Abstract
Testosterone levels in men decrease with age; this decline has been linked to various diseases and can shorten life expectancy. Geranylgeraniol (GGOH) is an isoprenoid found in plants that plays an important role in several biological processes; however, its role in steroidogenesis is unknown. Here, we report that GGOH enhances the production of testosterone and its precursor progesterone in testis-derived I-10 tumor cells. GGOH induced protein kinase A (PKA) activity and increased cAMP levels and was found to regulate cAMP/PKA signaling by activating adenylate cyclase without altering phosphodiesterase activity. GGOH also stimulated mRNA and protein levels of steroidogenic acute regulatory protein, a downstream effector in the cAMP/PKA pathway. These results demonstrate that GGOH enhances steroidogenesis in testis-derived cells by modulating cAMP/PKA signaling. Our findings have potential applications for the development of therapeutics that increase testosterone levels in aging men.
Graphical abstract
Geranylgeraniol enhanced testosterone production by activating the cAMP/protein kinase A pathway in testis-derived I-10 tumor cells.
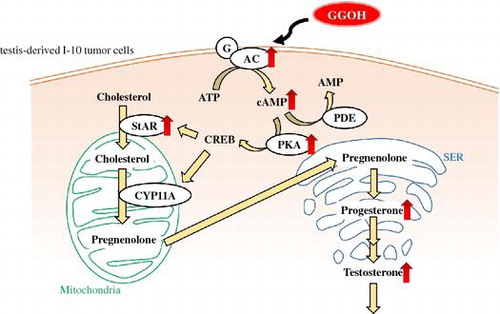
Geranylgeraniol (GGOH) is a C20 isoprenoid found in fruits, vegetables, and grainsCitation1) that plays an important role in several biological processes. GGOH may also have anti-tumorigenic effects against leukemia,Citation2) prostate cancer,Citation3) colon cancerCitation4) cells, and anti-inflammatory activity in rats.Citation5) However, the detailed mechanisms underlying these effects remain unclear.
Testosterone plays an important role in fetal development, sperm production, and the development of male secondary sex characteristics. A population-based study showed that free serum testosterone levels decreased with age in Japanese men aged 40–79 years.Citation6) Low testosterone levels cause infertility and sexual dysfunction in men, with a subset of men developing late-onset hypogonadism. Low testosterone can also predict the development of type 2 diabetes and cardiovascular disease and has been linked to increase risk of mortality in male veterans.Citation7–11)
In men, testosterone is produced by Leydig cells in the testicles. Steroidogenic acute regulatory protein (StAR) transports cholesterol to the inner mitochondrial membrane in these cells to initiate steroidogenesis. P450scc (also known as CYP11A), a cholesterol side chain cleavage enzyme, catalyzes a cascade of reactions that converts cholesterol to the steroid hormone precursor pregnenolone, which is converted to testosterone. Steroid synthesis is also regulated by multiple signaling events, including adenylate cyclase (AC) activation and elevation of intracellular cAMP levels, followed by activation of protein kinase A (PKA) and other steroidogenic proteins.Citation12) In our previous study, we reported that menaquinone-4 stimulated testosterone production in rats and testis-derived tumor cells via regulation of PKA activity.Citation13) Menaquinone-4 is composed of 2-methyl-1,4-naphthoquinone and a geranylgeranyl side chain that is considered the effector component rather than the naphthoquinone ring of menaquinone-4. Although some studies have described the potent effects of GGOH on cancer cells and inflammation, its role in steroidogenesis has never been reported.Citation2–5) We addressed this issue in this study using mouse testis-derived tumor cells.
Experimental procedures
Cell line and culture conditions
I-10 mouse testis-derived tumor cells were obtained from the Health Science Research Resources Bank (Osaka, Japan) and were maintained in Ham’s F-10 medium (Sigma-Aldrich, St. Louis, MO, USA) supplemented with 10% fetal bovine serum (Biowest, Nuaillé, France), 50 U/mL penicillin, and 50 mg/ml streptomycin in a 5% CO2 humidified incubator at 37 °C. Cells were used in experiments when they reached 80–90% confluence. GGOH was purchased from Sigma-Aldrich and dissolved in ethanol to obtain a stock solution (100 mM). H89 (Sigma-Aldrich), MDL12,330A (MDL; Calbiochem, San Diego, CA, USA), and 3-isobutyl-1-methylxanthine (IBMX; Cayman Chemical, Ann Arbor, MI, USA)–inhibitors of PKA, AC, and phosphodiesterase (PDE), respectively–were dissolved in dimethyl sulfoxide (Sigma-Aldrich) to obtain a stock solution (10 mM).
Measurement of testosterone and progesterone levels in culture medium
I-10 cells were seeded in 12-well plates at a density of 6.0 × 104 cells/well and incubated overnight. The culture medium was then replaced with fresh medium containing GGOH (3, 10, or 30 μM). After incubation for 0–24 h, the culture medium was collected and centrifuged at 1,000 × g for 5 min. Testosterone and progesterone concentrations in the supernatant were determined with specific enzyme immunoassay (EIA) kits (Cayman Chemical).
Reporter gene assay
FuGENE HD transfection reagent (Promega, Madison, WI, USA) was used to transfect cells with reporter plasmids. Cells were seeded in a 6-well plate and incubated overnight, and the medium was replaced with Opti-MEM (Gibco/Invitrogen, Carlsbad, CA, USA) containing the pGL4.29 and pmiwZ plasmids and transfection reagent. The pGL4.29 plasmid harboring the firefly luciferase gene under the control of the cAMP response element (CRE) was purchased from Promega; the pmiwZ plasmid containing the β-galactosidase reporter geneCitation14) was used as an internal control. After incubation for 24 h, fresh medium was added to the culture, followed by incubation for another 24 h; cells were then treated with GGOH for 3 h, collected, and lysed with Reporter Lysis Buffer (Promega). Luciferase and β-galactosidase activities were measured with the Luciferase Assay System (Promega) and Galacto-Star β-galactosidase reporter gene system (Invitrogen) using an AB-2250 luminometer (ATTO, Tokyo, Japan).
Measurement of cAMP levels
I-10 cells were seeded in 60-mm dishes and incubated overnight. The culture medium was refreshed with GGOH-containing medium, followed by incubation for 1.5 h. Cells were lysed with 0.1 M HCl and centrifuged at 1,000 × g for 10 min. The supernatant was acetylated by adding 4 M KOH and acetic acid anhydride before measurement. cAMP concentration in cell lysates was determined with a cAMP EIA kit (Cayman Chemical).
RNA interference
Stealth RNAi short interfering RNA (siRNA) for mouse Ac9 (Invitrogen) was used for gene knockdown experiments. The double-stranded siRNA sequence was as follows: 5′-CAU AGG AGU AGA AGA GGC CAG UGA A-3′. The negative control siRNA was also purchased from Invitrogen. Cells were seeded in a 6-well plate and transfected with 10 μM siRNA using Lipofectamine RNAiMAX Reagent (Invitrogen) according to the manufacturer’s instructions.
Quantitative reverse transcriptase-mediated PCR (qRT-PCR)
Total RNA were extracted from I-10 cells treated with different concentrations of GGOH for 3 h using the Isogen acid phenol–guanidine thiocyanate-based reagent (Nippon Gene, Tokyo, Japan) according to the manufacturer’s instructions. For cDNA synthesis, 4 μg of RNA was used as a template; the detailed procedure has been previously described.Citation15) PCR were carried out on an ABI 7300 Real-Time PCR system (Applied Biosystems, Foster City, CA, USA) using SYBR Premix EX Taq (Takara Bio, Otsu, Japan). Primer sequences are shown in Table .
Table 1. Nucleotide sequences of primers used for qRT-PCR.
Western blot analysis
I-10 cells were harvested by scraping, and total cellular protein was prepared in lysis buffer consisting of 50 mM Tris-HCl (pH 7.5), 150 mM NaCl, 0.1% SDS, 5 mM EDTA, Complete proteinase inhibitor cocktail, and PhosSTOP phosphatase inhibitor cocktail (both from Roche Applied Science, Mannheim, Germany). Protein concentrations were measured by the Lowry method. Cell extracts (10 μg protein) were resolved by electrophoresis on a 10–20% SDS polyacrylamide gel (Wako Pure Chemical Industries, Osaka, Japan) at 150 V for 150 min. Proteins were transferred to a polyvinylidene difluoride membrane (Millipore, Billerica, MA, USA), which was blocked overnight with blocking buffer containing 10 mM Tris-HCl (pH 7.5), 150 mM NaCl, 0.1% Tween 20, and 5% (w/v) skim milk. The membrane was then incubated with buffer (Can Get Signal; Toyobo Co., Tokyo, Japan) containing an antibody against StAR (Affinity BioReagents, Golden, CO, USA) for 1 h, followed by incubation with horseradish peroxidase-linked secondary antibody for 1 h at room temperature. Immunoreactive bands were detected with Immobilon Western Detection Reagent (Millipore) on an LAS-4000 mini luminescent image analyzer (Fujifilm, Tokyo, Japan). Relative expression levels of each protein were normalized to the amount of α-tubulin detected using a specific antibody (Sigma-Aldrich).
Statistical analysis
Data are expressed as mean ± SD and were analyzed with the Student’s t-test or by one-way analysis of variance followed by Tukey’s honestly significant difference test using SAS v.9.3 software (SAS Institute, Cary, NC, USA). A P value <0.05 was considered significant.
Results
GGOH and other isoprenoid derivatives stimulate testosterone and progesterone production
To determine whether GGOH enhances testosterone production, I-10 cells were treated with GGOH (0–30 μM for 0, 3, 9, 15, or 24 h). GGOH enhanced testosterone level in the culture medium in a dose- and time-dependent manner (Fig. (A)). Similarly, the level of progesterone–a testosterone precursor–was increased in a time- and dose-dependent manner following treatment with GGOH (Fig. (B) and (C)).
Fig. 1. GGOH stimulates testosterone and progesterone production in I-10 cells. Cells were treated with indicated concentrations of GGOH for 0–24 h. Testosterone (A) and progesterone levels in several time (B) and various concentrations for 24 h (C) in the culture medium were measured by EIA.
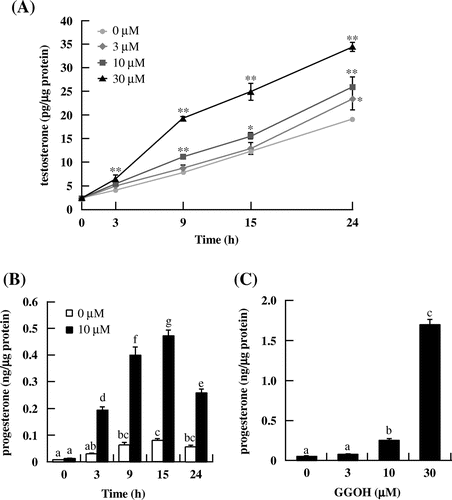
The ability of structurally related other isoprenoids to stimulate steroidogenesis was also examined. We selected geraniol (GOH) and farnesol (FOH) that have 2 and 3 isoprene units, respectively. And we examined phytol (POH) and geranylgeranyl diphosphate (GGPP) as chemicals structurally close to GGOH. Testosterone and progesterone levels in I-10 cells culture medium were markedly increased in the presence of POH (Fig. (A) and (C)) and GGPP (Fig. (B) and (D)) but not GOH (Fig. (A) and (C)). Meanwhile, FOH enhanced progesterone (Fig. (C)) but not testosterone (Fig. (A)) level. These results indicate that POH and GGOH had similar effects on testosterone and progesterone production, although unexpectedly, GOH did not affect steroidogenesis in I-10 cells.
Fig. 2. Effects of isoprenoid derivatives on testosterone and progesterone production in I-10 cells. Cells were treated with isoprenoid derivatives (GOH, FOH, POH, or GGPP) for 24 h. Testosterone (A, B) and progesterone (C, D) levels in the culture medium were measured by EIA.
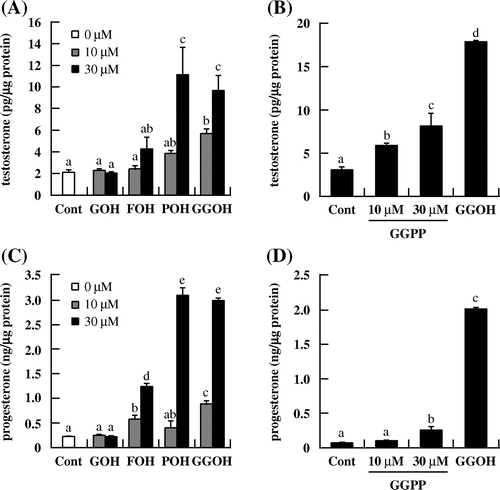
GGOH regulates PKA activity during steroidogenesis in a cAMP-dependent manner
We speculated that GGOH increased testosterone levels via activation of PKA. To test this hypothesis, we first assessed the effect of GGOH on PKA activity with the CRE-driven reporter gene assay. We found that GGOH (30 μM) induced luciferase activity relative to the control (Fig. (A)). The increase in progesterone level induced by GGOH was completely abolished by treatment with the PKA inhibitor H89 (Fig. (B)). In addition, intracellular cAMP level was increased in a dose-dependent manner by treatment with GGOH for 1.5 h (Fig. ). These results indicate that GGOH enhances progesterone production via regulation of PKA activity in a cAMP-dependent pathway.
Fig. 3. GGOH increases PKA activity in I-10 cells. (A) Cells were transfected with a CRE-inducible reporter gene and then treated with indicated concentrations of GGOH for 3 h. Reporter activity in cell lysates was measured with the luciferase assay. (B) I-10 cells were treated with H89 and GGOH for 3 h, and progesterone levels in the culture medium were measured by EIA.
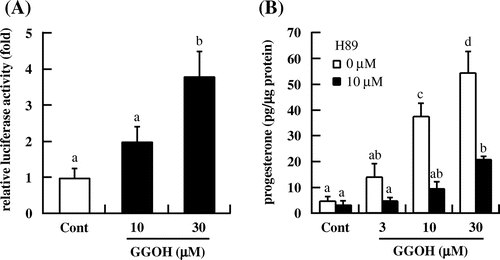
Fig. 4 GGOH stimulates intracellular cAMP levels in I-10 cells. Cells were treated with indicated concentrations of GGOH for 1.5 h, then collected and acetylated before measuring of cAMP levels in cell lysates by EIA.Notes: Data are presented as mean ± SD (n = 3). Different letters indicate significant differences (p < 0.05).
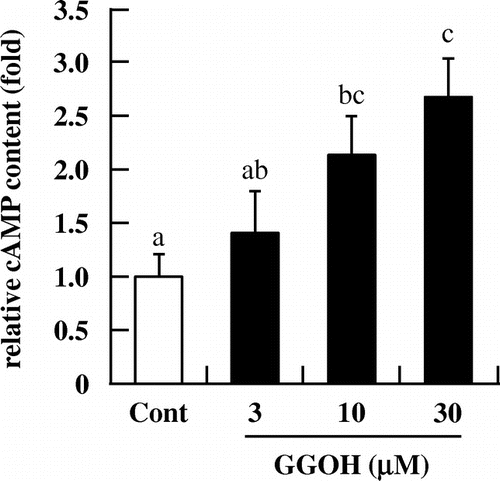
GGOH increases cAMP levels by modulating AC activity
To clarify the mechanism underlying the effects of GGOH on the cAMP/PKA pathway and steroidogenesis in I-10 cells, we inhibited the activities of two enzymes that regulate cAMP concentration–i.e., AC and PDE using MDL and IBMX, respectively. The GGOH-induced increase in progesterone level was suppressed in the presence of MDL (Fig. (A)) but not IBMX (Fig. (B)), suggesting that GGOH regulates cAMP concentration by inducing AC activity and not by suppressing PDE.
Fig. 5. GGOH induces progesterone production via regulation of AC but not PDE activity in I-10 cells. Cells were treated with GGOH and MDL12,330A (A) or IBMX (B) for 3 h, and progesterone levels in the culture medium were measured by EIA.
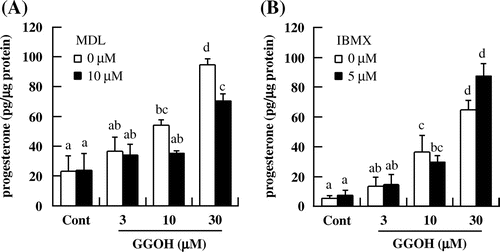
To confirm that GGOH stimulates steroidogenesis via induction of AC activity, we knocked down Ac expression using siRNA. The Ac9 isoform was highly expressed in I-10 cells (data not shown). The knockdown efficiency 48 h after transfection of Ac9 siRNA was about 53% (Fig. (A)). Cells were treated with GGOH for 3 h after siRNA transfection. In Ac9-deficient cells, GGOH treatment had no effect on progesterone levels relative to the control group (Fig. (B)). The result indicates that GGOH stimulates steroidogenesis via regulation of Ac9 activity.
Fig. 6. Effects of Ac9 knockdown on progesterone production in I-10 cells. (A) The mRNA levels of Ac9 after transfection of appropriate siRNA for 48 h were determined by qRT-PCR. (B) I-10 cells were transfected with siRNAs targeting Ac9, and progesterone levels in the culture medium were measured by EIA.
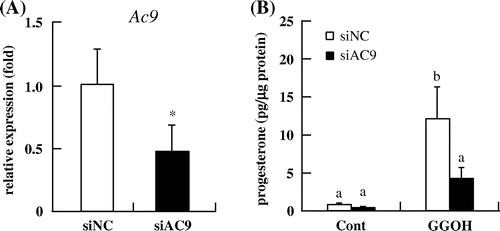
GGOH induces the upregulation of StAR expression
To further clarify the mechanism by which GGOH induces steroidogenesis via the cAMP/PKA axis, we measured Ac9, Cyp11a, and StAR mRNA and protein levels in I-10 cells following GGOH treatment. There was no change in Ac9 mRNA levels (Fig. (A)) or Cyp11a mRNA (Fig. (B)) or its protein expression (data not shown) upon GGOH application. However, StAR mRNA and protein levels were increased in the presence of 30 μM GGOH (Figs. (C), and ). These results demonstrate that GGOH induces the upregulation of StAR expression via the cAMP/PKA signaling pathway to enhance steroid production.
Discussion
We previously reported that menaquinone-4, one of vitamin K2, stimulates testosterone production in I-10 cells via regulation of PKA activityCitation13) and presumed that unsaturated side chain of menaquinone-4, geranylgeranyl group, may be important to express this activity. And we also found that both menaquinone-4 and GGOH have anti-inflammatory activity in lipopolysaccaride-induced inflammation model.Citation5,15) Here, we firstly demonstrated that GGOH stimulates steroidogenesis via activation of cAMP/PKA pathway.
Testosterone production is regulated by a complex signaling cascade, with cholesterol transported into mitochondria to initiate steroidogenesis in Leydig cells. Progesterone is an important hormone that is converted to several steroids–including testosterone–and is secreted by I-10 cells.Citation16,17) GGOH stimulated both testosterone and progesterone productions (Fig. ). Therefore, we measured progesterone levels as a precursor of testosterone in further experiments in Figs. (B), , and (C) to clarify detailed mechanism of GGOH in steroidogenesis.
Previous studies have found that GGOH restores the function of cells in which mevalonic acid has been depletedCitation18) and maintains the beneficial effects of cholesterol synthesis in THP-1 cells treated with a mevalonate pathway inhibitor,Citation19) suggesting that GGOH is an important metabolic precursor in this pathway after statin treatment. GGOH is hydrolyzed from GGPP by a specific pyrophosphatase enzyme, which is a common precursor of natural diterpenoids.Citation20) Recent evidence has revealed the biological activity of compounds derived from intermediates of the isoprenoid/cholesterol synthesis pathway. These isoprenoids–including FOH, GOH, and POH–regulate various biological processes.Citation20–22) The results of this study indicate that not only GGOH, but other isoprenoid derivatives can enhance testosterone and progesterone levels, although the mechanisms by which other isoprenoids exert these effects has yet to be determined.
cAMP is a second messenger between membrane receptors and intracellular targets that is formed and degraded by AC and PDE, respectively. There are 10 AC isoforms (AC1–10) in mammals; AC1–9 are transmembrane enzymes, while AC10 is soluble. The transmembrane forms are mainly regulated via G-protein-coupled receptors.Citation23,24) Human AC9 mRNA is highly expressed in skeletal muscle, heart, and brain;Citation25) our findings demonstrate that Ac9 is the most highly expressed isoform in I-10 cells and may regulate cAMP production. On the other hand, we did not find any evidence of a role for PDE in steroidogenesis. StAR functions in the rate-limiting step of steroid production in Leydig cells and is required for the delivery of cholesterol to the inner mitochondrial membrane.Citation26) We showed that GGOH treatment increased the RNA and protein levels of StAR, which acts downstream in the cAMP/PKA pathway. Taken together, our results demonstrate that GGOH enhances testosterone and progesterone production in I-10 cells via induction of cAMP/PKA signaling. These findings provide novel mechanistic insight into the process of steroidogenesis and may be useful for the development of therapeutic strategies to counter age-associated declines in testosterone levels in men.
Author contributions
HJ Ho and H Shirakawa designed the study and wrote the manuscript. R Yoshida and A Ito carried out the experiments shown in Figs. and and analyzed the data. M Maeda evaluated adenylate cyclase and phosphodiesterase mRNA levels. HJ Ho performed the other experiments. T Goto and M Komai contributed to the interpretation of results.
Funding
This work was partially supported by a Grant-in-aid for Scientific Research from the Japan Society for the Promotion of Science to HS [#20380071], [#23380070].
Disclosure statement
No potential conflict of interest was reported by the authors.
Notes
Abbreviations: AC, adenylate cyclase; FOH, farnesol; GGOH, geranylgeraniol; GGPP, geranylgeranyl diphosphate; GOH, geraniol; IBMX, 3-isobutyl-1-methylxanthine; MDL, MDL12,330A; PDE, phosphodiesterase; PKA, protein kinase A; POH, phytol; StAR, steroidogenic acute regulatory protein.
References
- Ahlquist L, Bergström G, Liljenberg C. Acyclic diterpene alcohols: occurrence and synthesis of geranylcitronellol, phytol and geranylgeraniol. Prog. Chem. Fats Other Lipids. 1978;16:231–255.10.1016/0079-6832(78)90046-0
- Masuda Y, Maeda S, Watanabe A, et al. A novel 21-kDa cytochrome c-releasing factor is generated upon treatment of human leukemia U937 cells with geranylgeraniol. Biochem. Biophys. Res. Commun. 2006;346:454–460.10.1016/j.bbrc.2006.05.161
- Fernandes NV, Yeganehjoo H, Katuru R, et al. Geranylgeraniol suppresses the viability of human DU145 prostate carcinoma cells and the level of HMG CoA reductase. Exp. Biol. Med. (Maywood). 2013; 238: 1265–1274.10.1177/1535370213492693
- Ohizumi H, Masuda Y, Nakajo S, et al. Geranylgeraniol is a potent inducer of apoptosis in tumor cells. J. Biochem. 1995;117:11–13.
- Giriwono PE, Shirakawa H, Ohsaki Y, et al. Dietary supplementation with geranylgeraniol suppresses lipopolysaccharide-induced inflammation via inhibition of nuclear factor-κB activation in rats. Eur. J. Nutr. 2013;52:1191–1199.10.1007/s00394-012-0429-y
- Okamura K, Ando F, Shimokata H. Serum total and free testosterone level of Japanese men: a population-based study. Int. J. Urol. 2005;12:810–814.10.1111/iju.2005.12.issue-9
- Huhtaniemi I. Late-onset hypogonadism: current concepts and controversies of pathogenesis, diagnosis and treatment. Asian J. Androl. 2014;16:192–202.10.4103/1008-682X.122336
- Shores MM, Matsumoto AM, Sloan KL, et al. Low serum testosterone and mortality in male veterans. Arch. Intern. Med. 2006;166:1660–1665.10.1001/archinte.166.15.1660
- Akishita M, Hashimoto M, Ohike Y, et al. Low testosterone level as a predictor of cardiovascular events in Japanese men with coronary risk factors. Atherosclerosis. 2010;210:232–236.10.1016/j.atherosclerosis.2009.10.037
- Ma RC, Tong PC. Testosterone levels and cardiovascular disease. Heart. 2010;96:1787–1788.10.1136/hrt.2010.207068
- Beatrice AM, Dutta D, Kumar M, et al. Testosterone levels and type 2 diabetes in men: current knowledge and clinical implications. Diabetes Metab. Syndr. Obes. 2014;7:481–486.
- Payne AH. Steroidogenic enzymes in Leydig cells. In: The Leydig Cell in Health and Disease. Payne AH, Hardy MP, editors. Totowa, NJ: Human Press; 2007. p. 157–171.10.1007/978-1-59745-453-7
- Ito A, Shirakawa H, Takumi N, et al. Menaquinone-4 enhances testosterone production in rats and testis-derived tumor cells. Lipids Health Dis. 2011;10:158.10.1186/1476-511X-10-158
- Suemori H, Kadodawa Y, Goto K, et al. A mouse embryonic stem cell line showing pluripotency of differentiation in early embryos and ubiquitous β-galactosidase expression. Cell Differ. Dev. 1990;29:181–186.10.1016/0922-3371(90)90120-L
- Ohsaki Y, Shirakawa H, Miura A, et al. Vitamin K suppresses the lipopolysaccharide-induced expression of inflammatory cytokines in cultured macrophage-like cells via the inhibition of the activation of nuclear factor κB through the repression of IKKα/β phosphorylation. J. Nutr. Biochem. 2010;21:1120–1126.10.1016/j.jnutbio.2009.09.011
- Shin S-I. Studies on interstitial cells in tissue culture: steroid biosynthesis in monolayers of mouse testicular interstitial cells. Endocrinology. 1967;81:440–448.10.1210/endo-81-3-440
- Ascoli M. Immortalized Leydig cell lines as models for studying Leydig cell physiology. In: The leydig cell in health and disease. Payne AH, Hardy MP, editors. Totowa, NJ: Human Press; 2007. p. 373–381.10.1007/978-1-59745-453-7
- Ownby SE. and Hohl RJ. Farnesol and geranylgeraniol: Prevention and reversion of lovastatin-induced effects in NIH3T3 cells. Lipids. 2002;37:185–192.10.1007/s11745-002-0879-1
- Campia I, Lussiana C, Pescarmona G, et al. Geranylgeraniol prevents the cytotoxic effects of mevastatin in THP-1 cells, without decreasing the beneficial effects on cholesterol synthesis. Br. J. Pharmacol. 2009;158:1777–1786.10.1111/j.1476-5381.2009.00465.x
- Holstein SA, Hohl RJ. Isoprenoids: remarkable diversity of form and function. Lipids. 2004;39:293–309.10.1007/s11745-004-1233-3
- Joo JH, Jetten AM. Molecular mechanisms involved in farnesol-induced apoptosis. Cancer Lett. 2010;287:123–135.10.1016/j.canlet.2009.05.015
- Chowdhury RR, Ghosh SK. Phytol-derived novel isoprenoid immunostimulants. Front. Immunol. 2012;3:49.
- Gancedo JM. Biological roles of cAMP: variations on a theme in the different kingdoms of life. Biol. Rev. Camb. Philos. Soc. 2013;88:645–668.10.1111/brv.2013.88.issue-3
- Steegborn C. Structure, mechanism, and regulation of soluble adenylyl cyclases-similarities and differences to transmembrane adenylyl cyclases. Biochim. Biophys. Acta. 2014;1842:2535–2547.10.1016/j.bbadis.2014.08.012
- Hacker BM, Tomlinson JE, Wayman GA, et al. Cloning, chromosomal mapping, and regulatory properties of the human type 9 adenylyl cyclase (ADCY9). Genomics. 1998;50:97–104.10.1006/geno.1998.5293
- Stocco DM, Wang X, Jo Y, et al. Multiple signaling pathways regulating steroidogenesis and steroidogenic acute regulatory protein expression: more complicated than we thought. Mol. Endocrinol. 2005;19:2647–2659.10.1210/me.2004-0532