Abstract
Cellular prion protein (PrP) copurifies with neuregulin type I-β1 (NRG I-β1), but no interaction has been detected by a general immunoprecipitation study. We speculate that PrP interacts with NRG I-β1. Here, the interaction of PrP with NRG I-β1 was detected by measuring fluorescence resonance energy transfer (FRET) between enhanced blue (EBFP) and enhanced green (EGFP) fluorescent protein-fusion proteins. Full-length PrP interacted with EGFP in addition to NRG I-β1. From this result, we deduced that PrP interacts with EGFP through its unstructured N-terminal domain. We therefore detected FRET between PrP deleting the N-terminal domain and NRG I-β1. In contrast, the C-terminal domain of PrP interacted with NRG I-β1 and the proteins dissociated completely in the presence of sodium chloride. This interaction occurs at the nanomolar level, which is important for the reaction to be functional in organisms. We concluded that PrP interacted with NRG I-β1 through its C-terminal domain.
Graphical abstract
FRET analysis detected that the C-terminal domain of PrP interacted with NRG I-β1.
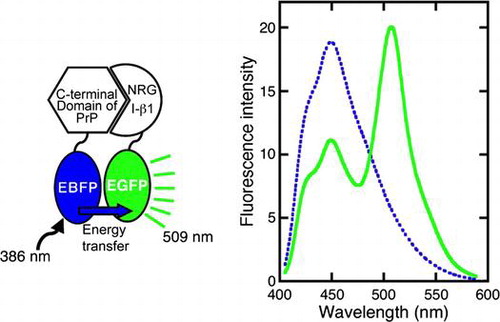
Prion diseases, such as scrapie in sheep and goats, bovine spongiform encephalopathy in cows and Creutzfeldt-Jakob disease in humans, are fatal and transmissible neurodegenerative diseases accompanied by cerebellar ataxia and myoclonus.Citation1–3) In prion diseases, the cellular form of the prion protein (PrP) is structurally transformed into an abnormal isoform with acquired proteinase-resistance.Citation4) The role of the abnormal isoform in prion diseases has been extensively studied. However, the physiological function of PrP is unclear. We believe that in order to elucidate the mechanisms involved in the pathogenesis for prion diseases, it is essential to demonstrate the physiological function of PrP.
PrP is displayed at the outer surface of cells, anchored by a glycosylphosphatidylinositol (GPI). Such GPI-anchored proteins are involved in signaling, immunomodulation, and host–pathogen interaction. Recombinant full-length PrP alone has been produced in an insoluble form in Escherichia coli cells. In addition, we have previously shown that recombinant full-length PrP can be produced in a soluble form by coexpression with neuregulin type I-β1 (NRG I-β1), which is comprised of 234 amino acid residues with the molecular mass of 28.3 kDa and contains an immunoglobulin-like domain and an epidermal growth factor-like domain, in E. coli.Citation5) This soluble coexpression implies some interaction between full-length PrP and NRG I-β1. Furthermore, PrP has been shown to regulate the cleavage of neuregulin 1 (NRG1) in aged PrP-null mouse.Citation6) PrP has also been copurified with acetylcholine receptor-inducing activity (ARIA; also called NRG I-β1) from chick brain,Citation7–9) although no interaction has been detected.Citation8) ARIA is a member of the NRG1 family and neurotrophic factorCitation10–13) that localizes to the neuromuscular junctions (NMJs).Citation14–17) These biochemical and histological evidences suggest that the interaction between these proteins is an important factor determining the physiological function of PrP.
Although PrP copurifies with NRG I-β1, no interaction was detected in a general immunoprecipitation study.Citation8) However, NRG I-β1 has been separated from PrP using a gradient of salts in a heparin column,Citation11–13) which also acts as a cation exchange column. This is because NRG I-β1 elutes at a high concentration of salts (>450 mM NaCl), while PrP is eluted at much lower salt concentrations,Citation11–13) suggesting that the PrP is released from NRG I-β1 at low ion strength. Such a weak interaction would be easily broken in the presence of salts and cannot be detected by a general immunoprecipitation study, which is generally considered an unsuitable tool for detection of weak interactions. We therefore considered that the interaction should be analyzed by fluorescence resonance energy transfer (FRET), which is suitable for the detection of both strong and weak interactions.Citation18)
In the FRET analysis, blue (EBFP) and green (EGFP) enhanced florescent proteins were utilized as the donor and acceptor, respectively. One of the fusion proteins, comprising full-length PrP linked to EBFP (PrP-EBFP) at the C-terminus of PrP, was constructed in our previous study.Citation19) Here, we constructed two fusion proteins, one comprising full-length NRG I-β1 linked to EGFP (NRG-EGFP) and the other was composed of the C-terminal domain (residues 125 to 231) of PrP linked to EBFP (ΔN-PrP-EBFP). As shown in Fig. , using these fusion proteins, we investigated whether PrP interacts with NRG I-β 1 using FRET measurement.
Fig. 1. Schematic model for detection of the interaction PrP with target protein using FRET and constructed fusion proteins.
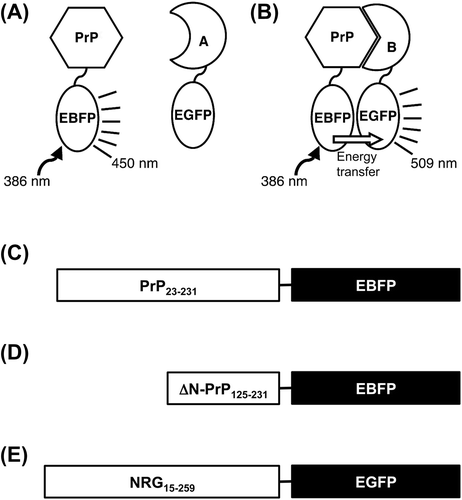
Materials and Methods
Materials
An EBFP-expression vector, pQBI 67, and EGFP expression vector, pQBI T7-GFP, were purchased from Wako Pure Chemical Industries (Osaka, Japan). For the vector constructions, primers were purchased from Hokkaido System Science Co. (Sapporo, Japan). Restriction enzymes were purchased from New England Biolabs Japan Inc. (Tokyo, Japan). E. coli cells, Origami B (DE3), were purchased from Merck (Tokyo, Japan).
Construction of expression vectors
Fusion proteins were constructed as shown in Fig. . A PrP-EBFP expression vector, pQBI 67-PrP, was constructed in accordance with protocols described in our previous study.Citation19) For ΔN-PrP-EBFP expression vector construction, pQBI 67-ΔN-PrP, a PrP cDNA encoding the C-terminal domain comprising the residues 125–231 of PrP (ΔN-PrP) was amplified from pQBI 67-PrP using the following primers: 5′-GCTGCTAGCGTGGCTACATGCTGGGG-3′ and 5′-CGAGCTAGCTAGGCTGGATCTCCCGTCG-3′, in which the Nhe I sites are underlined. The amplified fragments were treated with the restriction enzyme and inserted into pQBI 67.
For NRG-EGFP expression vector construction, pQBI-T7-NRG, an NRG I-β1 cDNA (GeneBank accession no. AY995221) encoding the extracellular region from residues 15 to 259 was amplified from pETDuet-PrP/NRG I-β1Citation5) using the following primers: 5′-CTAGCTAGCAAGAAGGACCGGGGATCCCGC-3′ and 5′-CTAGCTAGCCTTCTGGTAGAGCTCCTCCGC-3′, in which the Nhe I sites are underlined. The amplified fragments were inserted into the Nhe I site of pQBI T7-GFP (pQBI T7-NRG).
Sequence and direction of the inserted cDNAs were confirmed using a genetic analyzer (ABI310, Life Technologies, CA).
Preparation of recombinant proteins
Recombinant proteins were produced in E. coli cells, Origami B (DE3), and separated into soluble and insoluble fractions by centrifugation as described in our previous studies.Citation5,19) PrP-EBFP was purified according to our previously described method.Citation19) EBFP alone and EGFP alone were prepared according to the method as described by Palm et al.Citation20)
For the purification of NRG-EGFP, soluble proteins were precipitated with 30–60% saturated ammonium sulfate and collected by centrifugation. The pellet was resuspended in 20 mM Tris-HCl, pH 7.5, containing 1 mM EDTA and then dialyzed against 20 mM Tris-HCl, pH 7.5, containing 1 mM EDTA and 20 mM NaCl. The dialyzed sample was applied to a heparin-affinity column (HiTrap Heparin HP, GE Healthcare, Buckinghamshire, UK) and then eluted at a gradient of 0.02–2.0 M NaCl in 20 mM Tris-HCl, pH 7.5, containing 1 mM EDTA. Peak fractions containing NRG-EGFP were collected, and then desalted by gel filtration using a sephadex column (NAP-25, GE Healthcare). The sample was applied to an anion-exchange column (Hitrap Q HP, GE Healthcare), and then eluted with a gradient of 0.02–0.3 M NaCl in 20 mM Tris-HCl, pH 7.5, containing 1 mM EDTA. Peak fractions containing NRG-EGFP were collected for further use.
For purification of ΔN-PrP-EBFP, soluble proteins were precipitated with 0–30% saturated ammonium sulfate and collected by centrifugation. Proteins were applied to a heparin column, Butyl-S FF, and then eluted with a gradient of 2.0–0 M ammonium sulfate. Peak fractions containing ΔN-PrP-EBFP were collected and dialyzed in Tris-HCl, pH 9.0. The sample was applied to an anion-exchange column, HiTrap Q HP, and then eluted with a gradient to 0.5 M NaCl. Peak fractions containing ΔN-PrP-EBFP were collected.
The EBFP alone, PrP-EBFP and ΔN-PrP-EBFP concentrations were estimated by UV absorption at 386 nm and at a molecular extinction coefficient of 16,500.Citation20) The concentrations of NRG-EGFP and EGFP alone were estimated by UV absorption at 474 nm and at a molecular extinction coefficient of 26,200.Citation20) The purity was estimated from the intensity of bands detected by the ImageJ software (National Institutes of Health, MD, USA).Citation21)
FRET measurement
EBFP and EGFP were used as the donor and acceptor, respectively. As shown in Fig. , when PrP interacted with NRG, the energy transfer occurred from the excited EBFP to EGFP. The fluorescence spectra were recorded from 400 to 600 nm in 20 mM Tris-HCl, pH 7.5, with or without 250 mM NaCl. Data were recorded using a fluorescence spectrophotometer (Model F-2500, Hitachi High-Technologies Corporation, Tokyo, Japan) after excitation at 386 nm. FRET was measured by combining the acceptor at various concentrations with the donor at the concentration of 50 nM. The energy transfer efficiency, E, was calculated from Equation (Equation1(1) ),
(1)
where IDA and ID represent the fluorescence intensity at 450 nm in the presence and absence of EGFP, respectively.Citation18) The data represent the average of three independent experiments.
Determination of the dissociation constant and intermolecular distance
The energy transfer efficiency was plotted against each acceptor concentration. The plots were fitted by:(2)
where Emax, Kd, and [A] indicate the maximum efficiency, dissociation constant, and acceptor concentration, respectively, using the KaleidaGraph 3.6 software (Synergy Software, PA, USA).
To estimate the distance between chromophores, ΔEmax was calculated by the subtraction of Emax value in the presence of salts as a value for the acceptor bleaching from the Emax value in the absence of salts. The ΔEmax value depends on the inverse sixth power of the dyes.Citation18) The intermolecular distances were calculated from ΔEmax value using the Förster’s equation,(3)
where R and R0 indicate the intermolecular distance between donor and acceptor and Förster’s critical distance for the EGFP-EBFP pair, 34 Å, respectively.Citation22,23) Förster’s critical distance represents the distance at which the trans efficiency is 50%.Citation24)
Results
Fluorescence spectra of PrP-EBFP and NRG-EGFP
PrP-EBFP and NRG-EGFP were prepared with good purity (Fig. (A) and (C)). The purity was estimated to be approximately 99% for PrP-EBFP and 96% for NRG-EGFP from the band intensity determined by ImageJ software. The molecular mass of PrP-EBFP and NRG-EGFP was shown to be approximately 50 kDa and 56 kDa, respectively. These values were close to the theoretical mass, which is the sum of the molecular mass of PrP (23.0 kDa) and EBFP (27.1 kDa) for PrP-EBFP and is the sum of the molecular mass of NRG I-β1 (28.3 kDa) and EGFP (27.1 kDa) for NRG-EGFP.
Fig. 2. Purified fusion proteins and the fluorescence spectrum. (A) Purified PrP-EBFP was analyzed by SDS-PAGE. (B) Fluorescence emission spectrum of PrP-EBFP. The protein was excited at 386 nm. (C) Purified NRG-EGFP was analyzed by SDS-PAGE. (D) Fluorescence emission spectrum of NRG-EGFP. The protein was excited at 474 nm.
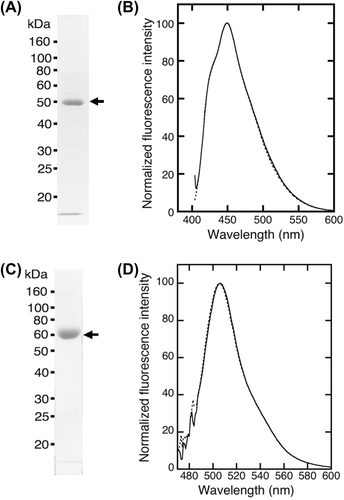
PrP-EBFP and NRG-EGFP were excited at 386 nm and 474 nm, respectively (Fig. (B) and (D)). The fluorescence emission spectra displayed an emission maximum at 450 nm for PrP-EBFP and at 509 nm for NRG-EGFP, which was similar to the spectra of EBFP alone and EGFP alone, respectively. These data show that these fusion proteins are suitable for investigating the interaction between PrP and NRG I-β1 using FRET measurement.
Measurement of FRET between PrP-EBFP and NRG-EGFP
When EBFP was in close proximity to EGFP, the fluorescence resonance energy was transferred from the excited EBFP to EGFP (Fig. ). The FRET was measured between PrP-EBFP and NRG-EGFP (Fig. ). FRET from EBFP to EGFP resulted in decrease of the maximum intensity of EBFP at 450 nm accompanied by an increase in the fluorescence intensity at 509 nm (Fig. ). The maximum intensity of EBFP decreased slightly in the EBFP alone and EGFP alone pair (Fig. (A)) and in the EBFP and NRG-EGFP pair (Fig. (B)), indicating the absence of FRET in these pairs. The results indicate that EBFP alone does not interact with EGFP alone and NRG I-β1. In the PrP-EBFP and EGFP alone pair (Fig. (C)), the peak at 450 nm was dramatically decreased but the intensity at 509 nm did not increase considerably. These results show that although FRET occurred, energy was not transferred from EBFP to EGFP. A previous study indicates that the N-terminal region of PrP, residues 90-125, shows two overlapping absorption bands at ~440 and 480 nm.Citation25) The emission maximum of EBFP overlaps the absorption bands of the N-terminal region of PrP. In addition, the N-terminal domain of PrP is a disordered and hydrophobic region.Citation26) From these evidences, we speculated that the N-terminal domain of PrP interacted with a hydrophobic region of EGFP and that the energy transfer occurred from EBFP to the N-terminal domain of PrP. Furthermore, compared with PrP-EBFP and EGFP alone, the PrP-EBFP and NRG-EGFP pair (Fig. (D)) showed a more dramatic decrease in the peak at 450 nm along with an increase in intensity at 509 nm. This result suggests that PrP interacts with NRG I-β1. However, the FRET measurement would also detect the interaction of PrP with EGFP in addition to that with NRG I-β1.
Fig. 3. FRET between PrP-EBFP and NRG-EGFP.
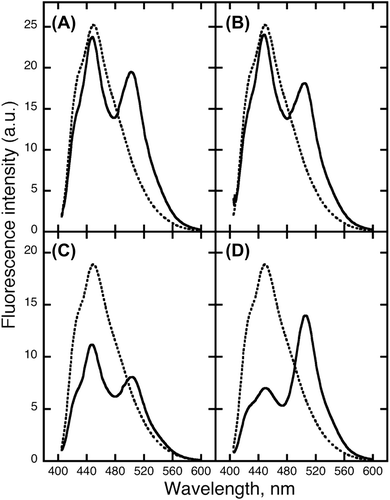
Analysis for the interaction between PrP-EBFP and NRG-EGFP
FRET efficiency (E) depends on the ratio of interacting molecules. To investigate the acceptor concentration-dependence, the E value was calculated using Equation Equation1(1) over various concentrations of the acceptor (Fig. ). In the PrP-EBFP and NRG-EGFP pair, the E value dramatically increased depending on the concentration of the acceptor (Fig. (A), closed circles). In the PrP-EBFP and EGFP alone pair, the efficiency also increased depending on the concentration of the acceptor (Fig. (A), closed circles). The maximum efficiency (Emax) and dissociation constant (Kd) values were calculated by curve-fitting analysis for the plot using Equation Equation2
(2) (Table ). The Emax value for the PrP-EBFP and NRG-EGFP pair (0.68) was higher than that for the PrP-EBFP and EGFP alone pair (0.46). In addition, the Kd value for the PrP-EBFP and NRG-EGFP pair (7.6 nM) was lower than that for the PrP-EBFP and EGFP alone pair (10.5 nM), indicating that PrP interacts with both NRG I-β1 and EGFP.
Fig. 4. Acceptor concentration-dependent change of FRET efficiency in the PrP-EBFP and NRG-EGFP pair.
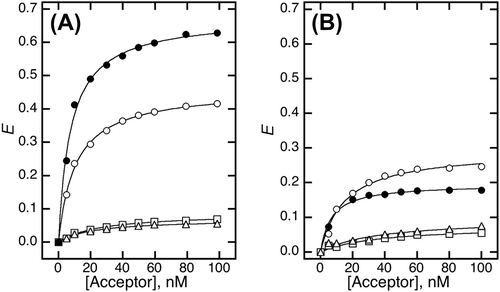
Table 1. FRET efficiency and dissociation constants between PrP-EBFP and NRG-EGFP.
We hypothesize that the interaction between PrP and NRG I-β1 is easily decreased in the presence of sodium chloride at a low concentration. The sodium concentration should be higher than that in extracellular matrix (approximately 150 mM) but lower than that required for separation of PrP from NRG I-β1 in a previous report (<450 mM).Citation11) We therefore analyzed the FRET in the presence of sodium chloride. In the presence of sodium chloride, in both the PrP-EBFP and EGFP alone pair and the PrP-EBFP and NRG-EGFP pair, the E value decreased considerably (Fig. (B), open and closed circles). The low efficiency shows that some proteins interact in the presence of sodium chloride. As shown in Table , the Emax value for the PrP-EBFP and NRG-EGFP pair (0.20) was lower than that for the PrP-EBFP and EGFP alone pair (0.29). Because the interaction between PrP and NRG I-β1 is reduced by addition of sodium chloride, the decrease in the Emax value for the PrP-EBFP and NRG-EGFP pair (0.48) was greater than that for the PrP-EBFP and EGFP alone pair (0.17). These results show that the interactions observed for both pairs involved interactions that were highly sodium chloride-sensitive as well as interactions that had low sensitivity to sodium chloride. The proportion of the low-sensitivity interactions in the PrP-EBFP and EGFP alone pair was greater than that in the PrP-EBFP and NRG-EGFP pair. From the difference in this ratio and the unstructured and highly hydrophobic nature of the N-terminal region of PrP, we deduced that the high-sensitivity interaction is the interaction between PrP and NRG I-β1 and that the low-sensitivity interaction was the interaction between the N-terminal region of PrP and EGFP. We therefore expected that the low-sensitivity interaction can be eliminated by deletion of the N-terminal region of PrP.
Measurement of FRET between ΔN-PrP-EBFP and NRG-EGFP
ΔN-PrP-EBFP was prepared for the measurement of FRET between ΔN-PrP-EBFP and NRG-EGFP with good purity (Fig. (A)). The molecular mass of ΔN-PrP-EBFP was shown to be approximately 39 kDa, similar to the theoretical mass, which is the sum of the molecular masses of ΔN-PrP (12.8 kDa) and EBFP (27.1 kDa). ΔN-PrP-EBFP was purified at high purity (~97%). The fluorescence emission spectra of ΔN-PrP-EBFP excited at 386 nm displayed an emission maximum at 450 nm, similar to that of EBFP alone (Fig. (B)). The data show that ΔN-PrP-EBFP is suitable for investigating the interaction between ΔN-PrP and NRG I-β1 using FRET analysis.
Fig. 5. Purified ΔN-PrP-EBFP and fluorescence spectrum. (A) Purified ΔN-PrP-EBFP was analyzed by SDS-PAGE. (B) Fluorescence emission spectrum of ΔN-PrP-EBFP. The protein was excited at 386 nm.
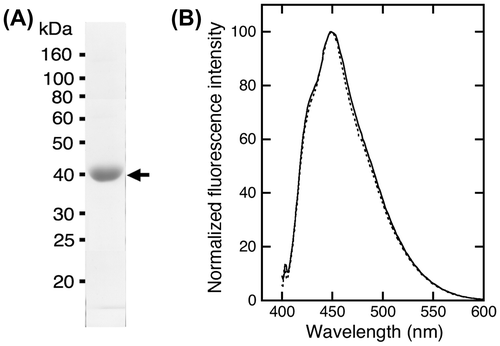
To investigate the interaction between ΔN-PrP and NRG I-β1, the FRET was measured using ΔN-PrP-EBFP and NRG-EGFP (Fig. ). Dramatic FRET was observed in the ΔN-PrP-EBFP and NRG-EGFP pair along with increase in the intensity at 509 nm (Fig. (B)). In contrast, in the ΔN-PrP-EBFP and EGFP alone pair, almost no FRET was detected (Fig. (A)). These results show that the C-terminal domain of PrP interacts with NRG I-β1 and indicate that the N-terminal domain of PrP interacts with EGFP.
Fig. 6. FRET between ΔN-PrP-EBFP and NRG-EGFP. FRET was investigated by combining by ΔN-PrP-EBFP with EGFP alone (A) and PrP-EBFP with NRG-EGFP (B).
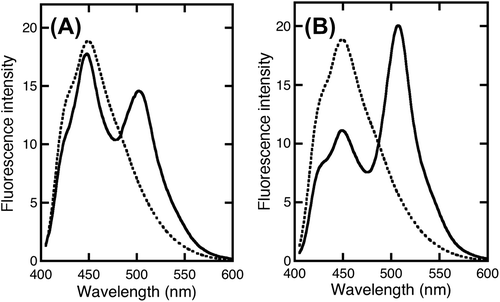
Analysis for interaction between ΔN-PrP-EBFP and NRG-EGFP
The acceptor concentration-dependence was investigated over various concentrations (Fig. ). In the ΔN-PrP-EBFP and NRG-EGFP pair, the E value increased dramatically depending on the concentration of the acceptor (Fig. (D), closed circles). The Emax and Kd values were calculated by curve-fitting analysis for the plot using Equation Equation2(2) (Table ). The Emax value for the ΔN-PrP-EBFP and NRG-EGFP pair (0.58) was clearly higher than that for the ΔN-PrP-EBFP and EGFP alone pair (0.07). The Kd value for the PrP-EBFP and NRG-EGFP pair was estimated to be 44.5 nM. The distance between the chromophores in the ΔN-PrP-EBFP and NRG-EGFP pair was calculated to be 34.2 Å using Equation Equation3
(3) (Table ). These results show that ΔN-PrP interacts with NRG I-β1.
Fig. 7. Acceptor concentration-dependent change of FRET efficiency in the ΔN-PrP-EBFP and NRG-EGFP Pair.
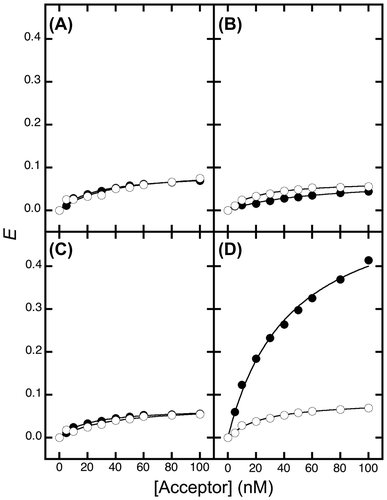
Table 2. FRET efficiency and dissociation constants between ΔN-PrP-EBFP and NRG-EGFP.
The E value decreased largely in the presence of sodium chloride (Fig. (D), open circles). The Emax value for the ΔN-PrP-EBFP and NRG-EGFP pair was calculated to be 0.09. This result indicates that the interaction between ΔN-PrP and NRG I-β1 is decreased in the presence of sodium chloride, which is suggestive of weak interaction between ΔN-PrP and NRG I-β1. We also elucidated that PrP interacts with NRG I-β1 through its C-terminal domain.
Discussion
In this study, our FRET analysis indicated that PrP interacted with NRG I-β1. Previous reports described that NRG I-β1 copurifies with PrP,Citation7–9) although the interaction was not detected in a previous immunoprecipitation study.Citation8) In fact, such a weak interaction cannot be detected by immunoprecipitation. However, using FRET analysis, we could kinetically analyze the interaction. The dissociation constant was determined to be 44.5 nM (Table ). The dissociation constant shows that the interaction likely occurs at the nanomolar level and is actually functional within an organism. Based on these findings, it seems very likely that the PrP-NRG I-β1 interaction plays a significant role in the biological function of PrP.
NRG I-β1 is also known to interact with the ErbB family of proteins, which are tyrosine kinase receptors,Citation27) and to regulate the accumulation of nicotinic acetylcholine receptors at the NMJs.Citation11) NRG I-β1 localizes to the presynapse of NMJs as a transmembrane protein,Citation28) and the extracellular region is released by a protease.Citation12) The released protein interacts with the extracellular domain of ErbB receptor tyrosine kinasesCitation27) to regulate the expression of nicotinic acetylcholine receptor subunits,Citation11,28–30) a function associated with neuroprotection.Citation31) Both PrP and ErbB family members localize to the postsynapse of NMJs. In addition, an electrophysiological study previously showed that PrP is associated with acetylcholine release at the NMJs.Citation32) A previous animal experiment also reported that PrP regulated the cleavage of NRG1.Citation6) These experimental evidences imply that the physiological function of PrP involves signal transduction at the NMJ. The weak interaction detected here would be important for the physiological function of PrP.
PrP is a GPI-anchored protein and is highly fluid on the cell membrane. Some GPI-anchored proteins such as a glia cell line-derived neurotrophic factor receptor α (GFRα) family, GFR α can work as a coreceptor for the activation of Ret kinase.Citation33) The similarity between PrP and GFRα shows the possibility that PrP also functions as a coreceptor. Taken together, the evidence presented here suggests that NRG I-β1 might be a physiological candidate for the ligand of PrP. This seems consistent with a recent report that PrP regulates the cleavage of NRG1 in aged PrP null mice.Citation6) However, considering the localization of these proteins, it is unlikely that PrP directly regulates the cleavage of NRG1. That might be possible only if PrP behaves as a coreceptor. The weak but significant interaction between PrP and NRG I-β1 noted here provides new insights into the physiological function of PrP. In addition, these findings could be used to explain some unexplained findings in PrP-transgenic animal experiments on neurodegenerative diseases. PrP-null mice develop and behave normally,Citation34–36) but at about 70 weeks of age, show progressive symptoms of ataxia.Citation37) The interaction of NRG I-β1 with the native tyrosine kinase receptor is involved in the synthesis and concentration of neurotransmitter receptor in the post synaptic muscle cells of motor neurons.Citation10) PrP functions as a coreceptor and increases the efficiency of the interaction between NRG I-β1 and ErbB receptor. PrP dysfunction would decrease the efficiency of the interaction of NRG I-β1 with ErbB receptor, leading to ataxia. In young mice, NRG I-β1 is fully released to interact with ErbB receptor, but its release is decreased in aged mice. However, because PrP aids the interaction between NRG I-β1 and ErbB receptor, aged normal mice have normal motor functions. NRG1 processing decreases in aged PrP-null mice, but not in normal young mice.Citation6) In addition, PrP knockout mice expressing amino-terminal truncated PrP, which lacks residues 32-121, caused severe ataxia.Citation38) In our study, PrP interacted with NRG I-β1 at the C-terminal domain. The N-terminal-truncated PrP might not be able to pass NRG I-β1 to ErbB receptor in order to exert its inhibitor activity. Recently, the disruption of the NRG1-ErbB pathway was shown to be involved in the pathogenesis of amyotrophic lateral sclerosis (ALS) in which the degeneration of motor neurons leads to progressive weakness and wasting of the limb, bulbar, and respiratory muscles.Citation39,40) The symptoms in ALS resemble those in prion diseases. We suppose that weak interaction of PrP with NRG I-β plays an important role in the preservation of motor neurons. Impairment of the weak PrP-NRG I-β interaction would induce the progressive symptoms of ataxia in prion disease.
Author contributions
Yasuhiro Arii and Shin-Ichi Fukuoka conceived and designed the experiments. Yasuhiro Arii and Hidenori Yamaguchi performed the experiments. Yasuhiro Arii analyzed the data.Yasuhiro Arii and Shin-Ichi Fukuoka contributed reagents/materials/analysis tools. Yasuhiro Arii, Masayuki Yamasaki, and Shin-Ichi Fukuoka wrote the paper.
Acknowledgment
We thank Ms Toshie Kuwahara for her technical assistance.
Disclosure statement
No potential conflict of interest was reported by the authors.
Funding
This work was supported by Grant-in-Aid for Young Scientists (B) from the Ministry of Education, Culture, Sports, Science and Technology of Japan [grant number 17710188].
References
- Prusiner SB. Prion diseases and the BSE crisis. Science. 1997;278:245–251.10.1126/science.278.5336.245
- Prusiner SB. Prions. Proc. Natl. Acad. Sci. USA. 1998;95:13363–13383.10.1073/pnas.95.23.13363
- Horwich AL, Weissman JS. Deadly conformations – protein misfolding in prion disease. Cell. 1997;89:499–510.10.1016/S0092-8674(00)80232-9
- Prusiner SB. Novel proteinaceous infectious particles cause scrapie. Science. 1982;216:136–144.10.1126/science.6801762
- Arii Y, Oshiro S, Wada K, et al. Production of a recombinant full-length prion protein in a soluble form without refolding or detergents. Biosci. Biotechnol. Biochem. 2011;75:1181–1183.10.1271/bbb.100839
- Benvegnù S, Gasperini L, Legname G. Aged PrP null mice show defective processing of neuregulins in the peripheral nervous system. Mol. Cell. Neurosci. 2011;47:28–35.10.1016/j.mcn.2011.02.005
- Usdin TB, Fischbach GD. Purification and characterization of a polypeptide from chick brain that promotes the accumulation of acetylcholine receptors in chick myotubes. J. Cell. Biol. 1986;103:493–507.10.1083/jcb.103.2.493
- Falls DL, Harris DA, Johnson FA, et al. Mr 42,000 ARIA: a protein that may regulate the accumulation of acetylcholine receptors at developing chick neuromuscular junctions. Cold Spring Harbor Symp. Quant. Biol. 1990;55:397–406.10.1101/SQB.1990.055.01.040
- Harris DA, Falls DL, Johnson FA, et al. A prion-like protein from chicken brain copurifies with an acetylcholine receptor-inducing activity. Proc. Natl. Acad. Sci. USA. 1991;88:7664–7668.10.1073/pnas.88.17.7664
- Peles E, Yarden Y. Neu and its ligands: from an oncogene to neural factors. BioEssays. 1993;15:815–824.10.1002/(ISSN)1521-1878
- Falls DL, Rosen KM, Corfas G, et al. ARIA, a protein that stimulates acetylcholine receptor synthesis, is a member of the neu ligand family. Cell. 1993;72:801–813.10.1016/0092-8674(93)90407-H
- Falls DL. Neuregulins: functions, forms, and signaling strategies. Exp. Cell Res. 2003;284:14–30.10.1016/S0014-4827(02)00102-7
- Fischbach GD, Rosen KM. ARIA: a neuromuscular junction neuregulin. Ann. Rev. Neurosci. 1995;20:429–458.
- Chu GC, Moscoso LM, Sliwkowski MX, et al. Regulation of the acetylcholine receptor ϵ subunit gene by recombinant ARIA: an in vitro model for transynaptic gene regulation. Neuron. 1995;14:329–339.10.1016/0896-6273(95)90289-9
- Moscoso LM, Chu GC, Gautam M, et al. Synapse-Associated Expression of an Acetylcholine Receptor-Inducing Protein, ARIA/Heregulin, and Its Putative Receptors, ErbB2 and ErbB3, in Developing Mammalian Muscle. Dev. Biol. 1995;172:158–169.10.1006/dbio.1995.0012
- Sandrock AW Jr, Goodearl AD, Yin QW, et al. ARIA is concentrated in nerve terminals at neuromuscular junctions and at other synapses. J. Neurosci. 1995;15:6124–6136.
- Wang JY, Miller SJ, Falls DL. The N-terminal region of neuregulin isoforms determines the accumulation of cell surface and released neuregulin ectodomain. J. Biol. Chem. 2001;276:2841–2851.10.1074/jbc.M005700200
- Selvin PR. Fluorescence resonance energy transfer. Methods Enzymol. 1995;246:300–334.10.1016/0076-6879(95)46015-2
- Arii Y, Yamaguchi H, Fukuoka S-I. Production of a soluble recombinant prion protein fused to blue fluorescent protein without refolding or detergents in Escherichia coli cells. Biosci. Biotechnol. Biochem. 2007;71:2511–2514.10.1271/bbb.70303
- Palm GJ, Zdanov GA, Gaitanaris GA, et al. The structural basis for spectral variations in green fluorescent protein. Nat. Struct. Biol. 1997;4:361–365.10.1038/nsb0597-361
- Abràmoff MD, Magalhães PJ, Ram SJ. Image processing with image J. Biophotonics International. 2004;11:36–42.
- Suzuki Y, Yasunaga T, Ohkura R, et al. Swing of the lever arm of a myosin motor at the isomerization and phosphate-release steps. Nature. 1998;396:380–383.
- Suzuki Y. Detection of the swings of the lever arm of a myosin motor by fluorescence resonance energy transfer of green and blue fluorescent proteins. Methods. 2000;22:355–363.10.1006/meth.2000.1087
- Stryer L. Fluorescence energy transfer as a spectroscopic ruler. Ann. Rev. Biochem. 1978;47:819–846.10.1146/annurev.bi.47.070178.004131
- Jones CE, Klewpatonond M, Abdelraheim SR, et al. Probing copper2+ binding to the prion protein using diamagnetic nickel2+ and 1H NMR: the unstructured N terminus facilitates the coordination of six copper2+ ions at physiological concentrations. J. Mol. Biol. 2005;346:1393–1407.
- Riek R, Hornemann S, Winder G, et al. NMR characterization of the full-length recombinant murine prion protein, mPrP(23-231). FEBS Lett. 1997;413:282–288.10.1016/S0014-5793(97)00920-4
- Yarden Y, Sliwkowski MX. Untangling the ErbB signalling network. Nat. Rev. Mol. Cell. Biol. 2001;2:127–137.10.1038/35052073
- Jo SA, Zhu X, Marchionni MA, et al. Neuregulins are concentrated at nerve-muscle synapses and activate ACh-receptor gene expression. Nature. 1995;373:158–161.
- Chang Q, Fischbach GD. An acute effect of neuregulin 1β to suppress α7-containing nicotinic acetylcholine receptors in hippocampal interneurons. J. Neurosci. 2006;26:11295–11303.10.1523/JNEUROSCI.1794-06.2006
- Herndon CA, Fromm L. Neuregulin-1 induces acetylcholine receptor transcription in the absence of GABPα phosphorylation. J. Neurosci. Res. 2008;86:982–991.10.1002/(ISSN)1097-4547
- Belluardo N, Mudò G, Blum M, et al. Central nicotinic receptors, neurotrophic factors and neuroprotection. Behav. Brain Res. 2000;113:21–34.10.1016/S0166-4328(00)00197-2
- Re L, Rossini F, Re F, et al. Prion protein potentiates acetylcholine release at the neuromuscular junction. Pharmacol. Res. 2006;53:62–68.10.1016/j.phrs.2005.09.002
- Heuckeroth RO, Enomoto H, Grider JR, et al. Gene targeting reveals a critical role for neurturin in the development and maintenance of enteric, sensory, and parasympathetic neurons. Neuron. 1999;22:253–263.10.1016/S0896-6273(00)81087-9
- Büeler H, Fischer M, Lang Y, et al. Normal development and behaviour of mice lacking the neuronal cell-surface PrP protein. Nature. 1992;356:577–582.10.1038/356577a0
- Manson JC, Clarke AR, Hooper ML, et al. 129/Ola mice carrying a null mutation in PrP that abolishes mRNA production are developmentally normal. Mol. Neurobiol. 1994;8:121–127.10.1007/BF02780662
- Sakaguchi S, Katamine S, Shigematsu K, et al. Accumulation of proteinase K-resistant prion protein (PrP) is restricted by the expression level of normal PrP mice inoculated with a mouse-adapted strain of the Creutzfelt-Jakob disease agent. J. Viol. 1995;69:7586–7592.
- Sakaguchi S, Katamine S, Nishida N, et al. Loss of cerebellar Purkinje cells in aged mice homozygous for a disrupted PrP gene. Nature. 1996;380:528–531.10.1038/380528a0
- Shmerling D, Hegyi I, Fischer M, et al. Expression of amino-terminally truncated PrP in the mouse leading to ataxia and specific cerebellar lesions. Cell. 1998;93:203–214.10.1016/S0092-8674(00)81572-X
- Takahashi Y, Fukuda Y, Yoshimura J, et al. ERBB4 mutations that disrupt the neuregulin-ErbB4 pathway cause amyotrophic lateral sclerosis type 19. Am. J. Hum. Genet. 2013;93:900–905.
- Gallart-Palau X, Tarabal O, Casanovas A, et al. Neuregulin-1 is concetrated in the postsnaptic subsurface cistern of C-bouton inputs to α-motoneurons and altered during motoneuron diseases. FASEB J. 2014;28:3618–3632.10.1096/fj.13-248583