:51–55.
- Skinner DM. Molting and regulation. In: Bliss DE, Mantel LH, editors. The Biology of Crustacea. Vol. 9. New York, NY: Academic Press; 1985. p. 43–146.
- Webster SG. Amino acid sequence of putative molt-inhibiting hormone from the crab Carcinus maenas. Proc. R. Soc. Lond. B. 1991;244:247–252.10.1098/rspb.1991.0078
- Nakatsuji T, Lee CY, Watson RD. Crustacean molt-inhibiting hormone: structure, function, and cellular mode of action. Comp. Biochem. Physiol. A Mol. Integr. Physiol. 2009;152:139–148.10.1016/j.cbpa.2008.10.012
- Audsley N, McIntosh C, Phillips JE. Isolation of a neuropeptide from locust corpus cardiacum which influences ileal transport. J. Exp. Biol. 1992;173:261–274.
- Endo H, Nagasawa H, Watanabe T. Isolation of a cDNA encoding a CHH-family peptide from the silkworm Bombyx mori. Insect Biochem. Mol. Biol. 2000;30:355–361.10.1016/S0965-1748(99)00129-0
- Drexler AL, Harris CC, dela Pena MG, et al. Molecular characterization and cell-specific expression of an ion transport peptide in the tobacco hornworm, Manduca sexta. Cell Tissue Res. 2007;329:391–408.10.1007/s00441-007-0391-9
- Gasparini S, Kiyatkin N, Drevet P, et al. The low molecular weight protein which co-purifies with α-latrotoxin is structurally related to crustacean hyperglycemic hormones. J. Biol. Chem. 1994;269:19803–19809.
- Charniaux-Cotton H. Discovery in an amphipod crustacean (Orchestia gammarella) of an endocrine gland responsible for the differentiation of primary and secondary male sex characteristics. C. R. Acad. Sci. Paris. 1954;239:780–782.
- Martin G, Sorokine O, Moniatte M, et al. The structure of a glycosylated protein hormone responsible for sex determination in the isopod, Armadillidium vulgare. Eur. J. Biochem. 1999;262:727–736.10.1046/j.1432-1327.1999.00442.x
- Okuno A, Hasegawa Y, Ohira T, et al. Characterization and cDNA cloning of androgenic gland hormone of the terrestrial isopod Armadillidium vulgare. Biochem. Biophys. Res. Commun. 1999;264:419–423.10.1006/bbrc.1999.1522
- Ohira T, Hasegawa Y, Tominaga S, et al. Molecular cloning and expression analysis of cDNAs encoding androgenic gland hormone precursors from two porcellionidae species, Porcellio scaber and P. dilatatus. Zool. Sci. 2003;20:75–81.10.2108/zsj.20.75
- Cerveau N, Bouchon D, Bergès T, et al. Molecular evolution of the androgenic hormone in terrestrial isopods. Gene. 2014;540:71–77.10.1016/j.gene.2014.02.024
- Manor R, Weil S, Oren S, et al. Insulin and gender: an insulin-like gene expressed exclusively in the androgenic gland of the male crayfish. Gen. Comp. Endocrinol. 2007;150:326–336.10.1016/j.ygcen.2006.09.006
- Ventura T, Manor R, Aflalo ED, et al. Temporal silencing of an androgenic gland-specific insulin-like gene affecting phenotypical gender differences and spermatogenesis. Endocrinology. 2009;150:1278–1286.10.1210/en.2008-0906
- Chung JS, Manor R, Sagi A. Cloning of an insulin-like androgenic gland factor (IAG) from the blue crab, Callinectes sapidus: implications for eyestalk regulation of IAG expression. Gen. Comp. Endocrinol. 2011;173:4–10.10.1016/j.ygcen.2011.04.017
- Banzai K, Ishizaka N, Asahina K, et al. Molecular cloning of a cDNA encoding insulin-like androgenic gland factor from the kuruma prawn Marsupenaeus japonicus and analysis of its expression. Fish. Sci. 2011;77:329–335.10.1007/s12562-011-0337-8
- Kegel G, Reichwein B, Weese S, et al. Amino acid sequence of the crustacean hyperglycemic hormone (CHH) from the shore crab, Carcinus maenas. FEBS Lett. 1989;255:10–14.10.1016/0014-5793(89)81051-8
- Soyez D, Le Caer JP, Noel PY, et al. Primary structure of two isoforms of the vitellogenesis inhibiting hormone from the lobster Homarus americanus. Neuropeptides. 1991;20:25–32.10.1016/0143-4179(91)90036-I
- Liu L, Laufer H. Isolation and characterization of sinus gland neuropeptides with both mandibular organ inhibiting and hyperglycemic effects from the spider crab Libinia emarginata. Arch. Insect Biochem. Physiol. 1996;32:375–385.10.1002/(ISSN)1520-6327
- Chung JS, Dircksen H, Webster SG. A remarkable, precisely timed release of hyperglycemic hormone from endocrine cells in the gut is associated with ecdysis in the crab Carcinus maenas. Proc. Natl. Acad. Sci. USA. 1999;96:13103–13107.10.1073/pnas.96.23.13103
- Dircksen H, Böcking D, Heyn U, et al. Crustacean hyperglycaemic hormone (CHH)-like peptides and CHH-precursor-related peptides from pericardial organ neurosecretory cells in the shore crab, Carcinus maenas, are putatively spliced and modified products of multiple genes. Biochem. J. 2001;356:159–170.10.1042/bj3560159
- Chen S-H, Lin C-Y, Kuo CM. Cloning of two crustacean hyperglycemic hormone isoforms in freshwater giant prawn (Macrobrachium rosenbergii): evidence of alternative splicing. Marine Biotechnol. 2004;6:83–94.10.1007/s10126-003-0014-8
- Nagasawa H, Yang W-J, Aida K, et al Chemical and biological characterization of neuropeptides in the sinus glands of the kuruma prawn, Penaeus japonicus. In: Shimonishi Y, editor. Peptide Science-Present and Future. Kluwer: Academic Publisher; 1999. p. 453–454.
- Yang W-J, Aida K, Nagasawa H. Amino acid sequences of a hyperglycaemic hormone and its related peptides from the kuruma prawn, Penaeus japonicus. Aquaculture. 1995;135:205–212.10.1016/0044-8486(95)01015-7
- Yang W-J, Aida K, Terauchi A, et al. Amino acid sequence of a peptide with molt-inhibiting activity from the kuruma prawn Penaeus japonicus. Peptides. 1996;17:197–202.10.1016/0196-9781(95)02122-1
- Yang W-J, Aida K, Nagasawa H. Amino acid sequences and activities of multiple hyperglycemic hormones from the kuruma prawn, Penaeus japonicus. Peptides. 1997;18:479–485.10.1016/S0196-9781(96)00332-4
- Ohira T, Katayama H, Tominaga S, et al. Cloning and characterization of a molt-inhibiting hormone-like peptide from the prawn Marsupenaeus japonicus. Peptides. 2005;26:259–268.10.1016/j.peptides.2004.09.003
- Yamano K, Unuma T. Expressed sequence tags from eyestalk of kuruma prawn, Marsupenaeus japonicus. Comp. Biochem. Physiol. A. 2006;143:155–161.10.1016/j.cbpa.2005.11.005
- Huberman A, Aguilar MB, Brew K, et al. Primary structure of the major isomorph of the crustacean hyperglycemic hormone (CHH-I) from the sinus gland of the Mexican crayfish Procambarus bouvieri (Ortmann): interspecies comparison. Peptides. 1993;14:7–16.10.1016/0196-9781(93)90004-Z
- Yasuda A, Yasuda Y, Fujita T, et al. Characterization of crustacean hyperglycemic hormone from the crayfish (Procambarus clarkii): multiplicity of molecular forms by stereoinversion and diverse functions. Gen. Comp. Endocrinol. 1994;95:387–398.10.1006/gcen.1994.1138
- Nagai C, Asazuma H, Nagata S, et al. A convenient method for preparation of biologically active recombinant CHH of the kuruma prawn, Marsupenaeus japonicus, using the bacterial expression system. Peptides. 2009;30:507–517.10.1016/j.peptides.2008.07.017
- Katayama H, Ohira T, Aida K, et al. Significance of a carboxyl-terminal amide moiety in the folding and biological activity of crustacean hyperglycemic hormone. Peptides. 2002;23:1537–1546.10.1016/S0196-9781(02)00094-3
- Ohira T, Katayama H, Aida K, et al. Expression of a recombinant crustacean hyperglycemic hormone of the kuruma prawn Penaeus japonicus in methylotrophic yeast Pichia pastoris. Fish. Sci. 2003;69:95–100.10.1046/j.1444-2906.2003.00592.x
- Mosco A, Edomi P, Guarnaccia C, et al. Functional aspects of cHH C-terminal amidation in crayfish species. Regul. Pept. 2008;147:88–95.10.1016/j.regpep.2008.01.005
- Ohira T, Tsutsui N, Nagasawa H, et al. Preparation of two recombinant crustacean hyperglycemic hormones from the giant freshwater prawn, Macrobrachium rosenbergii, and their hyperglycemic activities. Zool. Sci. 2006;23:383–391.10.2108/zsj.23.383
- Katayama H, Chung JS. The specific binding sites of eyestalk- and pericardial organ-crustacean hyperglycaemic hormones (CHHs) in multiple tissues of the blue crab, Callinectes sapidus. J. Exp. Biol. 2009;212:542–549.10.1242/jeb.022889
- Wang Y-J, Zhao Y, Meredith J, et al. Mutational analysis of the C-terminus in ion transport peptide (ITP) expressed in Drosophila Kc1 cells. Arch. Insect Biochem. Physiol. 2000;45:129–138.10.1002/(ISSN)1520-6327
- Katayama H, Nagasawa H. Effect of a glycine residue insertion into crustacean hyperglycemic hormone on hormonal activity. Zool. Sci. 2004;21:1121–1124.10.2108/zsj.21.1121
- Liu C-J, Huang S-S, Toullec J-Y, et al. Functional assessment of residues in the amino- and carboxyl-termini of crustacean hyperglycemic hormone (CHH) in the mud crab Scylla olivacea using point-mutated peptides. PLoS One. 2015;10:e0134983.10.1371/journal.pone.0134983
- Aguilar MB, Soyez D, Falchetto R, et al. Amino acid sequence of the minor isomorph of the crustacean hyperglycemic hormone (CHH-II) of the Mexican crayfish Procambarus bouvieri (Ortmann): presence of a d-amino acid. Peptides. 1995;16:1375–1383.10.1016/0196-9781(95)02024-1
- Bulau P, Meisen I, Reichwein-Roderburg B, et al. Two genetic variants of the crustacean hyperglycemic hormone (CHH) from the Australian crayfish, Cherax destructor: detection of chiral isoforms due to posttranslational modification. Peptides. 2003;24:1871–1879.10.1016/j.peptides.2003.10.002
- Mosco A, Zlatev V, Guarnaccia C, et al. Novel protocol for the chemical synthesis of crustacean hyperglycemic hormone analogues – an efficient experimental tool for studying their functions. PLoS One. 2012;7:e30052.10.1371/journal.pone.0030052
- Huberman A, Hernández-Arana A, Aguilar MB, et al. Secondary structure of a crustacean neuropeptide hormone family by means of CD. Peptides. 1989;10:1113–1115.10.1016/0196-9781(89)90198-8
- Katayama H, Ohira T, Nagata K, et al. A recombinant molt-inhibiting hormone of the kuruma prawn has a similar secondary structure to a native hormone: determination of disulfide bond arrangement and measurements of circular dichroism spectra. Biosci. Biotechnol. Biochem. 2001;65:1832–1839.10.1271/bbb.65.1832
- Inoue H, Tsutsui N, Nagai C, et al. Crystallization and preliminary X-ray analysis of crustacean hyperglycaemic hormone from the kuruma prawn Marsupenaeus japonicus in its weakly active precursor form Acta Crystallogr. Sect. F Struct. Biol. Cryst. Commun. 2011;67:1586–1589.10.1107/S1744309111040140
- Aguilar MB, Falchetto R, Shabanowitz J, et al. Complete primary structure of the molt-inhibiting hormone (MIH) of the Mexican crayfish Procambarus bouvieri (Ortmann). Peptides. 1996;17:367–374.10.1016/0196-9781(96)00010-1
- Chang ES, Prestwich GD, Bruce MJ. Amino acid sequence of a peptide with both molt-inhibiting and hyperglycemic activities in the lobster, Homarus americanus. Biochem. Biophys. Res. Commun. 1990;171:818–826.10.1016/0006-291X(90)91219-I
- Chung JS, Webster SG. Moult cycle-related changes in biological activity of moult-inhibiting hormone (MIH) and crustacean hyperglycaemic hormone (CHH) in the crab, Carcinus maenas. From target to transcript. Eur. J. Biochem. 2003;270:3280–3288.10.1046/j.1432-1033.2003.03720.x
- Nagasawa H, Yang WJ, Shimizu H, et al. Isolation and amino acid sequence of a molt-inhibiting hormone from the American crayfish, Procambarus clarkii. Biosci. Biotechnol. Biochem. 1996;60:554–556.10.1271/bbb.60.554
- Marco HG, Stoeva S, Voelter W, et al. Characterization and sequence elucidation of a novel peptide with molt-inhibiting activity from the South African spiny lobster, Jasus lalandii. Peptides. 2000;21:1313–1321.10.1016/S0196-9781(00)00273-4
- Bulau P, Okuno A, Thome E, et al. Characterization of a molt-inhibiting hormone (MIH) of the crayfish, Orconectes limosus, by cDNA cloning and mass spectrometric analysis. Peptides. 2005;26:2129–2136.10.1016/j.peptides.2005.03.005
- Kawakami T, Toda C, Akaji K, et al. Synthesis of a molt-inhibiting hormone of the American crayfish Procambarus Clarkii, and determination of the location of its disulfide linkages. J. Biochem. 2000;128:455–461.10.1093/oxfordjournals.jbchem.a022774
- Katayama H, Nagata K, Ohira T, et al. The solution structure of molt-inhibiting hormone from the kuruma prawn Marsupenaeus japonicus. J. Biol. Chem. 2003;278:9620–9623.10.1074/jbc.M212962200
- Ohira T, Nishimura T, Sonobe H, et al. Expression of a recombinant molt-inhibiting hormone of the kuruma prawn Penaeus japonicus in Escherichia coli. Biosci. Biotechnol. Biochem. 1999;63:1576–1581.10.1271/bbb.63.1576
- Katayama H, Ohira T, Nagata S, et al. Structure-activity relationship of crustacean molt-inhibiting hormone from the kuruma prawn Marsupenaeus japonicus. Biochemistry. 2004;43:9629–9635.10.1021/bi049433v
- Soyez D, Van Deijnen JE, Martin M. Isolation and characterization of a vitellogenesis-inhibiting factor from the sinus glands of the lobster Homarus americanus. J. Exp. Biol. 1987;244:479–484.
- Ollivaux C, Vinh J, Soyez D, et al. Crustacean hyperglycemic and vitellogenesis-inhibiting hormones in the lobster Homarus gammarus. FEBS J. 2006;273:2151–2160.10.1111/ejb.2006.273.issue-10
- Treerattrakool S, Panyim S, Chan SM, et al. Molecular characterization of gonad-inhibiting hormone of Penaeus monodon and elucidation of its inhibitory role in vitellogenin expression by RNA interference. FEBS J. 2008;275:970–980.10.1111/ejb.2008.275.issue-5
- Grève P, Sorokine O, Berges T, et al. Isolation and amino acid sequence of a peptide with vitellogenesis inhibiting activity from the terrestrial isopod Armadillidium vulgare (Crustacea). Gen. Comp. Endocrinol. 1999;115:406–414.10.1006/gcen.1999.7330
- Tiu SH, Chan SM. The use of recombinant protein and RNA interference approaches to study the reproductive functions of a gonad-stimulating hormone from the shrimp Metapenaeus ensis. FEBS J. 2007;274:4385–4395.10.1111/j.1742-4658.2007.05968.x
- Zmora N, Trant J, Zohar Y, et al. Molt-inhibiting hormone stimulates vitellogenesis at advanced ovarian developmental stages in the female blue crab, Callinectes sapidus 1: an ovarian stage dependent involvement. Saline Sys. 2009;5:7.10.1186/1746-1448-5-7
- Luo X, Chen T, Zhong M, et al. Differential regulation of hepatopancreatic vitellogenin (VTG) gene expression by two putative molt-inhibiting hormones (MIH1/2) in Pacific white shrimp (Litopenaeus vannamei). Peptides. 2015;68:58–63.10.1016/j.peptides.2014.11.002
- Avarre JC, Khayat M, Michelis R, et al. Inhibition of de novo synthesis of a jelly layer precursor protein by crustacean hyperglycemic hormone family peptides and posttranscriptional regulation by sinus gland extracts in Penaeus semisulcatus ovaries. Gen. Comp. Endocrinol. 2001;124:257–268.10.1006/gcen.2001.7710
- Tsutsui N, Katayama H, Ohira T, et al. The effects of crustacean hyperglycemic hormone-family peptides on vitellogenin gene expression in the kuruma prawn, Marsupenaeus japonicus. Gen. Comp. Endocrinol. 2005;144:232–239.10.1016/j.ygcen.2005.06.001
- Ohira T, Okumura T, Suzuki M, et al. Production and characterization of recombinant vitellogenesis-inhibiting hormone from the American lobster Homarus americanus. Peptides. 2006;27:1251–1258.10.1016/j.peptides.2005.10.019
- Okuno A, Hasegawa Y, Nishiyama M, et al. Preparation of an active recombinant peptide of crustacean androgenic gland hormone. Peptides. 2002;23:567–572.10.1016/S0196-9781(01)00635-0
- Muir TW, Sondhi D, Cole PA. Expressed protein ligation: a general method for protein engineering. Proc. Natl. Acad. Sci. USA. 1998;95:6705–6710.10.1073/pnas.95.12.6705
- Dawson PE, Muir TW, Clark-Lewis I, et al. Synthesis of proteins by native chemical ligation. Science. 1994;266:776–779.10.1126/science.7973629
- Katayama H, Hojo H, Ohira T, et al. Correct disulfide pairing is required for the biological activity of crustacean androgenic gland hormone (AGH): synthetic studies of AGH. Biochemistry. 2010;49:1798–1807.10.1021/bi902100f
- Hua QX, Mayer JP, Jia W, et al. The folding nucleus of the insulin superfamily: a flexible peptide model foreshadows the native state. J. Biol. Chem. 2006;281:28131–28142.10.1074/jbc.M602616200
- Maruyama K, Nagata K, Tanaka M, et al. Synthesis of bombyxin-IV, an insulin superfamily peptide from the silkworm, Bombyx mori, by stepwise and selective formation of three disulfide bridges. J. Protein Chem. 1992;11:1–12.10.1007/BF01025086
- Rosen O, Manor R, Weil S, et al. A sexual shift induced by silencing of a single insulin-like gene in crayfish: ovarian upregulation and testicular degeneration. PLoS One. 2010;5:e15281.10.1371/journal.pone.0015281
- Katayama H, Kubota N, Hojo H, et al. Direct evidence for the function of crustacean insulin-like androgenic gland factor (IAG): total chemical synthesis of IAG. Bioorg. Med. Chem. 2014;22:5783–5789.10.1016/j.bmc.2014.09.031
- Nagai C, Mabashi-Asazuma H, Nagasawa H, et al. Identification and characterization of receptors for ion transport peptide (ITP) and ITP-like (ITPL) in the silkworm Bombyx mori. J. Biol. Chem. 2014;289:32166–32177.10.1074/jbc.M114.590646
- Guex N, Peitsch MC. SWISS-MODEL and the Swiss-Pdb Viewer: an environment for comparative protein modeling. Electrophoresis. 1997;18:2714–2723.10.1002/(ISSN)1522-2683
- DeLano WL. The PyMOL molecular graphics system, version 1.7.4. Schrödinger, LLC. http://www.pymol.org.