Abstract
Mitochondrial impairment and the resulting generation of reactive oxygen species (ROS) have been associated with aging and its related pathological conditions. Recently, dietary antioxidants have gained significant attention as potential preventive and therapeutic agents against ROS-generated aging and pathological conditions. We previously demonstrated that food-derived antioxidants prevented intracellular oxidative stress under proteasome inhibition conditions, which was attributed to mitochondrial dysfunction and ROS generation, followed by cell death. Here, we further screened dietary antioxidants for their activity as redox modulators by visualization of the redox state using Redoxfluor, a fluorescent protein redox probe. Direct alleviation of ROS by antioxidants, but not induction of antioxidative enzymes, prevented mitochondria-mediated intracellular oxidation. The effective antioxidants scavenged mitochondrial ROS and suppressed cell death. Our study indicates that redox visualization under mitochondria-mediated oxidative stress is useful for screening potential antioxidants to counteract mitochondrial dysfunction, which has been implicated in aging and the pathogenesis of aging-related diseases.
Graphical abstract
Direct alleviation of mitochondrial impairment-producing ROS by dietary antioxidants maintained intracellular redox state and improved cell viability.
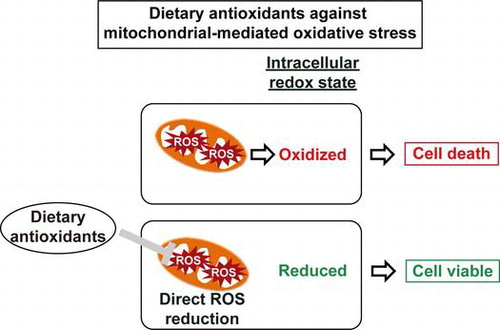
Mitochondria are the power house of cells and are vital organelles for numerous key cellular processes like energy metabolism, calcium homeostasis, apoptosis, and cell signaling.Citation1) Mitochondria are also the major sites for the generation of intracellular reactive oxygen species (ROS). ROS are formed as a result of electrons leaking from the electron transfer chain (ETC) as byproducts during ATP production. The ETC and mitochondrial DNA are susceptible to damage induced by ROS.Citation2,3) With increasing lifetime, mitochondrial activity is impaired due to decreased mitochondrial content and function.Citation4,5) Mitochondrial dysfunction, inducing ROS generation, and oxidative stress, have been implicated in the pathogenesis of age-related neurodegenerative diseases.Citation6,7)
Living tissues including plants are constantly exposed to different exogenous environmental stresses such as heat and light, and endogenous stresses like hydrogen peroxide and transition metals. To protect tissues from such stresses, antioxidants have been developed in these living tissues in the form of flavonoids, polyphenolics, tocopherols, and carotenoids to prevent free radicals, lipid oxidation, and production of toxic forms of transition metals in situ.Citation8–10) We have been consuming these various sources of antioxidants from early ages as fruits, vegetables, herbs, and spices. These bioactive dietary compounds with antioxidant potential play an important role in modulating the amounts of ROS produced in cells due to different stress-causing factors. Recently, a number of studies have focused on the treatment of pathological conditions with naturally occurring antioxidants, especially food-derived dietary antioxidants.Citation11–13)
The ubiquitin proteasome system is the major pathway for eliminating aberrant proteins in cells. Over the course of aging, oxidative modification and decreased expression of proteasomal proteins result in reduced proteasomal activity.Citation14,15) Proteasomal impairment has been linked to the induction of neurodegeneration via oxidative injury.Citation14,16) We previously showed that proteasome inhibition, an aging-model condition in cells, initially causes mitochondrial impairment, and dietary antioxidants capable of scavenging ROS from the impaired mitochondria are effective in maintaining reduced cytosolic conditions and ultimately improving cell viability.Citation17)
In this study, we investigated the activity of dietary antioxidants derived from fruits, vegetables, herbs, and spices against mitochondrial oxidative stress caused by proteasome inhibition using intracellular redox visualization. Among the tested compounds, those possessing direct ROS-scavenging activity showed redox modulating activity, reducing oxidative stress in the cytosol, and alleviating mitochondria ROS generation, thereby increasing cell viability.
Materials and methods
Cell culture
Chinese hamster ovary (CHO) cells were grown in Ham′s F–12 medium supplemented with 10% fetal bovine serum and antibiotics (100 U/mL penicillin and 100 μg/mL streptomycin) under humidified air containing 5% CO2 at 37 °C.
Reagents
MG132, resveratrol, and sesamin were obtained from Wako Pure Chemical (Osaka, Japan). Pyrroloquinoline quinone (PQQ) and its oxidized form, PQQ disodium salt (Bio-PQQ™) were gifts from Mitsubishi Gas Chemical Company, Japan. Lycopene, S-Allyl-L-cysteine, DL-sulforaphane, (±)-α-lipoic acid, and DL-α-tocopherol were purchased from Sigma Aldrich (Tokyo, Japan). MTT (3-(4,5-dimethylthiazol-2-yl)-2,5-diphenyltetrazolium bromide) reagent (Nacalai tesque, Kyoto, Japan) was used for cell viability assays.
FRET analysis using Redoxfluor
A IX70 fluorescence microscope (Olympus, Tokyo, Japan) equipped with a CoolSNAP HQ2 CCD camera (Photometrics, Tucson, AZ) was used for FRET measurement as described previously.Citation17) FRET ratio images were generated from the acquired fluorescent images using the intensity modulated display mode of MetaMorph imaging software (Universal Imaging Corp., Downingtown, PA).
MTT assay
Cell viability was determined by the MTT assay as previously described.Citation17) Briefly, cells were grown in a 96-well microplate incubated with 5 mg/mL MTT for 3 h at 37 °C and 100 μL of solubilization solution (isopropanol with 0.04 mol/L HCl) was added and mixed by pipetting to dissolve precipitated formazans. The absorbance at 570 nm was recorded and cell viability was expressed relative to that of DMSO-treated control cells.
Fluorescence microscopy
A LSM 510 META confocal microscope (Carl Zeiss, Tokyo, Japan) was used for mitochondrial ROS detection under live cell imaging conditions. Once cells reached the desired confluency on glass-based culture dishes, they were incubated with 0.3 μM MitoSOX Red (Invitrogen, Tokyo) for 20 min at 37 °C in the dark and then the medium containing the probe was replaced with new culture medium. Thereafter, MitoSOX Red fluorescence produced by mitochondrial ROS was excited at 405 nm and its emission was detected through a 560-nm long path filter.Citation18,19) ImageJ was used for quantification of acquired images. Eighty cells from minimum of six fields for each treatment were randomly selected for quantification of fluorescence intensity regardless of number of cells in particular field. Fluorescent intensity within a particular size of circle per one cell was quantified and background intensity within the same size of circle was subtracted.
Results
Effect of dietary antioxidants on mitochondria-mediated cytosolic oxidation
In a previous study, we revealed that, under proteasome inhibition conditions, functional mitochondria are crucial to maintain a normal redox state in the cytosol as well as cell viability; in addition, the intracellular oxidation was attributed to impaired mitochondria and their production of ROS.Citation17) To assess the effect of naturally occurring antioxidants against the mitochondrial ROS-mediated oxidation, the redox state in the cytosol was detected using CHO cells stably expressing the redox sensing protein, Redoxfluor.Citation20) Redoxfluor has the C-terminal cysteine-rich domain of the yeast oxidative stress-responding transcription factor Yap1p in tandem, and shows a redox-dependent efficiency of fluorescence resonance energy transfer (FRET) between the N-terminal cerulean and the C-terminal citrine. The reduced form of Redoxfluor indicates a higher FRET ratio and its oxidation decreases the FRET ratio. The FRET ratio under control conditions (treatment with DMSO) was visualized as an orange color in the cytosolic region, whereas treatment with MG132 as a proteasome inhibitor changed the FRET ratio color from orange to green, indicating an oxidized redox state in the cytosol under these conditions (Fig. (A), left upper panel) as previously reported.Citation17) PQQ in its reduced form is an antioxidant that is found in a wide variety of foods like parsley, spinach, and kiwi fruit.Citation21) It plays important roles in the growth and development of plants; however, plants do not have any PQQ biosynthetic pathways.Citation22) Microbes including methylotrophic bacteria, which live on the surface of plants and synthesize PQQ, are believed to be the major source of PQQ for these organisms.Citation23–26) PQQ has been reported to act in a concentration-dependent manner such that concentrations of 50 μM and above act as a pro-oxidant and lower concentrations act as an antioxidant.Citation27–29) In our study, we used 30 μM of each form of PQQ. Simultaneous treatment with MG132 and PQQ resulted in the same cytosolic orange FRET image as observed with control cells (Fig. (A), left middle panel). Interestingly, cells treated with Bio-PQQ™, an oxidized form of PQQ, also exhibited a similar colored FRET image as the cells treated with a reduced form of PQQ. The quantified FRET ratio upon PQQ treatment showed almost the same FRET value as the control, and that of Bio-PQQ™ was slightly lower than the control (Fig. (A), right panel). Both PQQ and Bio-PQQ™ treatments showed higher FRET values compared to resveratrol and sesamin, which showed orange to yellow colored FRET images under conditions of mitochondria-mediated oxidative stress (Fig. (A), left lower panel) as previously described.Citation17)
Fig. 1. FRET analysis of CHO cells treated with antioxidants under proteasome inhibition.
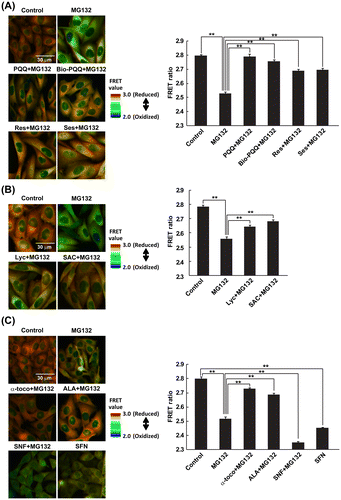
Next, two pigmented dietary antioxidants, bright-red colored lycopene (Lyc)Citation30,31) derived from tomato and beige-colored S-allyl-L-cysteine (SAC) were examined for redox modulating ability under the MG132-treatment condition. SAC is an abundant sulfur-containing antioxidant found in fresh garlic and aged garlic extract.Citation32) Both antioxidants substantially recovered the FRET image from green to orange with statistically significant increases in the FRET values (Fig. (B)).
Furthermore, DL-α-tocopherol (α-toco), a well-known antioxidant and biologically active form of vitamin E,Citation33) (±)-α-lipoic acid (ALA), an antioxidant found in many foods,Citation34) and DL-sulforaphane (SFN), an indirect antioxidant derived from broccoli sprouts Citation35) were used for detection of their redox modulating effect. SFN has been reported to be an inducer of phase II antioxidant genes via the nuclear factor erythroid-2-related factor 2 (Nrf2) pathway.Citation36,37) Among these three natural compounds, α-toco and ALA maintained the normal reduced state in the cytosol under conditions of mitochondrial-ROS-mediated oxidative stress, whereas SFN did not show a redox modulating effect, causing a more oxidized cytosol compared to the MG132-only treated condition. Quantified FRET values for SFN + MG132 and SFN treatments clearly showed the lowest FRET ratios of the conditions examined (Fig. (C), right panel), although SFN treatment significantly induced two phase II genes, glutathione S-transferase A2 and heme oxygenase-1 regardless of the presence of MG132 (Fig. S1). During SFN-treatment conditions, diffusion of Redoxfluor into the nuclear region was observed, whereas Redoxfluor was localized in the cytosol and not in the nucleus under control conditions or in the MG132-treated condition, probably because its larger molecular size (64 kDa) prevented diffusion through the nuclear pore (Fig. S2). This result might suggest that SFN treatment caused damage to either the nucleopore or the nuclear membrane. All the dietary antioxidants except SFN themselves did not affect FRET value of Redoxfluor (Fig. S3). These data suggest that the redox recovery effect of the antioxidants derived from foods under the mitochondria-mediated oxidative stress conditions was brought about by direct antioxidation rather than an oxidative stress response via the Nrf2 pathway.
Preclusive effect of dietary antioxidants on mitochondrial ROS generation causing cytosolic oxidative stress
Next, we examined the dietary antioxidants used in this study for their capacity to prevent the production of mitochondrial ROS, especially superoxide anion generation, which causes cytosolic oxidative stress. Mitochondrial superoxide anion was detected using MitoSOX Red, which preferentially accumulates in mitochondria due to the positive charge and lipophilic nature of triphenylphosphonium linked to hydroethidine,Citation18) which selectively binds to superoxide anions produced in mitochondria.Citation19) Under MG132-treated conditions, there was increased MitoSOX Red fluorescence derived from mitochondrial superoxide anion production (Fig. (A)). PQQ, which has been reported to be a promising antioxidant specifically protecting mitochondria from oxidative stress,Citation27–29) significantly decreased mitochondrial ROS production compared to MG132 treatment; similar results were obtained with resveratrol and sesamin. Bio-PQQ™ also substantially decreased mitochondrial ROS but the effect was slightly less compared to those of PQQ, resveratrol, and sesamin. Lyc and SAC also had similar effects, reversing the effect of proteasome inhibition-induced mitochondrial ROS production. Both of these compounds showed almost equal mitochondrial superoxide anion scavenging effects with statistical significance (Fig. (B)). α-toco, ALA, and SFN were also examined for their effect to reduce mitochondrial ROS production during MG132 treatment. As expected from the results of the cytosolic redox state (Fig. (C)), α-toco and ALA significantly decreased mitochondrial ROS production (Fig. (C)). SFN treatment alone substantially increased ROS production compared to DMSO treatment, whereas SFN treatment in the presence of MG132 did not further aggravate mitochondrial ROS production compared with MG132 treatment alone. To discriminate whether above-mentioned effective dietary antioxidants inhibit mitochondrial ROS production or scavenge ROS generated in mitochondria, mitochondrial ROS was quantified when they were added later after MG132 treatment. As shown in Fig. , all these effective antioxidants even when they were added at 4 h after MG132 treatment prevented increase in mitochondrial ROS at 8 h after the inhibitor treatment. We previously observed mitochondrial oxidation, indicating ROS generation in mitochondria, at 3 h after proteasome inhibitor treatment.Citation17) Therefore, it was suggested that these dietary antioxidants scavenged mitochondrial ROS, not inhibiting the generation of mitochondrial ROS. These data indicate that all the dietary antioxidants that prevented intracellular oxidation also scavenged ROS generated in mitochondria.
Fig. 2. Detection of mitochondrial ROS.
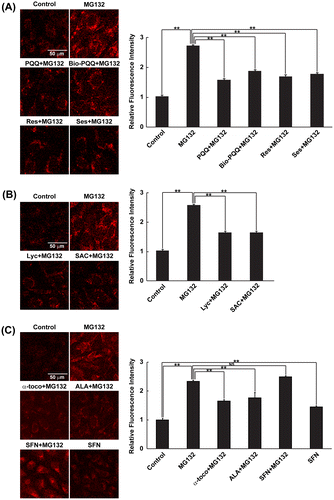
Fig. 3. Effect of dietary antioxidants against ROS generated in mitochondria.
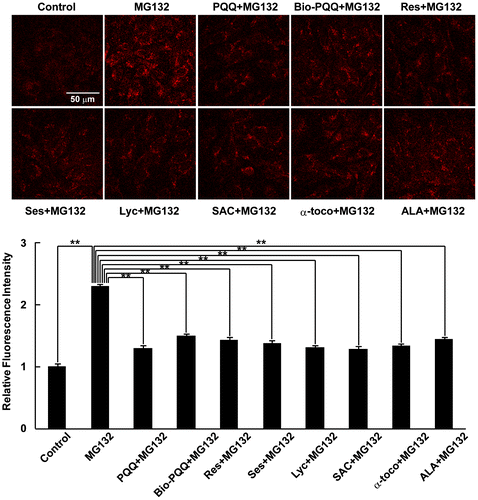
Effect of food-derived antioxidants against cell death
PQQ and Bio-PQQ™ most effectively recovered the cytosolic redox state with prevention of mitochondrial ROS generation under mitochondrial dysfunction conditions caused by proteasome inhibition (Figs. (A) and (A)). We carried out cell viability assays following simultaneous treatment with PQQ or Bio-PQQ™ and MG132. Both PQQ and Bio-PQQ™ improved the viability of cells with statistical significance under conditions of mitochondria-mediated oxidative stress (Fig. (A)). The effect was almost the same as those with resveratrol and sesamin, although the redox recovery effect of PQQ and Bio-PQQ™ was higher compared to those of resveratrol and sesamin (Fig. (A), right panel). These data may suggest the existence of non-mitochondrial-ROS-mediated mechanisms by which proteasome inhibition causes cell death. Next, we evaluated cell viability following treatment with Lyc and SAC under the MG132-treated condition. MTT assays revealed a statistically significant improvement in cell survivability in response to Lyc and SAC treatment compared to treatment with the proteasome inhibitor alone (Fig. (B)). Furthermore, the protective effect of α-toco, ALA, and SFN against cell toxicity caused by MG132 treatment was examined. α-toco and ALA almost equally recovered the cell viability under mitochondria-mediated oxidative stress conditions (Fig. (C)). However, SFN did not improve cell viability during oxidative stress, which is consistent with the results of FRET analysis and mitochondrial ROS measurements. SFN treatment alone also decreased cell viability compared to the control, suggesting that cytotoxicity of SFN itself to cells (Fig. (C)). Taken together, these data demonstrate that all of the dietary antioxidants that prevented mitochondria-mediated cytosolic oxidation also decreased cell death.
Fig. 4. MTT Cell viability assay.
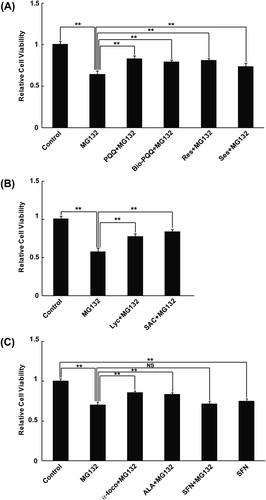
Discussion
We screened various antioxidants that are commonly found in a wide variety of foods, from fruits to vegetables and spices, against mitochondrial-ROS-mediated oxidative stress. All of the compounds used in this study except SFN were effective as redox modulators with the ability to maintain a normal intracellular redox state as visualized by Redoxfluor under mitochondrial oxidative stress conditions (Fig. ). Although SFN induced specific antioxidant genes via the Nrf2 pathway as an indirect antioxidant (Fig. S2), it did not maintain the normal cytosol redox state during oxidative stress (Fig. (C)). SAC has been reported to protect neuronal cells by Nrf2 activation against stroke in mice,Citation38) and SAC is also capable of scavenging ROS in PC12 cells.Citation39) α-toco and Lyc have been reported to be free radical scavengers in primary murine microglia and human lymphocytes.Citation40,41) ALA exerted its antioxidant effect by quenching ROS in primary cultures of neurons derived from embryonic rat forebrain.Citation42) PQQ functions as a scavenger of ROS, especially superoxide, in SH-SY5Y cells and rat forebrain neurons as reported by Hara et al.Citation43) and Zhang and Rosenberg.Citation44) Therefore, it was suggested that direct alleviation of ROS but not antioxidant gene induction in the cytosol is essential for cytosolic redox maintenance during mitochondrial oxidative stress.
Antioxidants, especially dietary antioxidants have attracted great attention for their ability to prevent ROS-mediated cellular damage causing neurodegenerative diseases; however, methods for evaluation of antioxidants are limited to in vitro or in vivo assays, and there has been no reliable assay suitable for high-throughput screening of antioxidants. Various in vitro methods have advantages for evaluating the ability to scavenge specific ROS, but their effects as ROS scavengers are not guaranteed in cells. PQQ is thought to be an excellent antioxidant, but cellular treatment with higher concentrations of PQQ showed pro-oxidant effects.Citation27,29) In many cases, blood concentration of dietary antioxidants (submicromolar order or less)Citation45–47) did not reach to the concentrations (10 or 30 μM) we used in this study, however single 600-mg dose of ALA given to rats produced a peak plasma concentration of 13.8 μM,Citation48) approximately equal to the concentrations in our cellular assay. In vivo assays such as treatment of animals with antioxidants are excellent for evaluating the overall effects on individual animals, but such assays are time consuming and expensive, and the results do not reveal their mechanisms of action. Our cellular-based assay using reversible redox visualization, which is correlated with cell viability as demonstrated in the previousCitation17) and the present study (Figs. and ), is a convenient, reliable, and cost-effective method for screening antioxidants. In addition, by carrying out further analysis, we can evaluate their mechanisms of action in cells. Actually, we could reveal that effective antioxidants under proteasome inhibition scavenged mitochondrial ROS in the previous Citation17) and the present study, suggesting that our cellular-based assay is useful for screening of antioxidants specific to organelle such as mitochondria. Although DCFDA system has often been used as a cellular-based assessment of antioxidants, DCFDA system is an irreversible ROS level indicator and not correlated with cell viability. This system is hard to be used for evaluation of action mechanisms of antioxidants in cells because DCFDA is spread all over cells. Some artifacts of ROS detection by DCFDA system have also been reported.Citation49) Therefore, our cell-based assay will certainly facilitate the identification of new effective antioxidants and elucidate their action mechanisms in the near future.
We previously demonstrated that cytosolic oxidation during inhibition of proteasomal degradation was attributed to mitochondrial dysfunction, and thus, antioxidation in mitochondria could prevent oxidative stress in the cytosol and the resulting cell death.Citation17) All of the effective antioxidants for redox maintenance in the cytosol decreased mitochondrial ROS and also restored cell viability (Figs. ). Our results demonstrated that the screening of antioxidants using redox visualization under mitochondria-ROS-mediated oxidative stress is beneficial for identification of those that are effective in preventing mitochondrial impairment, a major contributor to degenerative diseases and aging.Citation50,51) Our study has also shed light on where in cells antioxidants could be effective against oxidative stress underlying particular pathologies. Dietary antioxidants to nullify site-specific ROS that cause cellular damage will be beneficial for prevention of ROS-related pathogenesis including neurodegenerative diseases.
Author contributions
S. Maharjan, Y. Sakai, and J. Hoseki conceived and designed the experiments. S. Maharjan performed all the experiments and analyzed all the data. S. Maharjan, Y. Sakai, and J. Hoseki wrote the manuscript. All the authors reviewed and approved the final manuscript.
Funding
This work was supported by a Grant-in-Aid for Scientific Research (B) [grant number 26292052] and a Grant-in-Aid for Exploratory Research [grant number 26660061] from JSPS to Y.S., a Grant-in-Aid for Scientific Research (C) [grant number 26440050] from JSPS to J.H., and a scholarship from MEXT to S.M.
Supplemental material
Supplemental material for this article can be accessed at http://dx.doi.org/10.1080/09168451.2015.1123607
Sunita_et_al_Supporting_Information.pdf
Download PDF (1.1 MB)Acknowledgment
We thank Dr. Shinyo Gayama (Mitsubishi Gas Chemical Company) for kind gifts of PQQ and Bio-PQQ™.
Disclosure statement
No potential conflict of interest was reported by the authors.
Notes
Abbreviations: ALA, (±)-α-lipoic acid; α-toco, DL-α-tocopherol; Bio-PQQ™, pyrroloquinoline quinone disodium salt; CHO, chinese hamster ovary; ETC, electron transport chain; FRET, fluorescence resonance energy transfer; GSTA2, glutathione S-transferase A2; HO-1, heme oxygenase-1; Lyc, lycopene; MTT, 3-(4,5-dimethylthiazol-2-yl)-2,5-diphenyltetrazolium bromide; Nrf2, nuclear factor erythroid-2-related factor 2; PQQ, pyrroloquinoline quinone; Res, resveratrol; ROS, reactive oxygen species; SAC, S-allyl-L-cysteine; Ses, sesamin; SFN, DL-sulforaphane.
References
- Tzagoloff A. Mitochondria. New York (NY): Plenum Press; 1982.
- Sohal RS, Sohal BH, Orr WC. Mitochondrial superoxide and hydrogen peroxide generation, protein oxidative damage, and longevity in different species of flies. Free Radical Biol. Med. 1995;19:499–504.10.1016/0891-5849(95)00037-X
- Lin MT, Beal MF. Mitochondrial dysfunction and oxidative stress in neurodegenerative diseases. Nature. 2006;443:787–795.10.1038/nature05292
- Rooyackers OE, Adey DB, Ades PA, et al. Effect of age on in vivo rates of mitochondrial protein synthesis in human skeletal muscle. Proc. Nat. Acad. Sci. USA. 1996;93:15364–15369.10.1073/pnas.93.26.15364
- Trifunovic A, Larsson NG. Mitochondrial dysfunction as a cause of ageing. J. Int. Med. 2008;263:167–178.10.1111/jim.2008.263.issue-2
- Turner C, Schapira AH. Mitochondrial dysfunction in neurodegenerative disorders and ageing. Adv. Exp. Med. Biol. 2001;487:229–251.10.1007/978-1-4615-1249-3
- Balaban RS, Nemoto S, Finkel T. Mitochondria, oxidants, and aging. Cell. 2005;120:483–495.10.1016/j.cell.2005.02.001
- Agati G, Matteini P, Goti A, et al. Chloroplast-located flavonoids can scavenge singlet oxygen. New Phytol. 2007;174:77–89.10.1111/j.1469-8137.2007.01986.x
- Foyer CH, Noctor G. Redox homeostasis and antioxidant signaling: a metabolic interface between stress perception and physiological responses. Plant Cell. 2005;17:1866–1875.10.1105/tpc.105.033589
- Karamac M. Chelation of Cu(II), Zn(II), and Fe(II) by tannin constituents of selected edible nuts. Int. J. Mol. Sci. 2009;10:5485–5497.10.3390/ijms10125485
- Lee WC, Li LC, Chen JB, et al. Indoxyl sulfate-induced oxidative stress, mitochondrial dysfunction, and impaired biogenesis are partly protected by vitamin C and N-acetylcysteine. Sci. World J. 2015;2015:620826.
- Bonfili L, Cecarini V, Amici M, et al. Natural polyphenols as proteasome modulators and their role as anti-cancer compounds. FEBS J. 2008;275:5512–5526.10.1111/j.1742-4658.2008.06696.x
- Gorrini C, Harris IS, Mak TW. Modulation of oxidative stress as an anticancer strategy. Nat. Rev. Drug Discovery. 2013;12:931–947.10.1038/nrd4002
- Kitada T, Asakawa S, Hattori N, et al. Mutations in the parkin gene cause autosomal recessive juvenile parkinsonism. Nature. 1998;392:605–608.
- Keller JN, Huang FF, Markesbery WR. Decreased levels of proteasome activity and proteasome expression in aging spinal cord. Neuroscience. 2000;98:149–156.10.1016/S0306-4522(00)00067-1
- Keller JN, Hanni KB, Markesbery WR. Possible involvement of proteasome inhibition in aging: implications for oxidative stress. Mech. Ageing Dev. 2000;113:61–70.10.1016/S0047-6374(99)00101-3
- Maharjan S, Oku M, Tsuda M, et al. Mitochondrial impairment triggers cytosolic oxidative stress and cell death following proteasome inhibition. Sci. Rep. 2014;4:5896.
- Robinson KM, Janes MS, Beckman JS. The selective detection of mitochondrial superoxide by live cell imaging. Nat. Protoc. 2008;3:941–947.10.1038/nprot.2008.56
- Robinson KM, Janes MS, Pehar M, et al. Selective fluorescent imaging of superoxide in vivo using ethidium-based probes. Proc. Nat. Acad. Sci. USA. 2006;103:15038–15043.10.1073/pnas.0601945103
- Yano T, Oku M, Akeyama N, et al. A novel fluorescent sensor protein for visualization of redox states in the cytoplasm and in peroxisomes. Mol. Cell. Biol. 2010;30:3758–3766.10.1128/MCB.00121-10
- Kumazawa T, Sato K, Seno H, et al. Levels of pyrroloquinoline quinone in various foods. Biochem. J. 1995;307:331–333.10.1042/bj3070331
- Misra HS, Rajpurohit YS, Khairnar NP. Pyrroloquinoline-quinone and its versatile roles in biological processes. J. Biosci. 2012;37:313–325.10.1007/s12038-012-9195-5
- Urakami T, Yashima K, Kobayashi H, et al. Production of pyrroloquinoline quinone by using methanol-utilizing bacteria. Appl. Environ. Microbiol. 1992;58:3970–3976.
- Goosen N, Horsman HP, Huinen RG, et al. Acinetobacter calcoaceticus genes involved in biosynthesis of the coenzyme pyrrolo-quinoline-quinone: nucleotide sequence and expression in Escherichia coli K-12. J. Bacteriol. 1989;171:447–455.
- Toyama H, Chistoserdova L, Lidstrom ME. Sequence analysis of pqq genes required for biosynthesis of pyrroloquinoline quinone in Methylobacterium extorquens AM1 and the purification of a biosynthetic intermediate. Microbiology. 1997;143:595–602.10.1099/00221287-143-2-595
- Schnider U, Keel C, Voisard C, et al. Tn5-directed cloning of pqq genes from Pseudomonas fluorescens CHA0: mutational inactivation of the genes results in overproduction of the antibiotic pyoluteorin. Appl. Environ. Microbiol. 1995;61:3856–3864.
- He K, Nukada H, Urakami T, et al. Antioxidant and pro-oxidant properties of pyrroloquinoline quinone (PQQ): implications for its function in biological systems. Biochem. Pharmacol. 2003;65:67–74.10.1016/S0006-2952(02)01453-3
- Boots AW, Kubben N, Haenen GR, et al. Oxidized quercetin reacts with thiols rather than with ascorbate: implication for quercetin supplementation. Biochem. Biophys. Res. Commun. 2003;308:560–565.10.1016/S0006-291X(03)01438-4
- Boots AW, Li H, Schins RP, et al. The quercetin paradox. Toxicol. Appl. Pharmacol. 2007;222:89–96.10.1016/j.taap.2007.04.004
- Stahl W, Sies H. Lycopene: a biologically important carotenoid for humans? Arch. Biochem. Biophys. 1996;336:1–9.10.1006/abbi.1996.0525
- Nguyen ML, Schwartz SJ. Lycopene: chemical and biological properties. food Technol. 1999;53:38–45.
- Nagae S, Ushijima M, Hatono S, et al. Pharmacokinetics of the garlic compound S-allylcysteine. Planta Med. 1994;60:214–217.10.1055/s-2006-959461
- Engin KN. Alpha-tocopherol: looking beyond an antioxidant. Mol. Vis. 2009;15:855–860.
- Lodge J, Youn H, Handelman G, et al. Natural sources of lipoic acid: determination of lipoyllysine released from protease-digested tissues by high performance liquid chromatography incorporating electrochemical detection. J. Appl. Nutr. 1997;49:3–11.
- Ganin H, Rayo J, Amara N, et al. Sulforaphane and erucin, natural isothiocyanates from broccoli, inhibit bacterial quorum sensing. Med. Chem. Commun. 2013;4:175–179.10.1039/C2MD20196H
- Lee YJ, Lee SH. Sulforaphane induces antioxidative and antiproliferative responses by generating reactive oxygen species in human bronchial epithelial BEAS-2B Cells. J. Korean Med. Sci. 2011;26:1474–1482.10.3346/jkms.2011.26.11.1474
- Misiewicz I, Skupinska K, Kowalska E, et al. Sulforaphane-mediated induction of a phase 2 detoxifying enzyme NAD(P)H:quinone reductase and apoptosis in human lymphoblastoid cells. Acta Biochim. Pol. 2004;51:711–721.
- Shi H, Jing X, Wei X, et al. S-allyl cysteine activates the Nrf2-dependent antioxidant response and protects neurons against ischemic injury in vitro and in vivo. J. Neurochem. 2015;133:298–308.10.1111/jnc.2015.133.issue-2
- Ito Y, Kosuge Y, Sakikubo T, et al. Protective effect of S-allyl-L-cysteine, a garlic compound, on amyloid beta-protein-induced cell death in nerve growth factor-differentiated PC12 cells. Neurosci. Res. 2003;46:119–125.10.1016/S0168-0102(03)00037-3
- Godbout JP, Berg BM, Kelley KW, et al. α-Tocopherol reduces lipopolysaccharide-induced peroxide radical formation and interleukin-6 secretion in primary murine microglia and in brain. J. Neuroimmunol. 2004;149:101–109.10.1016/j.jneuroim.2003.12.017
- Bohm F, Tinkler JH, Truscott TG. Carotenoids protect against cell membrane damage by the nitrogen dioxide radical. Nat. Med. 1995;1:98–99.10.1038/nm0295-98
- Koenig ML, Meyerhoff JL. In vitro neuroprotection against oxidative stress by pre-treatment with a combination of dihydrolipoic acid and phenyl-butyl nitrones. Neurotox Res. 2003;5:265–272.10.1007/BF03033384
- Hara H, Hiramatsu H, Adachi T. Pyrroloquinoline quinone is a potent neuroprotective nutrient against 6-hydroxydopamine-induced neurotoxicity. Neurochem. Res. 2007;32:489–495.10.1007/s11064-006-9257-x
- Zhang Y, Rosenberg PA. The essential nutrient pyrroloquinoline quinone may act as a neuroprotectant by suppressing peroxynitrite formation. Eur. J. Neurosci. 2002;16:1015–1024.10.1046/j.1460-9568.2002.02169.x
- Takahashi S, Nakashima Y. Repeated and long-term treatment with physiological concentrations of resveratrol promotes NO production in vascular endothelial cells. Br. J. Nutr. 2012;107:774–780.10.1017/S0007114511003588
- Harris CB, Chowanadisai W, Mishchuk DO, et al. Dietary pyrroloquinoline quinone (PQQ) alters indicators of inflammation and mitochondrial-related metabolism in human subjects. J. Nutr. Biochem. 2013;24:2076–2084.10.1016/j.jnutbio.2013.07.008
- Rosen RT, Hiserodt RD, Fukuda EK, et al. Determination of allicin, S-allylcysteine and volatile metabolites of garlic in breath, plasma or simulated gastric fluids. J. Nutr. 2001;131:968S–971S.
- Petersen Shay K, Moreau RF, Smith EJ, et al. Is α-lipoic acid a scavenger of reactive oxygen species in vivo? Evidence for its initiation of stress signaling pathways that promote endogenous antioxidant capacity. IUBMB Life. 2008;60:362–367.10.1002/(ISSN)1521-6551
- Rota C, Chignell CF, Mason RP. Evidence for free radical formation during the oxidation of 2′-7′-dichlorofluorescin to the fluorescent dye 2′-7′-dichlorofluorescein by horseradish peroxidase:possible implications for oxidative stress measurements. Free Radical Biol. Med. 1999;27:873–881.10.1016/S0891-5849(99)00137-9
- Brand MD, Affourtit C, Esteves TC, et al. Mitochondrial superoxide: production, biological effects, and activation of uncoupling proteins. Free Radical Biol. Med. 2004;37:755–767.10.1016/j.freeradbiomed.2004.05.034
- Gonzalez-Freire M, de Cabo R, Bernier M, et al. Reconsidering the role of Mitochondria in Aging. J. Gerontol A Biol. Sci. Med. Sci. 2015.