Abstract
We compared the cytotoxic activities of dietary epoxylignans and their stereoisomers and found (−)-verrucosin, which is (7S,7′R,8R,8′R)-7,7′-epoxylignan, to be the most cytotoxic epoxylignan against HeLa cells (IC50 = 6.6 μM). On the other hand, the activity was about a factor of 10 less against HL-60. In this research on the relationship between the structure and cytotoxic activity of (−)-verrucosin 13, the 7-(4-methoxyphenyl)-7′-(3,4-dimethoxyphenyl) derivative 60, for which the activity (IC50 = 2.4 μM) is three times greater than (−)-verrucosin 13, was discovered. The induction of apoptosis by caspase 3/7 was observed upon treatment with the (−)-verrucosin derivative.
Graphical abstract
3′,4,4′-Trimethoxy-7,7′-epoxylignan showed highest IC50 value (2.4 μM) in this structure–cytotoxic activity relationship experiment of 7,7′-epoxylignan. The IC50 value of natural (−)-verrucosin was 6.6 μM.
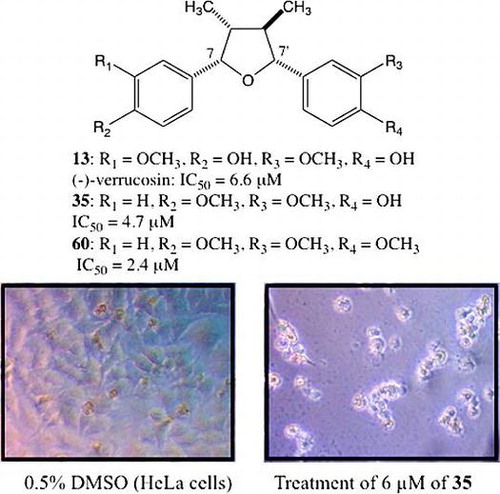
EpoxylignansCitation1) are a well-known family of dietary lignans. Pinoresiol (7,9′;7′,9-diepoxylignan) and lariciresinol (7,9′-epoxylignan) are biosynthesized in vegetables and fruit.Citation2) Verrucosin (7,7′-epoxylignan) and its stereoisomers are contained in spices and nutmeg.Citation3–8) Although the cytotoxic activities of some 7,7′-epoxylignans have been reported,Citation9–11) we have been unable to find any reports on the relationship between the structure and the cytotoxic activity. For the application of lignan compounds to medicine and food supplements, the construction of a library of the relationships between the biological activity and the lignan structure is required. This article describes the effect of the structure of dietary epoxylignan on the cytotoxic activity and the relationship between the stereochemistry of 7,9′- and 7,7′-epoxylignans and its activity. The effects on activity of 7- and 7′-aryl substituents and the 9- and 9′-structure of 7,7′-epoxylignan were also examined by evaluating the activities of (−)-verrucosin derivatives. Since lignans obtained from natural sources are a mixture of (−)- and (+)-forms,Citation12,13) optically pure lignans synthesized using previously reported methods,Citation14–19) but with modifications, were employed for the biological tests in this experiment.
Experimental
Melting points (mp) data are uncorrected. NMR data were acquired by a JEOL JNM-EX400 using TMS as a standard (0 ppm) for 1H, 13C NMR, and trifluoroacetic acid as a standard (−76.5 ppm) for 19F NMR, respectively. MS data were acquired with a JEOL JMS-MS 700 V spectrometer (JEOL Ltd., Tokyo, Japan), and optical rotation values were evaluated with a JASCO P-2100 instrument (JASCO, Tokyo, Japan). Supplementary data associated with this article can be found in Supplementary File.
Cytotoxic assay
Human cervical carcinoma cell line HeLa cells and Human promyelocytic leukemia cell line HL-60 cells were obtained from American Type Culture Collection (Manassas, VA). These cells were cultured in the DMEM medium (Sigma, St. Louis, MO) supplemented with 10% fetal bovine serum (FBS) at 37 °C in a humidified atmosphere of 5% CO2. HeLa cells were inoculated in 96-well culture plate at 2.0 × 104 cells/well suspended in the DMEM medium and HL-60 cells were inoculated in 96-well culture plate at 1.0 × 104 cells/well suspended in RPMI1640 with FBS and various concentrations of different compounds. After cultivation for 48 h, the cell viability was assessed by a WST-8 reduction assay (Dojin Laboratories, Japan). The WST-8 reduction activity of the cells is presented as the ratio of cell activity. Briefly, a WST-8 solution was added to the culture medium at 10% concentration and incubated for 1 h at 37 °C prior to colorimetry at 450 nm. The single cytotoxic assay was performed in triplicate.
Immunofluorescence analysis
HeLa cells were plated and incubated for 24 h before treatment with 0.5% DMSO (negative control), 9.4 μM 4-methoxy derivative, and 0.2 μM staurosporine (positive control) for another 24 h separately. Both adherent and floating cells were harvested with cold PBS and centrifuged at 200 × g for 5 min. Cells were suspended in 10% FBS-DMEM followed by the addition of FAM-FLICA Caspase-3/7 inhibitor (Immunochemistry Technologies) and kept at 37 °C for 1 h protected from light. Then, cells were exposed to 0.5% volume of Hoechst at 37 °C for 5 min and then washed with apoptosis wash buffer twice. Suspension of cells in apoptosis wash buffer was subsequently incubated with 0.5% volume of propidium iodide for 5 min. After washing, cells were analyzed within 4 h under confocal microscope (Fluoview FV10i).
Results and Discussion
Fig. shows the cytotoxic activity of dietary epoxylignans. The activities of (−)- and (+)-pinoresinol (1 and 2) bearing a 7,9′;7′,9-diepoxylignan structure and (−)- and (+)-lariciresinol (3 and 4) bearing a 7,9′-epoxylignan structure were very weak against both HL-60 and HeLa cells. On the other hand, (−)-verrucosin (13), which is a 7,7′-epoxylignan, had the highest activity against HeLa cells. It is noteworthy that the (−)-verrucosin was specifically effective against HeLa cells (IC50 = 6.6 μM), with an activity about 10 times greater than against HL-60 cells.
Fig. 1. Cytotoxic activities of dietary epoxylignans (IC50, μM ± SD, n = 3). Ar = 4-hydroxy-3-methoxyphenyl.
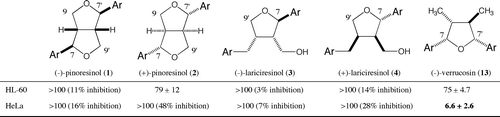
Fig. 2. Cytotoxic activities of stereoisomers of 7,9′-epoxylignans (A) and 7,7′-epoxylignans (B), IC50, μM ± SD, n = 3. Ar = 4-hydroxy-3-methoxyphenyl.
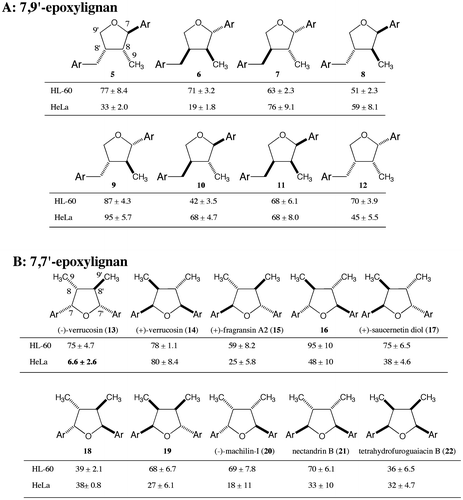
Fig. 5. Cytotoxic activities of 7-(4-methoxyphenyl)-7′-aryl-7,7′-epoxylignans against HeLa cells (n = 3).
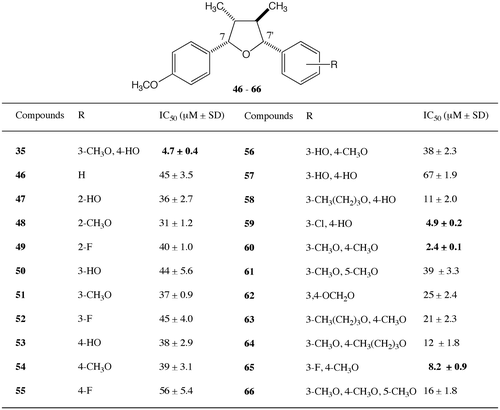
Fig. 7. Introduction of apoptosis by caspase 3/7. (A) Chromatin condensation pattern (blue) in HeLa cells stained with Hoechst. (B) Dead cells (red) detected with propidium iodide (PI) staining. (C) Active caspase 3/7 (green). (D) Merger of B with C.
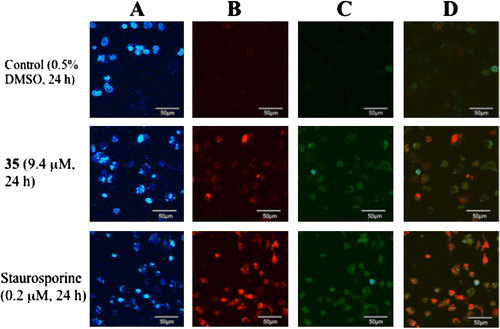
This might be because the activity is higher in a compound without a primary hydroxy group. The effect of a substituent tetrahydrofuran ring should also be examined. In the next step, the cytotoxic activities of all the stereoisomers of 9-dehydroxy lariciresinols 5–12 were examined (Fig. (A)). Although (−)- and (+)-lariciresinol 3 and 4 did not show any activity (Fig. ), all the compounds 5–12 displayed some activity, suggesting that the 9-hydroxy group of 7,9′-epoxylignan moderates the activity. The most potent stereochemistry against HL-60 cells was (7R,8R,8′R)-10, with an IC50 value of 42 μM. Against HeLa cells, (7S,8S,8′R)-6 displayed the most effective activity (IC50 = 19 μM); however, this activity was one-third of that of (−)-verrucosin (13), suggesting the advantages of the 7,7′-epoxylignan structure. The (7S,8S,8′S)-stereoisomer 9 exhibited the weakest activity against both cells.
To obtain a preliminary insight into the relationship between the structure and the activity, the stereochemistry of 7,7′-epoxylignan was examined (Fig. (B)). All stereoisomers of (−)-verrucosin 13–22 were assessed for their cytotoxic activities against HL-60 and HeLa cells. The IC50 values against HL-60 and HeLa cells ranged from 36 to 95 μM and from 6.6 to 80 μM, respectively. Against HL-60 cells, (7R,7′S,8S,8′R)-22 (IC50 = 36 μM) and (7S,7′S,8R,8′R)-18 (IC50 = 39 μM) were more effective than the other stereoisomers, which had IC50 values greater than 59 μM. In the experiment against HeLa cells, (7R,7′S,8S,8′S)-14 showed the weakest activity (IC50 = 80 μM) and the IC50 values of the other stereoisomers were less than 48 μM. In particular, the most potent stereoisomer was (7S,7′R,8R,8′R)-13 (IC50 = 6.6 μM). This was the highest activity from among the 7,7′-epoxylignans bearing the bis(4-hydroxy-3-methoxyphenyl) group and this forced us to select this stereochemistry for further research on the structure–activity relationship.
The Fig. illustrates the cytotoxic activities of the 9- and 9′-derivatives of (−)-(7S,7′R,8R,8′R)-verrucosin (13), which were found to be very weak, with IC50 values of more than 100 μM, suggesting that the hydrophilic group at both the 9- and 9′-positions is detrimental to the activity. The presence of small electron-withdrawing and longer hydrophobic groups at the 9- and 9′-postions decreased the activity, with the fluoro and butyl derivatives 24, 25, 27, and 28, showing 3–11-times less potency than (−)-verrucosin (13). The 9- and 9′-butyl derivatives 25, 28 were twice as potent as the corresponding 9- and 9′-fluoro derivatives 24, 27. This suggests that the hydrophobic group is more favorable than the electron-withdrawing group. The activities of the 9-fluoro and 9′-butyl derivatives 24, 25 were twice as potent as those of the 9′-fluoro and 9′-butyl derivatives 27, 28. A comparison shows the 9-derivatives had higher activities than the 9′-derivatives. Since we had obtained information for the 9- and 9′-derivatives, we decided to prepare 7- and 7′-aromatic derivatives without substituents at the 9- and 9′-positions for the next stage of our research.
The results of the cytotoxic activities of the 7-aromatic derivatives of (−)-verrucosin (13) are shown in Fig. . Examining the mono-hydroxyphenyl derivatives 30, 32, 34 and the mono-methoxyphenyl derivatives 31, 33, 35, the IC50 values of the 3-methoxyphenyl derivative 33, the 4-hydroxyphenyl derivative 34, and the 4-methoxyphenyl derivative 35 are less than 10 μM. The 4-hydroxyphenyl derivative 34 and the 4-methoxyphenyl derivative 35 were more potent. The best position for the hydroxy or the methoxy group for higher activity is the 4-position of the 7-aromatic ring, with the 4-hydroxyphenyl and 4-methoxyphenyl derivatives 34, 35, showing similar activities to natural (−)-verrucosin. The 3-hydroxyphenyl and 3-methoxyphenyl derivatives 32, 33 were 2-fold and 4-fold less potent than the 4-hydroxyphenyl and 4-methoxyphenyl derivatives 34, 35, respectively. The activities of the 2-hydroxyphenyl and 2-methoxyphenyl derivatives 30, 31 were 4-fold and 8-fold less potent than the 4-hydroxyphenyl and 4-methoxyphenyl derivatives 34, 35, respectively. The positions of the hydroxy and methoxy groups affected the activity. To maintain the same level of activity as (−)-verrucosin (13), the 3-methoxy group of 13 is not necessary and the 4-hydroxy group of 13 could be changed to a methoxy group without the need for the 3-methoxy group. From these results, no difference between the hydrophilic and hydrophobic groups was observed, suggesting that the electron-donor group is important on the 7-aromatic ring. Without a substituent, the 7-phenyl derivative 29 was 3 times less effective than (−)-verrucosin (13); however, the activity was recovered by the introduction of a hydroxy or methoxy group at the 4-position. The effect of a 4-substituent on the activity was examined by employing the derivatives 36–39. The 4-fluorophenyl derivative 36 was 3 times less active than (−)-verrucosin (13), suggesting that the electron-withdrawing group reduces the activity. Even with an electron-donor group at the 4-position, the longer carbon chain group was unfavorable, with the 4-butoxyphenyl derivative 39 having one-third of the activity of (−)-verrucosin (13). A smaller electron-donor substituent at the 4-position was effective, with the activities of the 4-methylphenyl derivative 37 and the 4-ethoxyphenyl derivative 38 being similar to that of (−)-verrucosin (13). (−)-Verrucosin (13) (IC50 = 6.6 μM), which has a 3-methoxy-4-hydroxylphenyl group, was more potent than the 3-hydroxy-4-methoxyphenyl derivative 40 (IC50 = 12 μM). In the case of disubstituted phenyl derivatives, the 3,4-dimethoxyphenyl derivative, (−)-aristolignin (41), was 12 times less potent than 13 and the activity of the 3,4-dihydroxyphenyl derivative 42 was 3 times less than that of 13. Thus, it was found that the 3-methoxy group and the 4-hydroxy group of natural (−)-verrucosin (13) are important for higher activity. It might be suggested that a smaller hydrophilic group on both the 3 and 4 positions is more advantageous than a bulky hydrophobic group on both the 3 and 4 positions. This suggestion is supported by the decrease in activity of the 3-longer alkoxyphenyl derivatives 44 and 45. The IC50 values of the 3-ethoxy-4-hydroxyphenyl derivative 44 and the 3-butoxy-4-hydroxyphenyl derivative 45 were 6 times larger than that of 13, the increase in hydrophobicity making the activity lower. The activity of the 3,5-dihydroxyphenyl derivative 43 was similar to that of the 3,4-dihydroxyphenyl derivative 42. We successfully simplified the aryl on the 7-position to give a 4-hydroxyphenyl or 4-methoxyphenyl group, which showed a similar activity to natural 13. Both the hydrophilic and hydrophobic groups on the 4-position of the 7-aromatic ring of 7,7′-epoxylignan were found to have higher cytotoxic activity.
In our next study, we examined the effect of substituents on the 7′-aromatic ring. We employed a 4-methoxyphenyl group on the 7-aromatic ring (Fig. ) due to its higher activity and easier synthesis. The activities with a phenyl derivative 46, mono-hydroxyphenyl derivatives 47, 50, 53, mono-methoxyphenyl derivatives 48, 51, 54, and mono-fluorophenyl derivatives 49, 52, 55 were all less active than 35 and from 5-fold to 8-fold less potent than the activity of 13. This means that the hydrophobic or hydrophilic nature nor the density of electrons were important factors for the activity and the presence of substituents at both the 3- and 4-positions might be necessary for higher activity. Compared with the compound 35 bearing the 3-methoxy-4-hydroxyphenyl group, the positional isomer 56 was 7-fold less potent, suggesting the positions of the two substituents on the 7′-aromatic ring are also important to achieve higher activity. Since the catechol derivative 57 had lower activity, the higher hydrophilic condition is disadvantageous. These results forced us to prepare the derivatives 58–66, which have 3-alkoxy/halo-4-hydroxyphenyl or 3-alkoxy/halo-4-alkoxyphenyl groups. The activities of the longer alkoxyphenyl derivatives 58, 63, 64, the 3,4-methylenedioxyphenyl derivative 62, and the 3,4,5-trimethoxyphenyl derivative 66 were weaker (IC50 = 11 – 25 μM). On the other hand, higher activities were observed for the 3-halo-4-hydroxy/methoxyphenyl derivatives 59, 65 and the 3,4-dimethoxyphenyl derivative 60 (IC50 = 2 – 8 μM). Of these derivatives, the 3,4-dimethoxyphenyl derivative 60 showed the highest activity (IC50 = 2.4 μM) in this project. It might be concluded that small hydrophobic substituents are favorable at the 3 and 4 positions for higher activity. The addition of a methoxy group to the 5-position of 60 decreased the activity, with the 3,4,5-trimethoxyphenyl derivative 66 being 7 times less active than 60. The 3,5-dimethoxyphenyl derivative 61 was 16-fold less potent, suggesting that the positions of the two methoxy groups at the 3 and 4 positions are important. The density of electrons on the 7′-aromatic ring is not important for higher activity, with the 3-chloro-4-hydroxyphenyl derivative 59 and the 3-fluoro-4-methoxyphenyl derivative 65 keeping the IC50 values to less than 10 μM. However, longer substituents at the 3- and 4-positions do not keep the activity high. The activities of the derivatives 63 and 64 bearing a butoxy group at the 3- or 4-position were 5 or 10-fold less potent.
Finally, the activities of the stereoisomers of 34 and 35 were compared. The compounds 67 and 68 were 20- and 10-fold less effective than 34 and 35, respectively (Fig. ). As shown in the first stages of this experiment, the stereochemistry of 7,7′-epoxylignan affects the activity to a large extent.
To examine the mechanism of the cytotoxic activity, we employed immunofluorescence analysis (Fig. ). Staining by Hoechst revealed a blue chromatin condensation pattern in the HeLa cells, which comprises both healthy and dead cells (A). When treated with the compound 35 (9.4 μM) and staurosporine (positive control), the application of PI (propidium iodide) showed half the cells to be red (dead cells) (B). The concentration of compound 35 was twice the IC50 value (recorded 48 h after treatment) used. The amount of dead cells observed 24 h after treatment with compound 35 was 46%. At the same time, activated caspase 3/7, displayed as green, was also observed in the cells treated with compound 35 and staurosporine (C). The cell death caused by the activated caspase 3/7 was clarified by merging B with C (D). Most of the red-stained cells were found to be stained with green also, demonstrating that the 7,7′-epoxylignans introduced the activated caspase 3/7, resulting in apoptosis. The introduction of apoptosis caused by caspase 9 and 3 has been reported in research on 1,7-seco-2,7′-cyclolignan.Citation20) The lignan structure introduced apoptosis as a result of the activation of caspase.Citation20,21)
Among the epoxylignans, we selected nutmeg lignan, (7S,7′R,8R,8′R)-7,7′-epoxylignan 13, which showed the most specific and highest cytotoxic activity against HeLa cells. An experiment to determine the relationship between the structure and the activity was performed in order to determine the best structures at the 9- and 9′-positions and on the aromatic rings. As for the 9- and 9′-positions, longer alkyl, fluoro, and hydroxy group were disadvantageous. The 4-methoxyphenyl group on the 7-aromatic ring was selected as the best group for higher activity. The 3,4-dimethoxyphenyl group was confirmed as the best group on the 7′-aromatic ring. These results led to compound 60 showing the highest cytotoxic activity (IC50 = 2.4 μM) against HeLa cells in this experiment, which is 3 times higher than that of (−)-verrucosin. In the case of acyclic 1,7-seco-2,7′-cyclolignanCitation22) and butane-type dihydroguaiaretic acid lignan,Citation23) different substituents from epoxylignans were selected for each position to obtain the highest activity. Even though the lignan consists of the same two C6-C3 units, the structural factor determines the activity. The difference in activity might be due to the different action mechanism or the different ease with which access to the action site of each lignan is gained. The relationship between the structure and the cytotoxic activity of the food plant component (−)-verrucosin was clarified, and a high activity for the simplified structure was shown. This discovery may help in the development of new medicines based on the natural 7,7′-epoxylignan structure. In a previous study, we simplified the structure of a butane-type lignan to increase the cytotoxic activity.Citation21) Substituents other than butane-type lignans were selected for the research presented here. Apoptosis caused by caspase 3/7 was also shown.
Author contribution
S. Y. and T. W. designed the research plan with assistance from H. N., K. N., T. S., K. A., and T. K. T. W. performed research and analyzed data. S.Y. assisted synthetic experiment and H. N., K. N., and T. S. assisted assay experiment. H. N. assisted apoptosis induction experiment. T. W., H. N., and S. Y. prepared manuscript with assistance from all authors. All authors revised and approved final manuscript.
Funding
This work was supported by the Marutomo Co. and ADRES (Johoku station) of Ehime University.
Supplemental material
The supplemental material for this paper is available at http://dx.doi.org/10.1080/09168451.2015.1123603.
Tuti4SI.doc
Download MS Word (1.1 MB)Acknowledgment
Part of this study was performed at ADRES (Johoku station) of Ehime University. We are grateful to Marutomo Co. for financial support.
Disclosure statement
No potential conflict of interest was reported by the authors.
References
- Ayres DC, Loike JD. Lignans. Cambridge: Cambridge University Press; 1990.10.1017/CBO9780511983665
- Peñalvo JL, Adlercreutz H, Uehara M, et al. Lignan content of selected foods from Japan. J. Agric. Food Chem. 2008;56:401–409.10.1021/jf072695u
- Hattori M, Hada S, Kawata Y, et al. New 2,5-bis-aryl-3,4-dimethyltetrahydrofuran lignans from the aril of Myristica fragrans. Chem. Pharm. Bull. 1987;35:3315–3322.10.1248/cpb.35.3315
- Nguyen PH, Le TVT, Kang HW, et al. AMP-activated protein kinase (AMPK) activators from Myristica fragrans (nutmeg) and their anti-obesity effect. Bioorg. Med. Chem. Lett. 2010;20:4128–4131.10.1016/j.bmcl.2010.05.067
- Huang H-C, Lin Y-C, Fazary AE, et al. New and bioactive lignans from the fruits of Schisandra sphenanthera. Food Chem. 2011;128:348–357.10.1016/j.foodchem.2011.03.030
- Nguyen PH, Kang HW, Le TVT, et al. Simple process for the decrease of myristicin content from Myristica fragrans (nutmeg) and its activity with amp-activated protein kinase (ampk). J. Food Biochem. 2011;35:1715–1722.10.1111/jfbc.2011.35.issue-6
- Zhang L, Chen H, Tian J, et al. Antioxidant and anti-proliferative activities of five compounds from Schisandra chinensis fruit. Ind. Crops Prod. 2013;50:690–693.10.1016/j.indcrop.2013.08.044
- Hien TT, Ki SH, Yang JW, et al. Nectandrin B suppresses the expression of adhesion molecules in endothelial cells: role of AMP-activated protein kinase activation. Food Chem. Toxicol. 2014;66:286–294.10.1016/j.fct.2014.01.052
- Lee JS, Kim J, Yu YU, et al. Inhibition of phospholipase Cγ1 and cancer cell proliferation by lignans and flavans from Machilus thunbergii. Arch. Pharm. Res. 2004;27:1043–1047.10.1007/BF02975429
- Chen J-J, Chou E-T, Duh CY, et al. New cytotoxic tetrahydrofuran- and dihydrofuran-type lignans from the stem of Beilschmiedia tsangii. Planta Med. 2006;72:351–357.10.1055/s-2005-916220
- Thuong PT, Hung TM, Khoi NM, et al. Cytotoxic and anti-tumor activities of lignans from the seeds of Vietnamese nutmeg Myristica fragrans. Arch. Pharm. Res. 2014;37:399–403.10.1007/s12272-013-0185-4
- Suzuki S, Umezawa T, Shimada M. Stereochemical diversity in lignan biosynthesis of Arctium lappa L. Biosci. Biotechnol. Biochem. 2002;66:1262–1269.10.1271/bbb.66.1262
- Finefield JM, Sherman DH, Kreitman M, et al. Enantiomeric natural products: occurrence and biogenesis. Angew. Chem. Int. Ed. 2012;51:4802–4836.10.1002/anie.v51.20
- Yamauchi S, Ichikawa H, Nishiwaki H, et al. Evaluation of plant growth regulatory activity of furofuran lignan bearing a 7,9′:7′,9-diepoxy structure using optically Pure (+)- and (−)-enantiomers. J. Agric. Food Chem. 2015;63:5224–5228.10.1021/acs.jafc.5b01099
- Yamauchi S, Sugahara T, Matsugi J, et al. Effect of the benzylic structure of lignan on antioxidant activity. Biosci. Biotechnol. Biochem. 2007;71:2283–2290.10.1271/bbb.70275
- Nishiwaki H, Kumamoto M, Shuto Y, et al. Stereoselective syntheses of all stereoisomers of lariciresinol and their plant growth inhibitory activities. J. Agric. Food Chem. 2011;59:13089–13095.10.1021/jf203222w
- Nishiwaki H, Nakayama K, Shuto Y, et al. Synthesis of all stereoisomers of 3,3′-dimethoxy-7,7′-epoxylignane-4,4′-diol and their plant growth inhibitory activity. J. Agric. Food Chem. 2014;62:651–659.10.1021/jf4046396
- Yamauchi S, Okazaki M, Akiyama K, et al. First enantioselective synthesis of (−)- and (+)-virgatusin, tetra-substituted tetrahydrofuran lignan. Org. Biomol. Chem. 2005;3:1670–1675.10.1039/b501151e
- Yamauchi S, Nakayama K, Nishiwaki H, et al. Structure-plant phytotoxic activity relationship of 7,7′-epoxylignanes, (+)- and (−)-verrucosin: simplification on the aromatic ring substituents. Bioorg. Med. Chem. Lett. 2014;24:4798–4803.10.1016/j.bmcl.2014.09.006
- Jin Z, El-Deiry WS. Overview of cell death signaling pathways. Cancer Biol. Ther. 2005;4:139–163.
- Fulda S, Debatin KM. Extrinsic versus intrinsic apoptosis pathways in anticancer chemotherapy. Oncogene. 2006;25:4798–4811.10.1038/sj.onc.1209608
- Wukirsari T, Nishiwaki H, Nishi K, et al. Cytotoxic activity of butane type of 1,7-seco-2,7′-cyclolignanes and apoptosis induction by caspase 9 and 3. Bioorg. Med. Chem. Lett. 2014;24:4231–4235.10.1016/j.bmcl.2014.07.033
- Wukirsari T, Nishiwaki H, Nishi K, et al. Cytotoxic activity of dietary lignan and its derivatives: structure–cytotoxic activity relationship of dihydroguaiaretic acid. J. Agric. Food Chem. 2014;62:5305–5315.10.1021/jf5010572