Abstract
The hypersensitive response (HR), a type of programmed cell death that is accompanied by DNA degradation and loss of plasma membrane integrity, is a common feature of plant immune responses. We previously reported that transcription of IREN which encodes a novel EF-hand containing plant nuclease is controlled by OsNAC4, a key positive regulator of HR cell death. Transient overexpression of IREN in rice protoplasts also led to rapid DNA fragmentation, while suppression of IREN using RNA interference showed remarkable decrease of DNA fragmentation during HR cell death. Maximum DNA degradation associated with the recombinant IREN was observed in the presence of Ca2+ and Mg2+ or Ca2+ and Mn2+. Interestingly, DNA degradation mediated by the recombinant IREN was completely abolished by Zn2+, even when Ca2+, Mg2+, or Mn2+ were present in the reaction buffer. These data indicate that IREN functions in the degradation of nuclear DNA during HR cell death.
Graphical abstract
IREN, novel EF-hand containing plant nuclease, functions in the degradation of nuclear DNA during immune-related hypersensitive cell death.
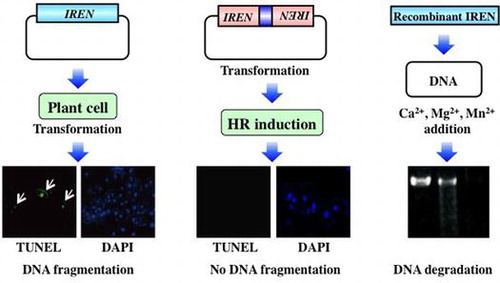
Programmed cell death (PCD) is a regulated physiological process involving cell death to remove unwanted and damaged cells.Citation1) In plants, PCD is essential to several aspects of plant life, including development and defense responses. The best-studied PCD process in plants is the hypersensitive response (HR), in which cell death occurs in response to avirulent pathogens.Citation2) The HR occurs at the site of attempted pathogen invasion, and in concert with several other defense responses, restricts the growth and spread of pathogens to other parts of the plant.Citation3,4) The recognition of avirulent pathogens by plants leads to HR cell death by a mechanism that involves the cellular efflux of anions and potassium and the influx of calcium and hydrogen ions.Citation5) Following the ion influx, the affected cells generate reactive oxygen species (ROS). The ROS cause membrane peroxidation and oxidative cross-linking of cell wall glycoproteins, and ROS may also act as a diffusible signal to induce subsequent immune responses involving the induction of immune-related genes.Citation6)
Several morphological and biochemical similarities were found between the HR cell death and apoptosis, the most typical form of PCD in animals. Apoptosis is accompanied by a distinct set of morphological and biochemical features, e.g. cell shrinkage, membrane blebbing, nuclear condensation, and DNA fragmentation.Citation7) The HR cell death also demonstrates similar morphological and biochemical traits including plasma membrane and nuclear condensation, loss of plasma membrane integrity and nuclear DNA fragmentation resulting from inter-nucleosomal cleavage.Citation8–12) Caspases function as the principal proteases that are activated and cleave a variety of proteins during apoptosis.Citation13) Although whole-genome sequencing has revealed that plants lack obvious homologs of animal caspases, a family of cysteine protease named metacaspases was found in plants.Citation14,15) Despite these morphological and biochemical similarities, several characteristics differ between the HR cell death and apoptosis. For example, apoptotic bodies, which in animal cells are phagocytosed by other cells, are not formed during plant HR cell death. Similarly, Bcl-2 family proteins, which play key roles in apoptosis, have not been identified in plants.Citation16) In addition, vacuoles, which are multifunctional plant cell organelles, play an important role in plant PCD, with a key role for the vacuolar processing enzyme (VPE).Citation17) These differences between the HR cell death and apoptosis indicate that the HR cell death in plant proceeds through a different mechanism than that which operates during apoptosis. Although the existence of the HR cell death in plants has been recognized for many years, the molecular mechanisms governing HR cell death remain poorly understood.
Acidovorax avenae can infect a wide range of monocotyledonous plants, although individual strains of the pathogen infect only one or a few host species.Citation18,19) We recently reported that a rice-avirulent strain, A. avenae N1141, caused clear HR cell death in rice cells accompanied with DNA fragmentation, the loss of plasma membrane integrity and typical cell morphological changes, including plasma membrane shrinkage and nuclear condensation.Citation8,10) We also demonstrated that the OsNAC4 gene exhibited rapid and transient transcriptional activation during the early stages of the HR cell death in rice cells. OsNAC4 contains a plant-specific transcription activation region (TAR)Citation20) and a NAC domain, which was originally defined based on a consensus sequence found in petunia NAMCitation21) and Arabidopsis ATAF1, ATAF2, and CUC2.Citation22) Overexpression of OsNAC4 in rice plants induced a form of cell death accompanied by the loss of plasma membrane integrity, nuclear DNA fragmentation, and morphological changes specific to the HR cell death.Citation10) The presence of these domains suggests that OsNAC4 is a plant-specific transcription factor functioning as a component of the cell death pathway in the HR cell death. In cell lines where OsNAC4 levels were suppressed by RNAi, HR cell death was markedly decreased in response to the A. avenae avirulent N1141 strain. Gene expression profiling using a rice cDNA microarray comparing wild-type and OsNAC4 RNAi cell lines demonstrated that OsNAC4 controls the transcription of IREN (immune-related endonuclease), which encodes a novel plant nuclease. The product of this gene contains an endonuclease motif in the N-terminal region and an EF hand motif, with a Ca2+-binding site, in the C-terminal region. Although several endonucleases, including caspase-activated DFF/CAD-ICADCitation23) and endonuclease G,Citation24) function to mediate DNA fragmentation during apoptosis, plant endonucleases related to HR cell death have not been clearly identified.
In this study, we examine the nuclease properties of IREN and its potential role in the induction of HR cell death. IREN is translocated into the nucleus using two nuclear localization signals during HR cell death. Transient overexpression of IREN led to rapid DNA fragmentation and DNA laddering in rice protoplasts and the recombinant IREN digested the double stranded DNA in vitro. By contrast, suppression of IREN using RNAi technique showed remarkable decrease of DNA fragmentation during HR cell death. These data indicate that IREN functions in the degradation of nuclear DNA during HR cell death. Moreover, DNA degradation by IREN overexpression did not trigger the rapid HR cell death, while IREN overexpression caused suppression of cell grown in yeast. From these results, we considered that although the DNA degradation by IREN overexpression dose not trigger the rapid HR cell death, the DNA degradation finally causes the suppression of cell growth.
Materials and methods
Plants and bacteria
Suspension cultures of rice cells (line Oc) were grown with R2S medium at 30 °C under light irradiation. The cells were diluted in fresh medium every 7 days, and experiments were performed 4 days after transfer. Acidovorax avenae avirulent strain N1141 (MAFF 301141) isolated from finger millet was used as previously described.Citation19,25) Saccharomyces cerevisiae strains BY4743 (MATa/α his3 Δ 1/his3 Δ 1 leu2 Δ 0/leu2 Δ 0 MET15/met5 Δ 0 LYS2/lys2 Δ 0 ura3 Δ 0/ura3 Δ 0) obtained from Euroscarf (Frankfurt, Germany) was cultured in YPD containing 2% peptone, 1% bactopeptone and 2% glucose.
Subcellular localization of IREN
IREN (Accession No. AK100514) was amplified by PCR from rice (Oryza sativa) cDNAs using gene specific primers (5′-CACCATGGCGAAGAAGCAGCGG-3′ and 5′-GGGGGTTCGAGGGGTGC-3′). The PCR product was cloned into pENTR-D-TOPO (Invitrogen, Carlsbad, CA), and the resulting plasmid was designated IREN/pENTR-D-TOPO. The Venus genes were amplified and cloned into pBI221 vector possessing the Gateway cassette (Invitrogen). The resulting vector was designated GW-Venus/pBI221. For the construction of Venus-fused proteins, the IREN/pENTR-D-TOPO plasmids were converted to a gateway-compatible destination vector (GW-Venus/pBI221) by the Gateway vector conversion system. Venus-fused NLS1-deleted IREN plasmid, Venus-fused NLS2-deleted IREN plasmid, and Venus-fused NLS1,2-deleted IREN plasmid were constructed using a KOD-Plus-mutagenesis kit (Toyobo, Osaka, Japan). The resulting plasmids were designated Venus-fused IREN/pBI221, Venus-fused NLS1-deleted IREN/pBI221, and Venus-fused NLS2-deleted IREN/pBI221 and Venus-fused NLS1,2-deleted IREN/pBI221, respectively.
Suppression of IREN using RNAi
Target fragment (508 bp; −113 to 395) of the IREN gene was initially amplified by PCR using specific primer set (forward, 5′-CACCCAAATCGCAGCGACAGCAAG-3′ and reverse, 5′-CCGTCTCCACGATTGTTGGT-3′). The resulting PCR product was cloned into the pENTR/D-TOPO cloning vector (Invitrogen). The target sequence was transferred into the pANDAmini vectorCitation26) by an LR clonase reaction. The resulting construct was designated pANDAmini-IREN. pANDAmini-IREN and pAHC17-DsRed or pANDAmini (empty vector) and pAHC17-DsRed were co-introduced into cultured rice cells using particle bombardment and the transformed cells were cultured with R2S medium for 24 h. The transformed cells were inoculated with the A. avenae avirulent N1141 strain and then TUNEL staining was performed.
TUNEL assay
For detection of DNA fragmentation in rice, TUNEL procedure was performed as previously described.Citation8)
For detection of DNA fragmentation in yeast, TUNEL staining was performed essentially as previously described.Citation27) Yeast cells were fixed with 3.7% (v/v) formaldehyde for 1 h, and then treated with Zymolyase 20 T (650 μg/mL, Nacalai tesque, Kyoto, Japan) at 37 °C for 40 min in 200 μL sorbitol buffer (35 mM potassium phosphate buffer (pH 7.3), 1.2 M sorbitol, 0.5 mM MgCl2). The cells were washed three times with PBS and digested with proteinase K (20 μg/mL) containing 100 mM Tris–HCl (pH 8.0) and 50 mM EDTA at 37 °C for 1 h and washed three times with PBS. The cells were incubated with 0.1% Triton X-100 (v/v) and 0.1% citric acid (w/v) at 4 °C for 15 min and washed three times with PBS. The cells were subjected to TUNEL using DeadEnd Fluoromeric TUNEL system (Promega) at 37 °C for 60 min in the dark. After washing with PBS, DAPI was added to the cells (1 μg/mL) and the cells were observed by fluorescence microscopy.
DNA extraction and laddering analysis
Cultured rice cells were incubated with A. avenae avirulent N1141 strain (108 cfu/mL) at 30 °C for different periods after inoculation. Cultured rice cells were harvested and washed with 50 mM Hepes-KOH (pH 7.2) twice. The washed cultured rice cells were ground to a fine powder in liquid nitrogen. Half a milliliter of DNA extraction buffer containing 2% (w/v) hexadecyl trimethyl ammonium bromide (CTAB), 1.4 M NaCl, 0.1 M Tris–HCl (pH 8.0), 20 mM EDTA, and 1% (w/v) polyvinylpyrrolicone-30 were added to each ground rice cell powder and then incubated for 10 min at 65 °C. After adding 60 μL of 3 M sodium acetate, DNA was extracted with an equal volume of phenol/chloroform and centrifuged at 12,000 g for 5 min. The aqueous phase was transferred to a new tube and an equal volume of isopropyl alcohol was added. Extraction of DNA was washed with 70% cold ethanol, dried, and then incubated at 37 °C for 30 min in 0.5 mL Tris–EDTA (TE) buffer supplemented with 100 μg/mL RNase A. After adding 60 μL of 3 M sodium acetate, DNA was purified by extraction with an equal volume of phenol/chloroform and the aqueous phase was transferred to a new tube. The aqueous phase was added with 0.6 mL isopropyl alcohol, and was incubated at room temperature for 5 min and then at −20 °C for 15 min. The DNA mixture was centrifuged at 4 °C for 1 s to remove the large size DNA and then the DNA in the supernatant was precipitated by centrifugation for 15 min at approximately 12,000 g. After washing with cold 70% ethanol, DNA was quantitated by measuring the absorbance at 260 and 280 nm. Ten micrograms of the purified DNA were subjected to electrophoresis on 2% (w/v) agarose gel and stained with 0.5 μg/mL final concentration ethidium bromide. Gels were photographed using the ATTO CCD camera system (Tokyo, Japan).
Protoplast preparation and gene transformation
Rice protoplasts were isolated from cultured Oc rice cells and PEG-mediated transformation was performed as described previously.Citation28)
RNA isolation and quantitative real-time RT-PCR
Total RNA was isolated from cultured rice cells using an RNeasy Plant Mini Kit (QIAGEN, Hilden, Germany) according to the manufacturer’s instructions. Quantitative real-time RT-PCR was performed on an Opticon2 (Bio-Rad, Biorad, Hercules, CA) using a QuantiTect SYBR Green RT-PCR Kit (QIAGEN) with the IREN primer set (forward, 5′-CTGCCAGGCACTTTGTCAGT-3′ and reverse, 5′-TCTTGGCTGCAGCATGTAGG-3′). The sizes of the PCR products were checked to confirm that only products from mRNA were amplified in all real-time RT-PCR experiments. The fluorescence data produced sigmoidal amplification plots in which the number of cycles was plotted against fluorescence. Quantification of each mRNA was calculated with calibration curve which was prepared using standard gene of known template amounts (1 ng–0.1 pg) and corrected with reference data of 18S rRNA gene.Citation29)
Nuclei purification and immunoblot analysis
Cultured rice cells were harvested and washed with 50 mM Hepes-KOH (pH 7.2) twice. The washed cultured rice cells were ground to a fine powder in liquid nitrogen. Nuclei were isolated by Plant Nuclei Isolation/Extraction kit according to the manufacture’s protocol (Sigma). To generate the specific anti-IREN antibody, the C-terminal region of IREN, encompassing the region from Asn-128 to Arg-466, was cloned in-frame into the pET-28(a)+vector (Novagen). The resulting His tag fusion protein was overproduced in the BL21 (DE3) strain of E. coli and purified using a HisTrap Kit according to the manufacturer’s protocol (GE Healthcare). The purified recombinant protein (3 mg) was used to immunize rabbits. Antiserum was further purified by affinity chromatography with a HiTrap N-hydroxysuccinimide-activated column coupled to recombinant IREN.
The nuclear fraction and the cytosol fraction were separated with SDS-polyacrylamide gel (12.5%). The separated proteins were electrophoretically transferred to a nitrocellulose membrane (Millipore) with a Bio-Rad semidry blotter (Bio-Rad). Nonspecific binding was blocked with 3% BSA in PBS buffer for 1 h at room temperature. Immunoreactive polypeptides were detected using a peroxidase-conjugated goat antibody raised against rabbit IgG (Medical & Biological Laboratories, Nagoya, Japan) and visualization was performed with an enhanced immunechemiluminescence kit (ECL-plus, GE Healthcare) and chemiluminescence was detected by LAS-4000 (Fujifilm, Tokyo, Japan).
Characterization of nuclease activity
For detection of total nuclease activity, nuclear extracts were isolated from the A. avenae avirulent N1141 strain-inoculated cultured rice cells using Plant Nuclei Isolation/Extraction kit according to the manufacture’s protocol (Sigma). The nuclear extracts were subjected to electrophoresis using rice genome-containing SDS-polyacrylamide gel. After electrophoresis, the gel was incubated in 10 mM Tris–HCl (pH7.8) at 50 °C for 1 h to remove SDS. To refolding of protein, the gel was incubated in 10 mM Tris–HCl (pH7.8) at 4 °C for 16 h. After refolding, the gel was incubated in reaction buffer containing Mg2+ and Ca2+, and stained with ethidium bromide. Images were acquired using a CCD camera, and the intensity of DNA staining in the images was then estimated.
For detection of IREN nuclease activity, the expression plasmid pGEX-6p-3-IREN was constructed. IREN (Accession No. AK100514) was amplified by PCR from rice (Oryza sativa) cDNAs using gene specific primers. The sequences of the primer set were 5′-CGGGATCCGCAATGGCGAAGAAGAAGCAGCGGAG-3′ (forward) and 5′-CGGGATCCGGCTAGGGGGTTCGAGGGGT-3′ (reverse). The PCR product was cloned into pGEM-T vector (Promega) and transformed into E. coli DH5α. IREN cDNA was amplified from plasmid pGEM-T-IREN using 5′-GGATCCATGGCGAAGAAGCAGCG-3′ (BamHI site-attached forward primer) and 5′-CTCGAGGGGGGTTCGAGGGGT-3′ (XhoI site-attached reverse primer). The PCR product was ligated into the pCR-Blunt plasmid (Invitrogen) and the resulting plasmid was designated pGEX-6p-3-IREN. The BL21 (DE3) strain of E. coli (Novagen) was transformed with the pGEX-6p-3-IREN plasmid. The resulting GST-tag fusion IREN protein was overproduced in the BL21 (DE3) strain by addition of 0.1 mM isopropyl β-D-thiogalactopyranoside (IPTG). After overnight culture for 37 °C, the GST-fused IREN protein was purified by affinity chromatography with a HiTrap SP column (GE Healthcare), and the GST tag was removed from the purified protein using PreScission protease.
For estimation of DNA degradation activity of recombinant IREN, 1 μg of digested linear plasmid (pBluescript) was incubated with recombinant IREN protein in reaction buffer (250 mM Tris–HCl, pH 7.0, 4 mM EDTA) for 16 h at 30 °C. The digested DNAs were electrophoresed on a 1.25% agarose gel and then stained with ethidium bromide.
Yeast cell death assay
The amplified IREN and NLS1,2-deleted IREN genes were introduced into the XbaI and HindIII site of pEG (KT) (Merck, Darmstadt, Germany) with an In-Fusion HD Cloning Kit (Clontech, Mountain View, CA). The resulting plasmid was designated pEG-IREN and pEG-NLS1,2-deleted-IREN. The specific primer sets were as follows: pEG-IREN, 5′-CCCGGGAATTCTAGAAATGGCGAAGAAGCAGCGGA-3′ (XbaI site-attached forward primer) and 5′-CGATGAATTAAGCTTCTAGGGGGTTCGAGGGGTG-3′ (HindIII site-attached reverse primer); pEG-NLS1,2-deleted-IREN, 5′-CCCGGGAATTCTAGAAATGGTGTCCTGCACCACGTTCAAC-3′ (XbaI site-attached forward primer) and 5′-CGATGAATTAAGCTTCTAGGGGGTTCGAGGGGTG-3′ (HindIII site-attached reverse primer). BY4743 yeast cells were transformed with pEG (KT): (vector control), pEG-IREN and pEG-NLS1,2-deleted-IREN using the LiAc method.Citation30) Single colony of yeast cells containing each plasmid was streaked on synthetic complete plates (SC; 0.67% (w/v) Bacto-yeast nitrogen base w/o amino acids, 2% (w/v) glucose, 0.2% (w/v) Dropout mix, and 2% (w/v) Bacto Agar) lacking leucine (SC/-Leu) and then incubated at 30 °C for 2–3 days. For expression of the protein, these cells were streaked on SG/-Leu plates (similar to SC/-Leu but replacing 2% glucose for 2% (w/v) galactose) and then incubated at 30 °C for 2–3 days.
Results
Nuclease responsible for HR cell death in rice
When cultured rice cells were inoculated with the A. avenae avirulent N1141 strain, HR cell death was detected 3 h after inoculation, and the number of dead cells gradually increased through 9 h after inoculation (Supplemental Fig. S1, see Biosci. Biotechnol. Biochem., Web Site.). To identify the nuclease associated with HR cell death, we examined the timing of DNA fragmentation during induction of HR cell death in cultured rice cells inoculated with the A. avenae avirulent N1141 strain. Terminal deoxynucleotidyl transferase-mediated dUTP nick-end labeling (TUNEL), which labels the free 3′-OH groups of DNA, was used to quantify DNA fragmentation.Citation31) Cultured rice cells infected with the A. avenae avirulent N1141 strain were positively labeled with the TUNEL reagent 12 h after inoculation (Fig. (A)). The TUNEL signals overlapped with the nuclei, which are identified by 4′, 6-diamidino-2-phenylindole (DAPI) staining. By contrast, the number of TUNEL-positive nuclei in the control cells was considerably less than that observed in the A. avenae avirulent N1141 strain-infected cells. Next, we examined whether DNA laddering occurred in cultured rice cells after inoculation of the A. avenae avirulent N1141 strain. Nuclei were isolated from the cells at various times post-inoculation. Genomic DNA was purified from the nuclei and analyzed by agarose gel electrophoresis. DNA degradation consisting of 180 bp nucleosomal DNA fragmentation was observed in the A. avenae avirulent N1141 strain-inoculated cells, but not in control cells (Fig. (B)).
Fig. 1. DNA degradation in cultured rice cells inoculated with the A. avenae avirulent N1141 strain.
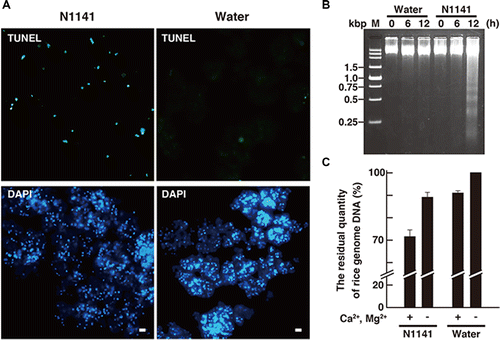
The induction of intensive DNA degradation during HR cell death would require the activation of nucleases. Nuclear extracts isolated from the A. avenae avirulent N1141 strain-inoculated cultured rice cells were subjected to electrophoresis on a rice genome-containing acrylamide gel. Intensive Mg2+ and Ca2+-dependent DNA degradation activity was observed when lysates prepared from the A. avenae avirulent N1141 strain-inoculated rice cells, but not from control cells, were added to rice genome DNA (Fig. (C)). These results suggested that the nuclease activity was increased in nuclei during induction of the HR cell death.
IREN as an endonuclease involved in HR cell death
To identify a nuclease involved in DNA degradation during HR cell death, we previously analyzed sequence motifs of genes that were upregulated by OsNAC4, a positive regulator of HR cell death.Citation10) Only one cDNA encoding a putative endonuclease was identified (AK100514), designated IREN. IREN is composed of 471 amino acids, and contains an endonuclease-exonuclease-phosphatase (EEP) domain and an EF-hand motif, comprising a Ca2+-binding site, in the C-terminal region (Supplemental Fig. S2, see Biosci. Biotechnol. Biochem., Web Site.). Motif analysis using PSORT (Genome NET Service, Osaka University, Japan)Citation32) revealed that two nuclear localization signals (NLS1 and NLS2) were observed in the N-terminal and middle regions of IREN (Supplemental Fig. S2, see Biosci. Biotechnol. Biochem., Web Site.). To determine which NLS contributed to the nuclear localization of IREN, a series of expression vectors containing deletions in one or both NLS regions-Venus-fused IREN, Venus-fused NLS1-deleted IREN/pBI221, Venus-fused NLS2-deleted IREN/pBI221 and Venus-fused NLS1,2-deleted IREN/pBI221 were constructed and introduced into protoplasts isolated from cultured rice cells. When a Venus-fused IREN/pBI221 was transformed into the protoplasts, IREN signals localized with the nuclei, indicating that IREN containing both NLS regions mainly localized to the nuclei (Fig. ).
Fig. 2. Impact of NLS1 and NLS2 on the subcellular localization of IREN in rice protoplasts.
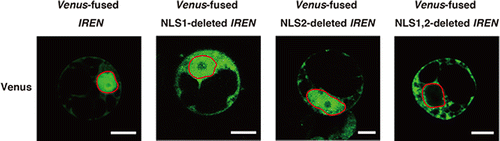
When either the Venus-fused NLS1-deleted IREN/pBI221 or the Venus-fused NLS2-deleted IREN/pBI221 was introduced into rice protoplasts, IREN-Venus signals were observed in both the cytoplasm and the nuclei. By contrast, in the protoplasts transformed by the Venus-fused NLS1, 2-deleted IREN/pBI221, IREN-Venus signals were observed in the cytosol, but not in the nuclei (Fig. ), suggesting that both NLS1 and NLS2 function as nucleus localization signals, and cooperate to achieve the nuclear translocation of IREN.
To clarify whether nuclear localization of IREN is necessary for it to mediate DNA degradation, we constructed a wild-type IREN expression vector (pBI221-IREN) and an NLS1, 2-deleted IREN expression vector (pBI221-NLS1,2-deleted IREN), and introduced each vector along with the reporter plasmid (pAHC17-DsRed: DsRed gene was under the control of the constitutive ubiquitin promoter derived from maizeCitation33)) into cultured rice cells using particle bombardment. After TUNEL staining, all of the IREN-expressing cultured rice cells (DsRed-positive cells) exhibited labeled nuclei (Fig. (A)). By contrast, no TUNEL positive nuclei were observed in cells that received either the NLS1, 2-deleted IREN expression vector (pBI221-NLS1, 2-deleted IREN), or the control pBI221 vector (Fig. (A)). We next examined whether DNA laddering occurred in IREN-transformed rice protoplasts. Rice protoplasts were transformed with the pBI221-IREN, pBI221-OsNAC4, or the control pBI221 empty vector. Twelve hours after transformation, genomic DNA was purified from the nuclei and analyzed by agarose gel electrophoresis. The DNA laddering was observed in IREN- or OsNAC4-transformed cells, but not in control cells (Fig. (B)), suggesting that the DNA fragmentation observed during HR cell death is caused by the IREN localization to the nucleus.
Fig. 3. The induction of DNA fragmentation by IREN in rice cells
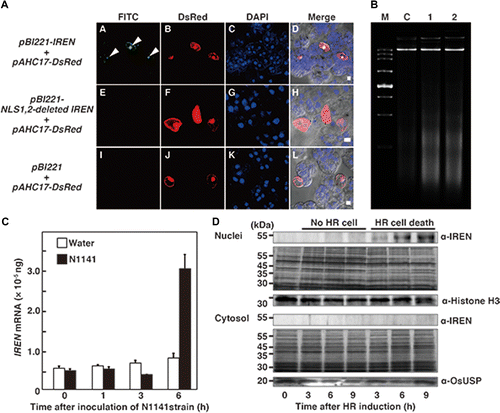
Next, we examined whether IREN protein accumulates in nuclei during HR cell death induced by the A. avenae avirulent N1141 strain. Real-time RT-PCR analysis revealed that the IREN transcript was induced 6 h post inoculation with the A. avenae avirulent N1141 strain (Fig. (C)). Immunoblot analysis using an anti-IREN antibody showed that inoculation with the A. avenae avirulent N1141 strain increased the amount of endogenous IREN protein in the nuclear fraction in a time-dependent manner, while treatment with water alone did not. The amount of IREN within the cytosolic fraction did not change after the A. avenae avirulent N1141 inoculation (Fig. (D)).
Suppression of IREN by RNA interference
To clarify whether IREN is involved in the HR-related DNA fragmentation, suppression of IREN by RNAi method was performed. We selected a 508 bp region of IREN cDNA that encodes the N-terminus for RNAi experiments. This contains the 5′UTR and the endonuclease domain, which is necessary for the nuclease activity of IREN. To determine whether this sequence is specific or shares similarity with other sequences, we searched the full-length rice cDNA database using BLASTN for short, nearly exact matches to the selected 508 bp region. No close similarity was detected for any genes other than IREN. Hence, the RNAi strategy was designed to target the IREN gene specifically. In this experiment, we wanted to measure mRNA levels in RNAi-expressed cells. However, quantity of IREN mRNA is technically very difficult because introduction efficiency is very low (approximately 5 to 10%). Therefore, we estimated an effect of RNAi by comparing the RNAi introduced cells with empty vector introduced cells. Gold particles for bombardment were coated with mixture of pANDAmini-IREN and pAHC17-DsRed or pANDAmini (empty vector) and pAHC17-DsRed and then bombarded into cultured rice cells, respectively. Twenty-four hours after transformation, the transformed cultured rice cells were inoculated with the A. avenae avirulent N1141 strain, and TUNEL staining was performed. The cultured rice cells transformed with pANDAmini (empty vector) and pAHC17-DsRed exhibited fluorescein-derived bright-green fluorescence of their nuclei (Fig. ). In contrast, remarkable decrease of bright-green fluorescence was observed in cultured rice cells co-transformed with pANDAmini-IREN and pAHC17-DsRed (Fig. ). These data indicated that IREN causes DNA fragmentation accompanied with HR cell death in rice.
Fig. 4. Decrease of HR-related DNA fragmentation in IREN-suppressed rice cells.
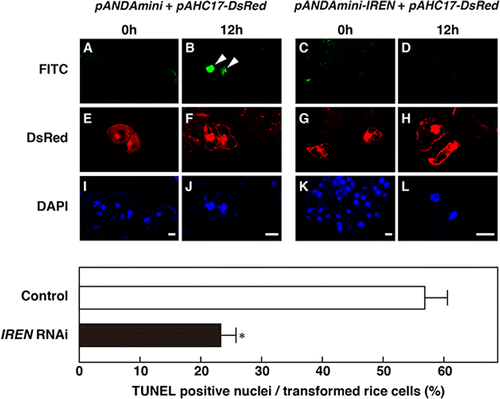
Characterization of IREN nuclease activity
To examine the nuclear DNA-degrading activity of IREN in vitro, GST-tagged IREN was prepared using an E. coli expression system and the GST moiety was removed using PreScission protease. Immunoblot analysis of the purified recombinant IREN protein revealed that the apparent molecular weight was 54 kDa, very close to the calculated molecular mass (data not shown). To confirm the nuclease activity of recombinant IREN, an in vitro nuclease assay using various metal ions and linear pBluescript as a substrate was performed. When recombinant IREN was incubated in a reaction buffer containing no metal ions, degradation of pBluescript was not observed (Fig. (A)). DNA degradation activity of IREN was detected when one of three metal ions (Ca2+, Mg2+, or Mn2+) was added to the reaction buffer. Maximum DNA degradation activity of the recombinant IREN was obtained in the presence of Ca2+ and Mg2+, or Ca2+ and Mn2+ (Fig. (A)), suggesting that the nuclease activity of recombinant IREN depended on Ca2+, Mg2+, or Mn2+. It is known that many DNases are activated by zinc (Zn2+). Interestingly, the DNA degradation activity of recombinant IREN was completely abolished by Zn2+, even in the presence of Ca2+, Mg2+, or Mn2+ (Fig. (A)), suggesting that Zn2+ functions as an inhibitor of IREN activity.
Fig. 5. Metal ions requirement on IREN nuclease activities
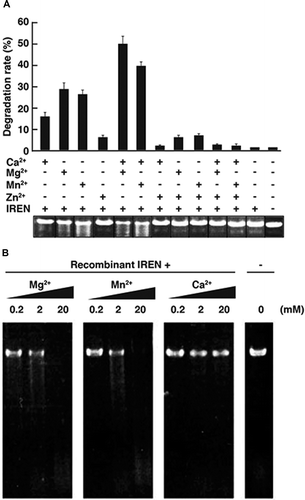
Then, we examined the dose-dependent activation of IREN Ca2+, Mg2+, and Mn2+. Linear pBluescript was slightly degraded by recombinant IREN in the presence of 2 mM Mg2+, and completely degraded in the presence of 20 mM of Mg2+. Similarly, 2 mM of Mn2+ also slightly activated DNA degradation by recombinant IREN, and the DNA was completely digested by the addition of 20 mM Mn2+ (Fig. (B)). By contrast, no notable DNA degradation activity was observed after the addition of 20 mM Ca2+ (Fig. (B)).
It is known that various DNA nucleases are activated at different pHs. To find the optimal pH for activity of the recombinant IREN, DNA degradation was measured under different pHs (Fig. ). IREN showed maximum activity at a pH range of 8.0–9.0 in the presence of 2 mM Mg2+. In the presence of 2 mM Ca2+, optimal DNA degradation was observed at pH 9.0. pH dependency in 2 mM Mn2+ was not measured because manganese ions were precipitated at pH 8.0 or above (data not shown).
Fig. 6. Effect of pH on the nuclease activity of recombinant IREN in the presence of various metal ions.
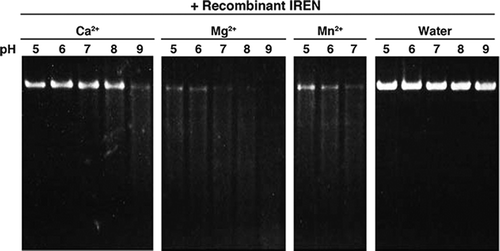
The role of IREN in the induction of cell death
We recently developed a transient assay system in cultured rice cells for evaluation of cell death.Citation10) Using particle gun bombardment, two plasmids, one containing the IREN gene and the other containing the uidA gene that encodes β-glucuronidase (GUS), were co-introduced into cultured rice cells. The IREN and uidA genes were under the control of the constitutive 35S promoter from CaMV and ubiquitin promoter derived from maize, respectively.Citation33) If IREN overexpression induced cell death, then little GUS activity would accumulate in the transformed cells. If overexpression did not induce cell death, then transformed cells would exhibit high levels of GUS activity. To determine the role of IREN in the induction of cell death, cultured rice cells were co-transfected with IREN and GUS plasmids (Fig. (A)). After 12 h transformation, although GUS activity in OsNAC4 plus uidA co-introduced cells was reduced, almost the same activity of GUS was detected in IREN/uidA cells and control uidA cells (Fig. (B)). Taken together, these results indicated that the DNA degradation by IREN is not the direct cause of cell death.
Fig. 7. The role of IREN in the induction of cell death.
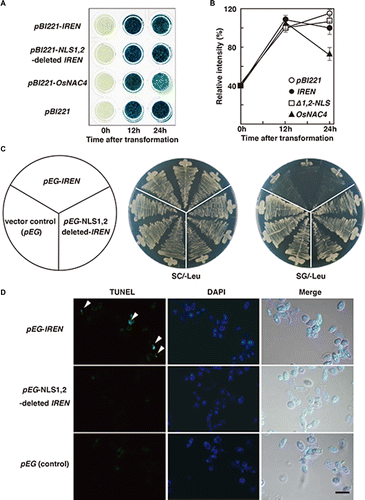
To evaluate the effect of IREN on cell growth, we introduced the IREN expression vector into yeast, and then measured cell death. BY4743 yeast cells were transformed with pEG-IREN, pEG-NLS1,2-deleted IREN, or pEG (control) and cultured on SG-Leu (induction) or SC-Leu (non-induction) plates. The same growth was observed in pEG-IREN-transformed yeast cells, pEG-NLS1,2-deleted IREN-transformed yeast cells, and pEG-transformed yeast cells (control) when the cells were cultured on SC-Leu plates. By contrast, when the cells were cultured on SG-Leu plates, pEG-IREN-transformed yeast cells grew extremely slowly (Fig. (C)), while yeast cells transformed with either pEG-NLS1,2-deleted IREN or pEG exhibited normal growth (Fig. (C)). Next, we examined DNA degradation in the transformed yeast cells by using TUNEL. pEG-IREN-transformed yeast cells showed significant TUNEL staining after 8 h induced-galactose (Fig. (D)). As expected, the TUNEL signals localized to the nuclei, which were identified by DAPI staining. pEG-NLS1,2-deleted IREN-transformed yeast cells and pEG-transformed yeast cells also showed some TUNEL positive nuclei, but the amount of signal was minor relative to that observed in the pEG-IREN-transformed yeast cells (Fig. (D)). Together, these results suggested that the degradation of nuclear DNA by IREN leads to the reduction of the cell growth.
Discussion
HR cell death occurs when a plant is attacked by an avirulent strain of a phytopathogenic organism. The most striking feature of HR cell death is the rapid DNA degradation in the nucleus. For example, DNA degradation has been demonstrated in TMV-inoculated tobacco,Citation34) fungus-infected cowpea,Citation35) bacteria-infected soybean,Citation36) and bacteria-infected rice.Citation8) Serial observations of nuclear DNA in living cells revealed that no detectable degradation occurred before the induction of HR cell death, but that DNA digestion was complete within 12 h after the induction of HR cell death (Fig. (B)), pointing to the presence of a DNA nuclease that was activated after the induction of HR cell death.
Despite many studies reporting the active degradation of genomic DNA in plants, the data is still relatively sparse regarding the number of deoxyribonucleases involved in particular biological processes. Genome-wide analyses revealed that 14 putative nucleases are present in the rice genome (Supplemental Fig. S2, see Biosci. Biotechnol. Biochem., Web Site.). These putative nucleases are classified into four large groups based on structural features. Group I, to which IREN belongs, is composed of the large EEP (exonuclease/endonuclease/phosphatase) superfamily that contains functionally diverse enzymes that share a common catalytic mechanism of cleaving phosphodiester bonds. Group II contains the S1-P1 nuclease family with homology to Bi-functional nuclease 1, e.g. BFN1/ENDO1 from Arabidopsis thaliana.Citation37,38) Group III contains the staphylococcal nuclease domain, e.g. EuCaN1/CaN2 from Eucommia ulmoidesCitation39) and group IV contains the homolog of OmBBD (for Oryza minuta bifunctional nuclease in basal defense response) that differ from the previously reported BFN family (Supplementary Fig. S2, see Biosci. Biotechnol. Biochem., Web Site.).Citation40,41) Several nucleases that are associated with plant cell death processes are reported.Citation42) DSA6 and BFN1 were expressed during senescent cell death; Bnuc1 was expressed in association with salt stress-induced cell death; and the endonuclease activities of ZEN1 from Zinnia and BEN1 from barley increase during programmed cell death in endosperm degradation or tracheary element differentiation.Citation43,44) Interestingly, all of the nucleases that have been previously associated with plant cell death processes are members of the S1-type nuclease family. Moreover, nuclear localization of IREN during HR cell death revealed that IREN degrades the genome DNA during HR cell death. Because IREN is classified in the EEP superfamily, it represents the first nuclease outside of the S1-type family that is demonstrated to be involved in HR cell death.
One common feature of animal apoptosis is the degradation of high molecular weight genomic DNA into smaller oligonucleosomal fragments. A ladder of 180 bp oligonucleosomes on an agarose gel is characteristic of apoptotic cells.Citation45,46) During the HR cell death in plants, a 180 bp DNA ladder and the activation of several nucleases have been reported,Citation35,47) but in some cases DNA laddering was not observed during HR cell death.Citation34) Therefore, the relationship of HR cell death and DNA laddering is still unclear. In here, we reported that 180 bp nucleosomal DNA laddering occurred during the HR cell death in cultured rice cells inoculated with avirulent N1141 strain of A. avenae.Citation11) The appearance of the DNA ladder during HR cell death indicates that nucleases digest double-stranded DNA. S1-type nuclease, such as ZEN1 and BEN1, degrades single-stranded DNA to yield 5′-phosphoryl terminated products.Citation43,44) By contrast, IREN digested double stranded DNA in the presence of Ca2+, Mg2+, or Mn2+ (Fig. ). These results raised the possibility that DNA laddering observed during HR cell death is caused by nucleases that digests double stranded DNA, such as IREN. In fact, we could show that overexpression of IREN in rice protoplasts leads to DNA laddering. Because the DNA laddering results from DNA cleavage at internucleosomal linker regions of chromosome, the DNA laddering is not observed in in vitro degradation of vector by the recombinant IREN. These strongly support the possibility that double stranded DNA-digesting nucleases cause the DNA laddering.
IREN possesses an EF-hand in its C-terminal region. The EF-hand motif contains a helix-loop-helix structure that resembles a hand with the forefinger and thumb (the two helices) extended at roughly right angles from the rest of the fingers, which are curled toward the palm (the loop).Citation48) The loop in each EF-hand motif consists of 12 residues, including conserved residues that coordinate a calcium ion. The EF-hand motif binds a Ca2+ ion in the loop and undergoes large conformational changes that result in the exposure of hydrophobic pockets, which in turn facilitate the interaction of the EF-hand-containing protein with a variety of protein partners. In most of the functional EF-hand motifs, the first amino acid within the loop is Asp and the twelfth is Glu.Citation49) The Glu residue contributes both of its side chain oxygen atoms to the metal ion. This consensus sequence was also found in the EF-hand motif of IREN, suggesting that its EF hand motif is functional and that Ca2+ binding to the EF hand causes large conformational changes in IREN that result in activity.
Eukaryotic nucleases are involved in several processes, including DNA restriction, repair, recombination, transposition, and programmed cell death. Plant nucleases can be classified into groups depending on their catalytic properties, largely described by their divalent metal ion requirements. Plants have two major classes of endonucleases, Zn2+- dependent and Ca2+-dependent.Citation49) Additional subgroups include nucleases whose activities are stimulated by other ions, including Mg2+, Mn2+, or Co2+.Citation50) Among these plant nucleases, Zn2+-dependent nucleases (also termed Type I or S1-type nucleases) are relatively well studied. This type contains neutral enzymes with a molecular mass between 30 and 45 kDa that efficiently degrade RNA and denatured DNA, but not double-stranded DNA. Several Zn2+-dependent nucleases, e.g. ZEN1, BEN1, and ENDO2, have been described in plants. Recently, CaMNUC32, classified as a Ca2+/Mg2+-dependent nuclease, was reported.Citation50) CaMNU32 activity is weak in the presence of Ca2+ or Mg2+ alone, but is much stronger in the presence of both Ca2+ and Mg2+. In addition, CaMNUC32 activity is inhibited by Zn2+ and by low pH.Citation51) A Ca2+/Mg2+-dependent nucleases with similar properties have been previously described in different systems. For example, rice OsCyt20 is a Ca2+/Mg2+-dependent nuclease that exhibits higher activity at neutral pH and is inhibited by Zn2+.Citation52) A Ca2+/Mg2+-dependent nuclease that is inhibited by Zn2+ has also been identified in wheat grain nucellar cells undergoing programmed cell death. A similar nuclease of 28 kDa was observed in calf thymus chromatin. Arabidopsis CAN1 and CAN2 nucleases are neutral, Ca2+-dependent nucleases displaying different specificities toward single-stranded DNA, double-stranded DNA, and RNA substrates.Citation53) In this study, we showed that IREN activity is slightly activated in the presence of Ca2+ or in the presence of Mg2+/Mn2+, but is strongly activated in the presence of both Ca2+ and Mg2+. In addition, IREN was inhibited by Zn2+ and degraded both single-stranded and double-stranded DNA (data not shown). These features of IREN agreed with those of a typical Ca2+/Mg2+-dependent nuclease. On the other hand, IREN is classified as part of the EEP superfamily, and contains an EF-hand motif. To our knowledge, this is the first example of a nuclease containing an EF-hand motif that is involved in HR cell death. Because in rice, IREN is the only the nuclease containing an EF-hand motif, IREN may be a unique nuclease specialized to mediate HR cell death.
DNA degradation in the range of 180 bp from cultured rice cells were observed 12 h after the A. avenae avirulent N1141 strain inoculation. By contrast, HR cell death was detected by Evans blue staining within 4 h after inoculation.Citation8) These data indicate that DNA degradation occurs at a late stage of HR cell death process. In addition, transient overexpression of IREN in cultured rice cells caused the DNA degradation, but not the loss of plasma membrane integrity accompanied with the HR cell death.Citation10) In the case of TMV-inoculated tobacco cells, activation of HR-related nucleases and DNA degradation also seemed to occur at a late stage of the cell death process.Citation34) Taken together, these results suggest that DNA degradation events are not the direct trigger of HR cell death induced by A. avenae avirulent strain but are rather downstream phenomena which are parts of the HR cell death. Interestingly, suppression of cell growth was observed when IREN expressed in yeast cells (Fig. ). Since DNA degradation by IREN would cause the decrease of gene expression, protein synthesis and metabolism, growth of cell was gradually suppressed. Actually, suppression of cell growth was observed in the yeast cells that the DNA was fragmented. Based on these, we considered that although the DNA degradation by IREN overexpression dose not trigger the rapid and programmed cell death, the DNA degradation by IREN finally causes the suppression of cell growth.
Author contributions
Yuka Ootsubo, Takanori Hibino and Fang-Sik Che conceived and designed the experiments. Yuka Ootsubo, Takanori Hibino, Takahito Wakazono, and Yukio Mukai performed the experiments. Yuka Ootsubo, Takanori Hibino, and Fang-Sik Che analyzed data. Yuka Ootsubo, Takanori Hibino, and Takahito Wakazono contributed reagents/materials/analysis tools. Yuka Ootsubo and Fang-Sik Che wrote the paper.
Supplemental material
The supplemental material for this paper can be accessed at http://dx.doi.org/10.1080/09168451.2015.1123610.
FigS2_BBB.eps
Download EPS Image (1.5 MB)Supplemental_data150643.docx
Download MS Word (14.7 KB)FigS1_BBB.eps
Download EPS Image (657.5 KB)Acknowledgments
The authors acknowledge Hiromi Morii for excellent technical support.
Disclosure statement
No potential conflict of interest was reported by the authors.
Notes
The nucleotide sequence reported in this paper has been submitted to NCBI database accession numbers AK100514 (IREN).
References
- Coll NS, Epple P, Dangl JL. Programmed cell death in the plant immune system. Cell Death Differ. 2011;18:1247-1256.10.1038/cdd.2011.37
- Dangl JL, Jones JD. Plant pathogens and integrated defence responses to infection. Nature. 2001;411:826-833.10.1038/35081161
- Heath MC. Hypersensitive response-related death. Plant Mol. Biol. 2000;44:321–334.10.1023/A:1026592509060
- Tian D, Wang J, Zeng X, et al. The rice TAL effector-dependent resistance protein XA10 triggers cell death and calcium depletion in the endoplasmic reticulum. Plant Cell. 2014;26:497–515.10.1105/tpc.113.119255
- Grant M, Brown I, Adams S, et al. The RPM1 plant disease resistance gene facilitates a rapid and sustained increase in cytosolic calcium that is necessary for the oxidative burst and hypersensitive cell death. Plant J. 2000;23:441–450.10.1046/j.1365-313x.2000.00804.x
- Lehmann S, Serrano M, L’Haridon F, et al. Reactive oxygen species and plant resistance to fungal pathogens. Phytochemistry. 2015;112:54–62.10.1016/j.phytochem.2014.08.027
- Geske FJ, Gerschenson LE. The biology of apoptosis. Hum. Pathol. 2001;32:1029–1038.10.1053/hupa.2001.28250
- Che FS, Iwano M, Tanaka N, et al. Biochemical and morphological features of rice cell death induced by Pseudomonas avenae. Plant Cell Physiol. 1999;40:1036–1045.10.1093/oxfordjournals.pcp.a029485
- Coffeen WC, Wolpert TJ. Purification and characterization of serine proteases that exhibit caspase-like activity and are associated with programmed cell death in Avena sativa. Plant Cell. 2004;16:857-873.10.1105/tpc.017947
- Kaneda T, Taga Y, Takai R, et al. The transcription factor OsNAC4 is a key positive regulator of plant hypersensitive cell death. EMBO J. 2009;28:926-936.10.1038/emboj.2009.39
- Tanaka N, Nakajima Y, Kaneda T, et al. DNA laddering during hypersensitive cell death in cultured rice cells induced by an incompatible strain of Pseudomonas avenae. Plant Biotech. 2001;18:295–299.
- Yao N, Tada Y, Park P, et al. Novel evidence for apoptotic cell response and differential signals in chromatin condensation and DNA cleavage in victorin-treated oats. Plant J. 2001;28:13–26.10.1046/j.1365-313X.2001.01109.x
- Kroemer G, Martin SJ. Caspase-independent cell death. Nat. Med. 2005; 11: 725-730.10.1038/nm1263
- Carmona-Gutierrez D, Fröhlich KU, Kroemer G, et al. Metacaspases are caspases. Doubt no more. Cell Death Differ. 2010;17:377–378.10.1038/cdd.2009.198
- Enoksson M, Salvesen GS. Metacaspases are not caspases-always doubt. Cell Death Differ. 2010; 17: 1221.10.1038/cdd.2010.45
- Kutuk O, Basaga H. Bcl-2 protein family: implications in vascular apoptosis and atherosclerosis. Apoptosis. 2006;11:1661–1675.10.1007/s10495-006-9402-7
- Hatsugai N, Kuroyanagi M, Yamada K, et al. A plant vacuolar protease, VPE, mediates virus-induced hypersensitive cell death. Science. 2004;305:855–858.10.1126/science.1099859
- Kadota I, Ohuchi A, Nishiyama K. Serological properties and specificity of pseudomonas avenae Manns 1909, the causal agent of bacterial brown stripe of rice. Ann Phytopathal Soc Jpn. 1991;57:268–273.10.3186/jjphytopath.57.268
- Kadota I, Mizuno A, Nishiyama K. Detection of a protein specific to the strain of Pseudomonas avenae Manns 1909 pathogenic to rice. Ann Phytopathol Soc Jpn. 1996;62:425–428.10.3186/jjphytopath.62.425
- Ooka H, Satoh K, Doi K, et al. Comprehensive analysis of NAC family genes in Oryza sativa and Arabidopsis thaliana. DNA Res. 2003;10:239-247.10.1093/dnares/10.6.239
- Souer E, van Houwelingen A, Kloos D, et al. The No Apical Meristem Gene of Petunia Is Required for Pattern Formation in Embryos and Flowers and Is Expressed at Meristem and Primordia Boundaries. Cell. 1996;85:159–170.10.1016/S0092-8674(00)81093-4
- Aida M, Ishida T, Fukaki H, et al. Genes involved in organ separation in Arabidopsis: an analysis of the cup-shaped cotyledon mutant. Plant Cell. 1997;9:841–857.10.1105/tpc.9.6.841
- Mukae N, Yokoyama H, Yokokura T, et al. Identification and developmental expression of inhibitor of caspase-activated DNase (ICAD) in Drosophila melanogaster. J. Biol. Chem. 2000;275:21402–21408.10.1074/jbc.M909611199
- Li LY, Luo X, Wang X. Endonuclease G is an apoptotic DNase when released from mitochondria. Nature. 2001;412:95–99.10.1038/35083620
- Che FS, Nakajima Y, Tanaka N, et al. Flagellin from an Incompatible strain of Pseudomonas avenae induces a resistance response in cultured rice cells. J. Biol. Chem. 2000;275:32347–32356.10.1074/jbc.M004796200
- Miki D, Shimamoto K. Simple RNAi vectors for stable and transient suppression of gene function in rice. Plant Cell Physiol. 2004;45:490–495.10.1093/pcp/pch048
- Kitagaki H, Araki Y, Funato K, et al. Ethanol-induced death in yeast exhibits features of apoptosis mediated by mitochondrial fission pathway. FEBS Lett. 2007;581:2935–2942.10.1016/j.febslet.2007.05.048
- Takai R, Kaneda T, Isogai A, et al. A new method for defense response analysis using a transient expression system in rice. Biosci. Biotechnol. Biochem. 2006;71:590-593.
- Jain M, Nijhawan A, Tyagi AK, et al. Validation of housekeeping genes as internal control for studying gene expression in rice by quantitative real-time PCR. Biochem. Biophys. Res. Commun. 2006;345:646–651.10.1016/j.bbrc.2006.04.140
- Schiestl RH, Gietz RD. High efficiency transformation of intact yeast cells using single stranded nucleic acids as a carrier. Curr. Genet. 1989;16:339–346.10.1007/BF00340712
- McCabe PF, Levine A, Meijer PJ, et al. A programmed cell death pathway activated in carrot cells cultured at low cell density. Plant J. 1997;12:267–280.10.1046/j.1365-313X.1997.12020267.x
- Nakai K, Kanehisa M. A knowledge base for predicting protein localization sites in eukaryotic cells. Genomics. 1992;14:897–911.10.1016/S0888-7543(05)80111-9
- Cornejo MJ, Luth D, Blankenship KM, et al. Activity of a maize ubiquitin promoter in transgenic rice. Plant Mol. Biol. 1993;23:567–581.10.1007/BF00019304
- Mittler R, Lam E. Identification, characterization, and purification of a tobacco endonuclease activity induced upon hypersensitive response cell death. Plant Cell. 1995;7:1951–1962.10.1105/tpc.7.11.1951
- Ryerson DE, Heath MC. Cleavage of nuclear DNA into oligonucleosomal fragments during cell death induced by fungal infection or by abiotic treatments. Plant Cell 1996;8:393–402.10.1105/tpc.8.3.393
- Levine A, Pennell RI, Alvarez ME, et al. Calcium-mediated apoptosis in a plant hypersensitive disease resistance response. Curr. Biol. 1996;6:427–437.10.1016/S0960-9822(02)00510-9
- Perez-Amador MA, Abler ML, De Rocher EJ, et al. Identification of BFN1, a bifunctional nuclease induced during leaf and stem senescence in Arabidopsis. Plant Physiol. 2000;122:169–180.10.1104/pp.122.1.169
- Triques K, Sturbois B, Gallais S, et al. Characterization of Arabidopsis thaliana mismatch specific endonucleases: application to mutation discovery by TILLING in pea. Plant J. 2007;51:1116–1125.10.1111/j.1365-313X.2007.03201.x
- Chen HM, Pang Y, Zeng J, et al. The Ca2+-dependent DNases are involved in secondary xylem development in Eucommia ulmoides. J. Integr. Plant Biol. 2012;54:456–470.10.1111/jipb.2012.54.issue-7
- Lesniewicz K, Karlowski WM, Pienkowska JR, et al. The plant S1-like nuclease family has evolved a highly diverse range of catalytic capabilities. Plant Cell Physiol. 2013;54:1064–1078.10.1093/pcp/pct061
- You MK, Shin HY, Kim YJ, et al. Novel bifunctional nucleases, OmBBD and AtBBD1, are involved in abscisic acid-mediated callose deposition in Arabidopsis. Plant Physiol. 2010;152:1015–1029.10.1104/pp.109.147645
- Sakamoto W, Takami T. Nucleases in higher plants and their possible involvement in DNA degradation during leaf senescence. J. Exp. Bot. 2014;65:3835–3843.10.1093/jxb/eru091
- Aoyagi S, Sugiyama M, Fukuda H. BEN1 and ZEN1 cDNAs encoding S1-type DNases that are associated with programmed cell death in plants. FEBS Lett. 1998;429:134–138.10.1016/S0014-5793(98)00563-8
- Ito J, Fukuda H. ZEN1 is a key enzyme in the degradation of nuclear DNA during programmed cell death of tracheary elements. Plant Cell. 2002;14:3201–3211.10.1105/tpc.006411
- Arends MJ, Morris RG, Wyllie AH. Apoptosis. The role of the endonuclease. Am. J. Pathol. 1990;136:593–608.
- Mesner PW Jr, Budihardjo II, Kaufmann SH. Chemotherapy-induced apoptosis. Adv. Pharmacol. 1997;41:461–499.10.1016/S1054-3589(08)61069-8
- Wang H, Li J, Bostock RM, et al. Apoptosis: a functional paradigm for programmed plant cell death induced by a host-selective phytotoxin and invoked during development. Plant Cell. 1996;8:375–391.10.1105/tpc.8.3.375
- Harmon AC. Calcium-regulated protein kinase of plants. Gravit. Space Biol. Bull. 2003;16:83–90.
- Tuteja N, Mahajan S. Calcium signaling network in plants: an overview. Plant Signal Behav. 2007;2:79–85.10.4161/psb.2.2.4176
- Sanchez-Pons N, Vicient CM. Identification of a type I Ca2+/Mg2+-dependent endonuclease induced in maize cells exposed to camptothecin. BMC Plant Biol. 2013;13:186–194.10.1186/1471-2229-13-186
- Jiang AL, Cheng Y, Li J, et al. A zinc-dependent nuclear endonuclease is responsible for DNA laddering during salt-induced programmed cell death in root tip cells of rice. J. Plant Physiol. 2008;165:1134–1141.10.1016/j.jplph.2007.12.008
- Domínguez F, Cejudo FJ. Identification of a nuclear-localized nuclease from wheat cells undergoing programmed cell death that is able to trigger DNA fragmentation and apoptotic morphology on nuclei from human cells. Biochem. J. 2006;397:529–536.10.1042/BJ20051809
- Leśniewicz K, Poręba E, Smolarkiewicz M, et al. Plant plasma membrane-bound staphylococcal-like DNases as a novel class of eukaryotic nucleases. BMC Plant Biol. 2012;12:195–214.10.1186/1471-2229-12-195