Abstract
The L-aspartate:L-alanine antiporter of Tetragenococcus halophilus (AspT) possesses an arginine residue (R76) within the GxxxG motif in the central part of transmembrane domain 3 (TM3)—a residue that has been estimated to transport function. In this study, we carried out amino acid substitutions of R76 and used proteoliposome reconstitution for analyzing the transport function of each substitution. Both l-aspartate and l-alanine transport assays showed that R76K has higher activity than the AspT-WT (R76), whereas R76D and R76E have lower activity than the AspT-WT. These results suggest that R76 is involved in AspT substrate transport.
Graphical abstract
Site-specific replacement of Arg76 in transmembrane region of Asp: Ala antiporter affected transport activities, suggesting the importance of Arg76 for transport function.
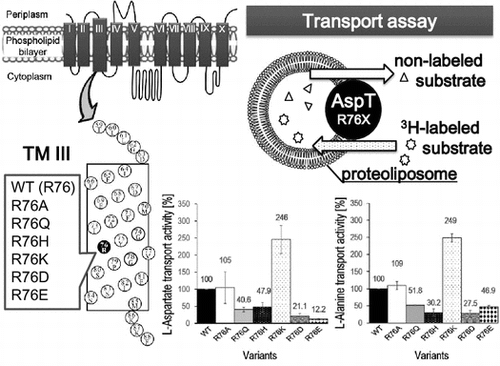
Recent studies using Corynebacterium glutamicum have found that a release channel (NCgl1221 mechanosensitive channel) plays an important role in glutamate production,Citation1) and substrate importers have also been shown to have a key role in ethanol production by yeast.Citation2) The importance of transporters responsible for substrate uptake and product release in fermentation that uses microorganisms is gradually being recognized, and studies paving the way for their technological utilization are currently underway.Citation3) The l-aspartate:l-alanine antiporter of Tetragenococcus halophilus (AspT) imports extracellular l-aspartate into the cell and releases intracellular l-alanine outside it. AspT acts in conjunction with aspartate decarboxylase (AspD) to create a proton concentration gradient and a membrane potential across the cell membrane, contributing to ATP synthesis by F1Fo-ATPase.Citation4,5) AspT is a member of the aspartate:alanine exchanger (AAEx) family (TC# 2.A.81) of secondary transporters in the transporter classification database (http://www.tcdb.org/index.php).Citation6,7) We previously demonstrated that AspT likely possesses ten transmembrane domains by single cysteine-scanning method,Citation8) and studies of substrate specificity suggest that l-aspartate and l-alanine each have different substrate binding sites.Citation9) Frequently seen in membrane proteins is the GxxxG motif, which helps to stabilize their structure via helix–helix interactions.Citation10) The GxxxG motif is also located right in the middle of transmembrane domain 3 (TM3) of AspT, from residues 74 to 78, and is highly conserved among AspT homologs. This suggests that TM3 constitutes the probable substrate translocation pathway of AspT.Citation8) Although as a transmembrane domain TM3 is highly hydrophobic, its GxxxG motif contains a charged arginine (R) amino acid residue at its center. Here, we focused on the functional role of R76. To investigate the possibility that R76 may be involved in AspT substrate transport, we generated variants with R76 substituted by the neutral amino acid residues alanine (A) and glutamine (Q), the basic amino acid residues histidine (H) and lysine (K), and the acidic amino acid residues aspartate (D) and glutamate (E), and reconstituted the mutants into proteoliposome to analyze the transport function of each substitution.
Materials and methods
Escherichia coli C43 (F– ompT hsdSB (rB– mB–) gal dcm (DE3)) was used to express AspT-WT (R76), R76A, R76Q, R76H、R76K, R76D, and R76E. The bacteria were shaking cultured at 37°C in 500 mL Luria-Bertani (LB) medium (30 mM d-glucose, 30 μg/mL carbenicillin, 1 mM pyridoxal 5′-phosphate, 50 mM l-aspartate (potassium salt), pH 7.0), and when the absorbance at 660 nm (A660) reached roughly 0.4, 0.2 mM isopropyl-β-d-thiogalactoside (IPTG) was added to induce expression. The bacteria were harvested at A660 ≒ 0.9, and after cell disruption, the membrane fraction was collected. Purification was then carried out by following the method of Sasahara.Citation9) Briefly, 2% n-dodecyl-β-d-maltoside was used to solubilize the membrane proteins, the His-tag of the solubilized AspT was adsorbed onto the Co2+ in 500 μL of TALON metal affinity resin (Clontech Laboratories, Inc., Mountain View, CA) (500 μL bed volume for a 2 L culture). After washing, AspT was then eluted by K-Pi containing 500 mM of imidazole. The protein concentration of the purified AspT was measured by the use of SDS-PAGE, and the purity of each R76 variant was confirmed as a single band on the gel. Proteoliposome reconstitution was carried out by filtering multi-lamellar vesicles (MLVs) produced from 5 μg aliquots of purified protein and 5% E. coli lipid (Avanti, Alabaster, AL) through a 1 μm polycarbonate (PC) membrane (Avanti, Alabaster, AL).Citation11) Proteoliposomes were produced by following Sasahara’sCitation9) dilution method, in which proteoliposomes are formed when the detergent in the detergent–lipid–protein mix drops below the critical micelle concentration (CMC) during dilution. In this process, the dilution buffer was adjusted so that the substrate concentration in the solution contained within the proteoliposomes was 100 mM l-aspartate or 50 mM l-alanine. l-[2,3-3H]aspartate or l-[2,3-3H]alanine (American Radiolabeled Chemicals, Inc., St. Louis, MO) was added to the outside of the reconstituted proteoliposomes, and 3H uptake into the proteoliposomes was measured one minute after this addition (n = 3 × 3). Criterion TGX Precast Gels (10%) (Bio-Rad Laboratories, Inc., Berkeley, CA) were used to quantitate the proteins reconstituted into proteoliposomes. Purified AspT (Cysless)-His (0–20 ng) was used as the standard protein. After electrophoresis, staining was carried out with Lumitein Protein Gel Stain (Biotium, Inc., Hayward, CA). Gel detection was carried out with a LAS-4000 imaging system (GE Healthcare, Piscataway, NJ), and ImageJ softwareCitation12) (US National Institutes of Health, Bethesda, MD, USA; http://imagej.nih.gov/ij/, 1997–2014) was used for quantitation.
Results and discussion
The genome sequences that have recently been determined for many bacteria reveal that many species possess AAEx family transporters. Fukui et al.Citation13) classified AAEx family transporters into four clusters. Alignment analysis of the amino acid sequences of TM3s in the AAEx family transporters suggests that GxxxG motif, and its boundaries in TM3 are highly conserved (Fig. ). Although GxxxG motifs are located in the central regions of hydrophobic TM3s, the amino acid corresponding to R76 in GxxxG motif of AspT is hydrophilic and varied. Therefore, we investigated whether R76 contributes to transport function.
Fig. 1. Multiple sequence alignment of members of the AAEx family of transporters.
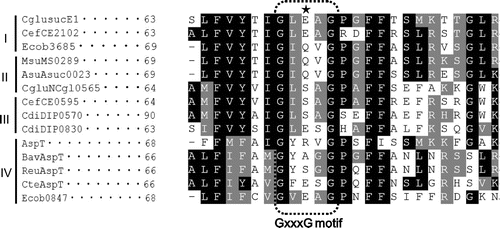
The substitution variants were purified from E. coli cell membranes expressing each R76 substitution, and each purified fraction was subjected to SDS-PAGE. Single bands were seen in the 57.2 kDa position, confirming that single purified proteins had been obtained (data not shown). Quantitation of the purified proteins using ImageJ software revealed that different amounts of protein were obtained for each variant (Table ). There was a roughly twofold difference in concentration between R76E, the variant with the lowest concentration, and R76K, the variant with the highest, suggesting that AspT expression differed between variants. On the basis of the quantitation results, 5 μg aliquots of the purified variants were reconstituted into proteoliposomes. To calculate the protein amounts reconstituted into proteoliposomes, aliquots of the proteoliposomes (3 μL) were subjected to SDS-PAGE, and the reconstituted proteins were quantitated (Fig. ).
Table 1. Concentrations of purified R76 variants.
Fig. 2. AspT variants reconstitution into proteoliposomes.
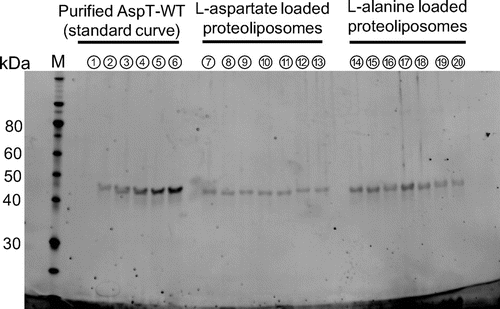
The exchange reaction between the non-radioactive substrate (l-aspartate or l-alanine) loaded in the proteoliposomes and the radioactive substrate ([3H] l-aspartate or [3H] l-alanine) outside the proteoliposomes was measured using the proteoliposomes reconstituted with each variant. The relative transport activity of each variant is shown in Fig. , with the transport activity of the wild type (WT) as 100%. This showed that R76K in particular exhibited a level of activity almost twice that of the WT, whereas R76D and R76E showed roughly one-third the activity of the WT. That the highest activity was exhibited by R76K, a basic residue, and the lowest activities by the acidic residues R76D and R76E, suggested that differences in electric charge may have some effect on substrate transport rate. That the changes in activity levels of both l-aspartate and l-alanine transport exhibited similar trends, however, suggested that the positive charge on R does not interact directly with the negative charge on the substrate l-aspartate to cause the transport reaction, but rather that R76 may contribute to structural stability by forming salt bridges with other negative amino acid residues of AspT. Because the size exclusion chromatography (SEC) profiles of the GxxxG variants were similar to the SEC profile of authentic AspT (Cysless AspT) (data not shown), there may not be marked differences in dimer (or oligomer) structure across the R76 variants. The possibility that the amino acid substitutions at R76 position induce disorder of the dimeric or oligomeric structure of AspT cannot be completely excluded. It is further necessary to analyze detailed dimeric (or oligomeric) structures of the AspT R76 variants using SEC with a multi angle light scattering detector (MALS).
Fig. 3. Analysis of the l-aspartate (A) or l-alanine (B) transport capacities of R76 variants.
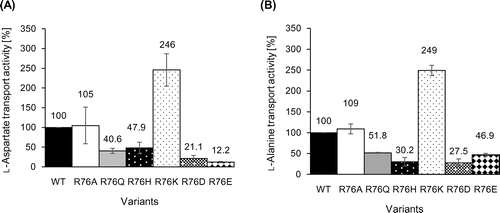
In the AAEx family, there are several homologs that possess E corresponding to R76 position of T. halophilus AspT of which R76E variant showed the lowest transport activity.Citation8) Because residue 76 is the least well conserved of the residues in the GxxxG motif (Fig. ), R76 may be involved in substrate selectivity. Analysis of the transport function of R76 variants for substrates other than l-aspartate and l-alanine may provide support for its involvement in substrate selectivity. AspT has four GxxxG motifs in its TM1, TM2, TM3, and reentrant loop 4 between TM4 and TM5.Citation14) If the GxxxG motifs of one AspT molecule interact with those of another AspT molecule, and the two AspT molecules consequently form a dimer or an oligomer, then GxxxG (S/A) motifs in TMs other than TM3 might be involved in dimerization or oligomerization.Citation15,16)
That R76K exhibited a transport activity nearly twice that of the WT in this study indicates that a single amino acid substitution increased the transport rate of the transporter. That the expression of R76K was higher than that of the other variants, including the WT, also indicated that modifying the transporter promises to offer an effective method of improving the productivity of industrial fermentation by microorganisms. Our amino acid substitutions of R76 may have affected adjacent amino acid residues that are functionally important.
Going forward, we intend to continue carrying out similar analyses of transport function, using variants with amino acid substitutions for the various amino acid residues with a focus on TM3, which contains the GxxxG motif and is estimated to constitute the substrate translocation pathway. We will also aim to identify sites responsible for substrate recognition, binding, and kinetic regulation, in the hope that these will lead to industrial applications.
Author contributions
S. S., K. N., and K. A. designed the study. S. S. performed the experiments and analyzed the results. S. S., K. N., and K. A. prepared the manuscript. All authors discussed the results.
Funding
This work was supported partly by KAKENHI, a Grant-in-Aid for Challenging Exploratory Research (Japan Society for the Promotion of Science; [grant number 2265108 and 15K14911] to K. A., and Grant-in-Aid for JSPS Fellows [grant number 25⋅3082] to S. S.). This study was also partly supported by an NISR Research Grant from the Noda Institute for Scientific Research (to K. A.).
Disclosure statement
No potential conflict of interest was reported by the authors.
Acknowledgments
We thank the Kikkoman Corporation for the gift of the Tetragenococcus halophilus asp operon. We thank Dr. Futai (Graduate School of Agricultural Science, Tohoku University) and Dr. Yoshimura (Liposome Engineering Laboratory, Inc., Graduate School of Engineering, Mie University) for their advice.
References
- Nakamura J, Hirano S, Ito H, et al. Mutations of the Corynebacterium glutamicum NCgl1221 gene, encoding a mechanosensitive channel homolog, induce L-glutamic acid production. Appl. Environ. Microbiol. 2007;73:4491–4498.10.1128/AEM.02446-06
- Enquist-Newman M, Faust AM, Bravo DD, et al. Efficient ethanol production from brown macroalgae sugars by a synthetic yeast platform. Nature. 2014;505:239–243.
- Yamashita C, Hashimoto K, Kumagai K, et al. L-Glutamate secretion by the N-terminal domain of the corynebacterium glutamicum NCgl1221 mechanosensitive channel. Biosci. Biotechnol. Biochem. 2013;77:1008–1013.10.1271/bbb.120988
- Abe K, Hayashi H, Maloney PC. Exchange of aspartate and alanine: mechanism for development of a proton-motive force in bacteria. J. Biol. Chem. 1996;271:3079–3084.10.1074/jbc.271.6.3079
- Abe K, Ohnishi F, Yagi K, et al. Plasmid-encoded asp operon confers a proton motive metabolic cycle catalyzed by an aspartate-alanine exchange reaction. J. Bacteriol. 2002;184:2906–2913.10.1128/JB.184.11.2906-2913.2002
- Saier MH Jr, Tran CV, Barabote RD. TCDB: the transporter classification database for membrane transport protein analyses and information. Nucleic Acids Res. 2006;34:D181–D186.10.1093/nar/gkj001
- Saier MH Jr, Yen MR, Noto K, et al. The transporter classification database: recent advances. Nucleic Acids Res. 2009;37:D274–D278.10.1093/nar/gkn862
- Nanatani K, Maloney PC, Abe K. Structural and functional importance of transmembrane domain 3 (TM3) in the aspartate:alanine antiporter AspT: topology and function of the residues of TM3 and oligomerization of AspT. J. Bacteriol. 2009;191:2122–2132.10.1128/JB.00830-08
- Sasahara A, Nanatani K, Enomoto M, et al. Substrate specificity of the aspartate:alanine antiporter (AspT) of Tetragenococcus halophilus in reconstituted liposomes. J. Biol. Chem. 2011;286:29044–29052.10.1074/jbc.M111.260224
- Russ WP, Engelman DM. The GxxxG motif: a framework for transmembrane helix-helix association. J. Mol. Biol. 2000;296:911–919.10.1006/jmbi.1999.3489
- Fukushima H, Mizutani M, Imamura K, et al. Development of a novel preparation method of recombinant proteoliposomes using baculovirus gene expression systems. J. Biochem. 2008;144:763–770.10.1093/jb/mvn125
- Schneider CA, Rasband WS, Eliceiri KW. NIH Image to ImageJ: 25 years of image analysis. Nat. Methods. 2012;9:671–675.10.1038/nmeth.2089
- Fukui K, Koseki C, Yamamoto Y, et al. Identification of succinate exporter in Corynebacterium glutamicum and its physiological roles under anaerobic conditions. J. Biotechnol. 2011;154:25–34.10.1016/j.jbiotec.2011.03.010
- Nanatani K, Fujiki T, Kanou K, et al. Topology of AspT, the aspartate:alanine antiporter of Tetragenococcus halophilus, determined by site-directed fluorescence labeling. J. Bacteriol. 2007;189:7089–7097.10.1128/JB.00088-07
- Overton MC, Chinault SL, Blumer KJ. Oligomerization, biogenesis, and signaling is promoted by a glycophorin A-like dimerization motif in transmembrane domain 1 of a yeast G protein-coupled receptor. J. Biol. Chem. 2003;278:49369–49377.10.1074/jbc.M308654200
- Kohlway A, Pirakitikulr N, Barrera FN, et al. Hepatitis C virus RNA replication and virus particle assembly require specific dimerization of the NS4A protein transmembrane domain. J. Virol. 2014;88:628–642.10.1128/JVI.02052-13