Abstract
Isomerization of disaccharides (maltose, isomaltose, cellobiose, lactose, melibiose, palatinose, sucrose, and trehalose) was investigated in subcritical aqueous ethanol. A marked increase in the isomerization of aldo-disaccharides to keto-disaccharides was noted and their hydrolytic reactions were suppressed with increasing ethanol concentration. Under any study condition, the maximum yield of keto-disaccharides produced from aldo-disaccharides linked by β-glycosidic bond was higher than that produced from aldo-disaccharides linked by α-glycosidic bond. Palatinose, a keto-disaccharide, mainly underwent decomposition rather than isomerization in subcritical water and subcritical aqueous ethanol. No isomerization was noted for the non-reducing disaccharides trehalose and sucrose. The rate constant of maltose to maltulose isomerization almost doubled by changing solvent from subcritical water to 80 wt% aqueous ethanol at 220 °C. Increased maltose monohydrate concentration in feed decreased the conversion of maltose and the maximum yield of maltulose, but increased the productivity of maltulose. The maximum productivity of maltulose was ca. 41 g/(h kg-solution).
Graphical abstract
Promoted production of keto-disaccharides from aldo-disaccharides in subcritical aqueous ethanol.
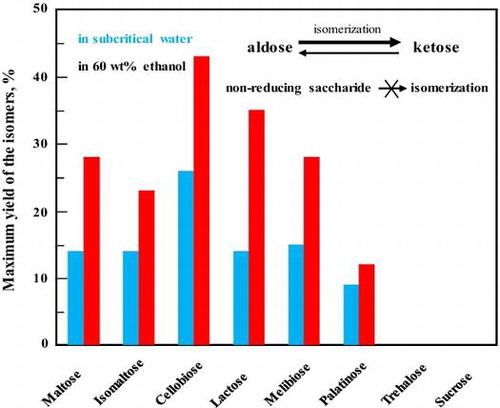
Keto-disaccharides such as maltulose, palatinose, lactulose, cellobiulose, and melibiulose are of great interest in studies of food nutritionCitation1) and biomass transformation,Citation2,3) as well as in organic synthesis, because they are natural chiral molecules.Citation4) As a food additive, for example, lactulose promotes the growth of BifidobacteriumCitation5) and decreases the formation of putrefactive products,Citation6) increasing health benefits for human beings.
Keto-disaccharides can be mainly synthesized by isomerization of the corresponding aldo-disaccharides using alkali, homogeneous or heterogeneous metal, and enzymatic catalysts.Citation7–12) Among the above methods, only alkali-catalyzed isomerization is versatile and can be reluctantly applied in food industry for reasons of food safety and productivity. However, even in alkali-catalyzed isomerization, a long time was required to attain reaction equilibrium and post-treatment processing was tedious. Therefore, a versatile and convenient method should be developed.
We reported that subcritical aqueous ethanol could be used as an excellent solvent for aldohexose to ketohexose isomerizations.Citation13–15) Our kinetic analysis showed that, compared to the decomposition steps, the isomerization steps accelerated when changing reaction solvent from subcritical water to subcritical aqueous ethanol.Citation15) Besides, the chemical equilibrium constant was high (ca. 3 at 200 °C for glucose to fructose isomerization), which suggested a possibly high yield of ketohexoses. Importantly, we reported that sucrose hydrolysis was strongly restricted in subcritical aqueous ethanol than in subcritical water;Citation16) this may be also applicable to other disaccharides. These results suggest that high yields of keto-disaccharides can be produced from the corresponding aldo-disaccharides using subcritical aqueous ethanol.
In the current study, we attempted to efficiently produce keto-disaccharides, maltulose, palatinose, cellobiulose, lactulose, and melibiulose, from the corresponding aldo-disaccharides, maltose, isomaltose, cellobiose, lactose, and melibiose, respectively, using subcritical aqueous ethanol, and to investigate the effect of the type of glycoside linkage of aldo-disaccharides on their isomerizations.
Materials and methods
Materials
Maltose (α-d-Glc-(1→4)-d-Glc) monohydrate; melibiose (α-d-Gal-(1→6)-d-Glc); lactose (β-d-Gal-(1→4)-d-Glc); sucrose (α-d-Glc-(1→2)-β-d-Fru), where Glc, Gal, and Fru represent d-glucose, d-galactose, and d-fructose, respectively; d-glucose, d-fructose, d-galactose, d-tagatose, d-talose, and ethanol were purchased from Wako Pure Chemical Industries (Osaka, Japan). Cellobiose (β-d-Glc-(1→4)-d-Glc), palatinose (α-d-Glc-(1→6)-d-Fru), syrupy isomaltose (α-d-Glc-(1→6)-d-Glc), and trehalose (α-d-Glc-(1→1)-d-Glc) were obtained from Hayashibara Biochemical Laboratories (Okayama, Japan). Lactose and d-mannose were purchased from Nacalai Tesque (Kyoto, Japan). Maltulose (α-d-Glc-(1→4)-d-Fru) monohydrate and isomaltose for calibration were purchased from Tokyo Chemical Industry (Tokyo, Japan). Cellobiulose (β-d-Glc-(1→4)-d-Fru) and melibiulose (α-d-Gal-(1→6)-d-Fru) for calibration were prepared and purified in this study, according to the methods described later. Because the present study only focused on the isomerization of the d-enantiomers, the prefix, d-, of all the saccharides is omitted hereafter.
Isomerization or hydrolysis of saccharides in subcritical aqueous ethanol
Isomerization of each saccharide was carried out in a continuous reaction system, in which the feed saccharide solution was delivered using an LC-10AD VP HPLC pump (Shimadzu, Kyoto, Japan), into a stainless steel tubular reactor (0.8 mm I.D. × 1.0 m length) heated in a temperature-controlled silicone oil bath (Dow Corning Toray Silicone, Tokyo, Japan). Upon exiting the reactor, the effluent was directly introduced to a stainless steel tube immersed in an ice water bath in order to quench the reaction. The pressure inside the reactor was regulated at ca. 10 MPa and reduced to ambient after cooling using a back-pressure valve (Upchurch Scientific Inc., Oak Harbor, WA, USA). The residence time was set in the range of 50–500 s. The reaction temperature was set at 180–220 °C. Ethanol concentration was adjusted from 0 to 80 wt% and the substrate feed concentration ranged from 0.5 to 5 wt% for maltose isomerization. For other saccharides, the reaction temperature was set at 200 °C; ethanol concentration was adjusted at 0 (subcritical water) and 60 wt% and the feed concentration was 0.5 wt%.
The residence time was calculated from the volumetric flow rate measured at room temperature according to the inner diameter, length of the stainless steel tube, and density of the subcritical aqueous ethanol, which was calculated according to the densities of water and ethanol.Citation17,18)
Saccharide analysis
The reaction effluent was compositionally analyzed using an HPLC system, which consisted of an LC-10AD VP pump (Shimadzu), a Cosmosil Sugar-D column (4.6 mm I.D. × 250 mm length, Nacalai Tesque), and an RI-101 refractometer (Showa Denko, Tokyo). To separate the yielded glucose and galactose clearly, another column, Asahipak NH2P-50 4E (4.6 mm I.D. × 250 mm length) was used for compositional analysis of the reaction mixture of lactose and melibiose. Column temperature was maintained at 30 °C in a CTO-10AVP column oven (Shimadzu). The mobile phase for both analyses was 80% (v/v) aqueous acetonitrile solution at a flow rate of 1.0 mL/min. The calibration curves were prepared using commercially available saccharides or saccharides prepared and purified in this study.
Product purification and NMR confirmation
The purification procedures for the produced keto-disaccharides were the same as those reported in our previous study.Citation14)
1H and 13C NMR analyses were performed using an Ascend 400 NMR spectrometer (400 MHz Bruker Japan, Osaka, Japan) with D2O as the solvent. Acetonitrile (δH = 2.06 ppm, δC (13CH3) = 1.47 ppm, δC (13CN) = 119.68 ppm) was used as the internal standard. Further, the 1H and 13C NMR spectra of maltulose, palatinose, and lactulose were compared with those of the commercial samples and reported data.Citation19–21) Because cellobiulose and melibiulose are not commercially available, we used 1H and 13C NMR spectra of suspected cellobiulose and melibiulose for comparison. Cellobiose and melibiose have been shown to undergo isomerization to their ketoses in subcritical water or in aqueous alkaline solutions,Citation2,22) and we have reported the promotion of aldose to ketose isomerization in subcritical aqueous ethanol.Citation13–15) Therefore, the formation of cellobiulose and melibiulose could be assured.
Solubility of maltose monohydrate in aqueous ethanol
The solubility of maltose monohydrate in aqueous ethanol was measured at 25 °C according to our previous method.Citation14)
Results and discussion
Effect of ethanol concentration on maltose isomerization
Maltose underwent slow conversion below 220 °C and rapid conversion at temperatures higher than 220 °C in subcritical water.Citation23) Further, we have reported that the conversion of reducing hexose was increasedCitation15) and hydrolysis of sucrose was restrictedCitation16) by increasing ethanol concentration. Therefore, the reaction temperature of 220 °C was selected to investigate the effect of ethanol concentration on hydrolysis and isomerization of maltose. Fig. shows the isomerization and hydrolysis processes of maltose in subcritical water and 60 wt% subcritical aqueous ethanol at 220 °C. To easily calculate the mass balance during the reaction, the yield of each produced monosaccharide was defined as the molar ratio of produced monosaccharide to glucose residues of maltose. Glucose, fructose, mannose, and maltulose were produced from maltose in subcritical water and 60 wt% aqueous ethanol. The formation of maltulose was assured by comparing the 1H and 13C NMR spectra of the suspected maltulose with that of the commercial maltulose (Fig. ). However, epimaltose, a C-2 epimer of glucose in the reducing side of maltose, was not detected. It has also been reported that epimaltose is not detected in alkali-catalyzed maltose isomerization.Citation24) This may be because of its low yield controlled by thermodynamic equilibrium and high isomerization reactivity under subcritical aqueous conditions, since the reducing side of epimaltose is a mannose residue. We previously reported that among the mutual isomerization of glucose, mannose, and fructose, mannose was the most easily isomerized, and its formation from glucose and fructose was difficult in subcritical aqueous ethanol.Citation15) In subcritical water, glucose was the most producible and its maximum yield was ca. 45% at the residence time of ca. 400 s. The yield of maltulose increased with the residence time until 100 s and then decreased, indicating that the maltulose formed had decomposed. Fructose, which can be directly obtained through hydrolysis of maltulose, as well as through isomerization of glucose and mannose, was gradually produced by prolonging the residence time; however, the yield of fructose was <15%. On the other hand, <0.5% mannose was obtained within the investigated residence time. Changing the reaction solvent from subcritical water to 60 wt% subcritical aqueous ethanol significantly suppressed the hydrolysis of maltose, and all the hexoses were produced in <10% yields. We previously reported that ethanol showed dilute effect on water concentration during sucrose hydrolysis.Citation16) It may be also suggested that ethanol affects the maltose hydrolysis in the same manner. The yield of maltulose in 60 wt% ethanol was higher than that in subcritical water at the same residence time. Rapid conversion of maltose occurred in 60 wt% subcritical aqueous ethanol until ca. 200 s, after which the speed of conversion decreased to lesser than that in subcritical water. This could be because the addition of ethanol promoted the isomerization of maltulose to maltose and because the isomerization dominantly affected maltose conversion after 200 s as previously shown.Citation15)
Fig. 1. Isomerization and hydrolysis processes of (♢) maltose to (△) maltulose, (□) glucose, (○) fructose, and (▹) mannose in (a) subcritical water and (b) 60 wt% subcritical aqueous ethanol at 220 °C. The feed concentration of maltose monohydrate was 0.5 wt%.
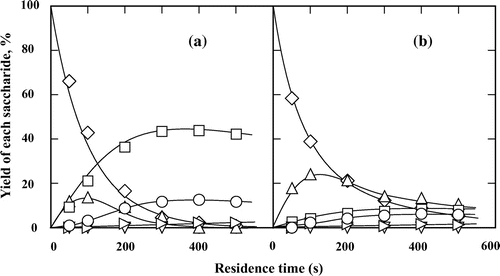
Fig. 2. 1H and 13C NMR spectra of the standard and purified sample of maltulose. (a) 1H NMR of standard sample of maltulose, (b) 1H NMR of purified sample of maltulose, (c) 13C NMR of standard sample of maltulose, and (d) 3C NMR of purified sample of maltulose.
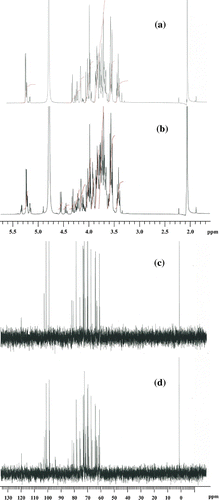
The above-mentioned results clearly showed that hydrolysis and isomerization of maltose were more suppressed and promoted, respectively, in subcritical aqueous ethanol than in subcritical water. In order to investigate the effect of ethanol concentration on maltose isomerization in detail, we further examined maltose isomerization at different ethanol concentrations (Table ). The maximum yield of maltulose and its selectivity at that yield, 24 and 39%, respectively, were higher at high ethanol concentration. The rate constant for isomerization of maltose to maltulose, which was calculated from the initial slope of time course for maltulose formation, almost doubled when changing the solvent from water to 80 wt% aqueous ethanol.
Table 1. Effect of ethanol concentration on maltose isomerization in subcritical aqueous ethanol at 220 °C.
Temperature dependence of maltose isomerization
Effect of reaction temperature on the isomerization of maltose to maltulose was examined in 60 wt% aqueous ethanol at the feed substrate concentration of 0.5 wt% (Table ). The maximum yield of maltulose markedly increased by ca. 10% by increasing the reaction temperature from 180 to 200 °C. However, reaction temperatures above 200 °C were not suitable for producing maltulose within the residence time of 500 s, because relatively low maximum yields were obtained at such temperatures. On the other hand, at the temperatures above 200 °C, the maximum yield of maltulose was achieved at short residence times. Thus, the disadvantages of increasing the reaction temperature were significant decreases in selectivity of maltulose and total sugar content obtained at specific residence times, for example, a residence time of 500 s. These results indicated that increasingly significant decompositions of di- and monosaccharides occurred at high temperature.
Table 2. Effect of temperature on maltose isomerization in 60 wt% aqueous ethanol.
The rate constant of maltose-to-maltulose isomerization increased by increasing the reaction temperature, and the activation energy was calculated to be ca. 110 kJ/mol according to the Arrhenius plot (Fig. ), which was similar to that of glucose-to-fructose isomerization in subcritical aqueous ethanolCitation15) and that of maltose-to-maltulose isomerization in alkaline solution.Citation24)
Effects of the type of glycoside linkage and constituent monosaccharides of the disaccharides on their isomerization and hydrolysis
To investigate the effects of the type of glycoside linkage and constituent monosaccharides on the reaction behaviors of disaccharides, we assessed the effects of seven other disaccharides (isomaltose, cellobiose, lactose, melibiose, palatinose, trehalose, and sucrose) in subcritical water and 60 wt% aqueous ethanol at 200 °C (Figs. ). In contrast to maltose, the other tested aldo-disaccharides (isomaltose, cellobiose, lactose, and melibiose) predominantly underwent isomerization rather than hydrolysis in subcritical water and 60 wt% aqueous ethanol. The addition of ethanol suppressed the hydrolysis of disaccharides and promoted their conversion to the corresponding keto-disaccharides. The formation of palatinose from isomaltose (Fig. ) and lactulose from lactose (Fig. ) were also assured by comparing the 1H and 13C NMR spectra of the suspected products with those of the commercial ones (data not shown). The formation of cellobiulose from cellobiose (Fig. ) and melibiulose from melibiose (Fig. ) was assured by comparing the change in the 1H and 13C NMR spectra between the substrates and the purified samples and by the fact that ketose can be produced in high yield from the corresponding aldose in subcritical aqueous ethanol.Citation13–15) The yields and selectivities of cellobiulose, lactulose, and melibiulose increased in 60 wt% aqueous ethanol than in subcritical water. The selectivity of the produced disaccharides was defined as the molar ratio of the product to the consumed substrate. Moreover, these results also showed that the type of glycoside linkage affected the isomerization of the aldo-disaccharides, i.e. the aldo-disaccharides linked by β-glycosidic bond (cellobiose and lactose) were more easily isomerized than those linked by α-glycosidic bond (isomaltose and melibiose) in subcritical aqueous ethanol. However, for maltose and isomaltose with α glycoside linkage, it was showed that the yield of the produced keto-disaccharides was not significantly affected by the binding position of glucose on reducing side.
Fig. 4. Isomerization and hydrolysis processes of (♢) isomaltose to (△) palatinose, (∇) epiisomaltose, (□) glucose, (○) fructose, and (▹) mannose in (a) subcritical water and (b) 60 wt% subcritical aqueous ethanol at 200 °C. The feed concentration of isomaltose was 0.5 wt%.
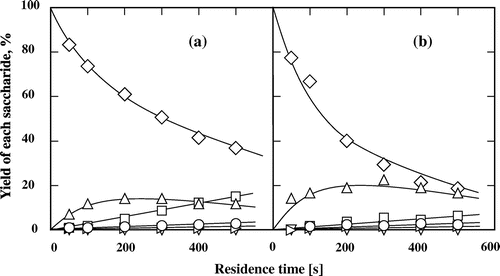
Fig. 5. Isomerization and hydrolysis processes of (♢) cellobiose to (△) cellobiulose, (∇) epicellobiose, (□) glucose, (○) fructose, and (▹) mannose in (a) subcritical water and (b) 60 wt% subcritical aqueous ethanol at 200 °C. The feed concentration of cellobiose was 0.5 wt%.
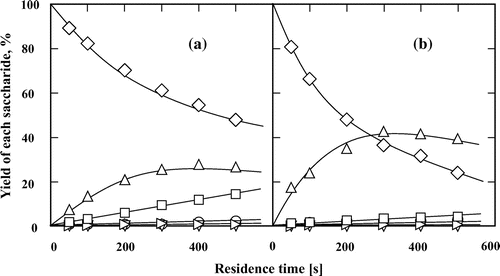
Fig. 6. Isomerization and hydrolysis processes of (♢) lactose to (△) lactulose, (∇) epilactose, (□) glucose, (○) fructose, (▹) mannose, (■) galactose, (●) tagatose, and (►) talose in (a) subcritical water and (b) 60 wt% subcritical aqueous ethanol at 200 °C. The feed concentration of lactose was 0.5 wt%.
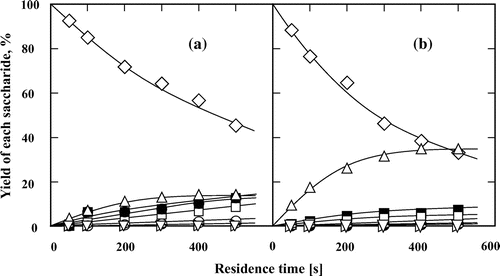
Fig. 7. Isomerization and hydrolysis processes of (♢) melibiose to (△) melibiulose, (∇) epimelibiose, (□) glucose, (○) fructose, (▹) mannose, (■) galactose, (●) tagatose, and (►) talose in (a) subcritical water and (b) 60 wt% subcritical aqueous ethanol at 200 °C. The feed concentration of melibiose was 0.5 wt%.
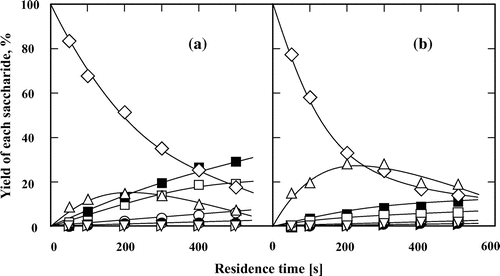
Fig. 8. Isomerization and hydrolysis processes of (♢) palatinose to (△) isomaltose, (□) glucose, (○) fructose, and (▹) mannose in (a) subcritical water and (b) 60 wt% subcritical aqueous ethanol at 200 °C. The feed concentration of palatinose was 0.5 wt%.
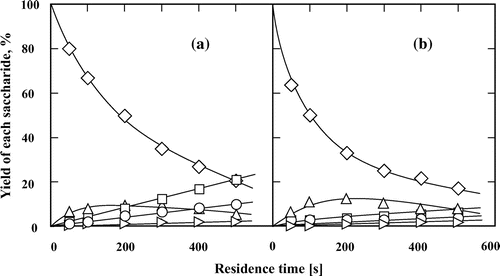
Fig. 9. Hydrolysis processes of (♢,♦) trehalose to (○,●) glucose, in subcritical water (open symbols) and 60 wt% subcritical aqueous ethanol (closed symbols) at 200 °C. The feed concentration of trehalose was 0.5 wt%.
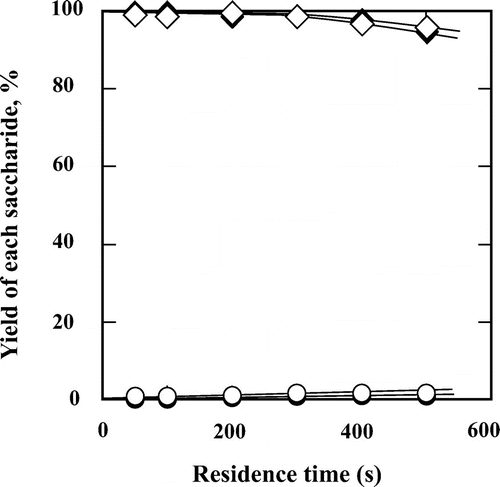
Fig. 10. Hydrolysis processes of (♢) sucrose to (□) glucose, (○) fructose, and (△) mannose in 60 wt% subcritical aqueous ethanol at 200 °C. The feed concentration of sucrose was 0.5 wt%.
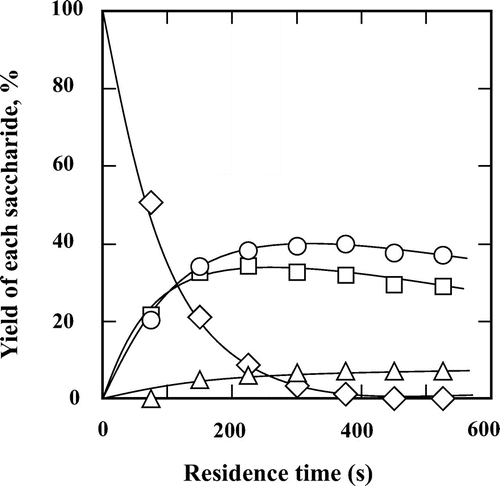
Palatinose was used as a typical substrate to investigate the isomerization of keto-disaccharide in subcritical water and 60 wt% subcritical aqueous ethanol (Fig. ). Monosaccharides were the dominant products in subcritical water. Changing the reaction solvent to 60 wt% ethanol suppressed hydrolysis to improve the conversion of palatinose and yield of isomaltose, and suppressed hydrolysis. The maximum yield of isomaltose was ca. 10%. Compared with isomaltose, palatinose yield decreased rapidly at short residence times. However, the yield of isomaltose formed from palatinose was lower than that of palatinose formed from isomaltose under the same reaction conditions, indicating that increasing difficulty in the isomerization of palatinose to isomaltose. We previously reported that ketohexose had a low ability to isomerize than aldohexose.Citation15) These results show that reducing keto-disaccharides mainly underwent decomposition rather than isomerization in subcritical aqueous ethanol.
In order to compare the isomerization of aldo-disaccharides with that of monosaccharides, isomerizations of glucose and galactose were also investigated in 60 wt% ethanol at 200 °C (data not shown). Fructose and tagatose were obtained from glucose and galactose at the maximum yields of 29 and 26%, respectively. No significant difference in the initial reaction rate was observed between reducing aldo-disaccharides and monosaccharides. This observation differed from that in alkaline solution. Previous studies have shown that reducing disaccharides isomerized considerably faster than did monosaccharides in alkaline solution.Citation25,26) We believe that the reason for no difference in the initial reaction rate between reducing aldo-disaccharides and monosaccharides in this study can be attributed to the change in temperature dependence of the isomerization rate constants under subcritical conditions.
Trehalose and sucrose, which are non-reducing disaccharides, only underwent hydrolysis and isomerization of their constituent monosaccharides (Figs. and ). However, trehalose was hydrolyzed slowly with little effect of the ethanol concentration. Treatment of trehalose in subcritical water and in 60 wt% ethanol for 500 s achieved a conversion of ca. 5%. On the other hand, in auto-catalytic mode, sucrose was easily hydrolyzed to fructose and glucose as the main products, which further slightly isomerized to mannose.Citation13,15,16) These results definitely showed that only reducing disaccharides can be isomerized in subcritical water and subcritical aqueous ethanol.
Productivity of maltulose at different feed maltose concentration
The results demonstrated above suggested a possible process to produce rare keto-disaccharides using subcritical aqueous ethanol. Maltose to maltulose isomerization was typically selected to examine the effect of feed substrate concentration on the isomerization in 60 wt% aqueous ethanol at 200 °C. We previously reported that high feed concentration of galactose improved productivity of the desired tagatose.Citation14) This would be expected to be true for maltulose production. The solubility of maltose monohydrate in subcritical aqueous ethanol was measured at 25 °C (Fig. ). The solubility of maltose monohydrate significantly decreased by the addition of ethanol from ca. 60 wt% in water to ca. 1 wt% in 80 wt% aqueous ethanol. The maximum solubility of maltose monohydrate was ca. 5.5 wt% in 60 wt% aqueous ethanol at 25 °C, which was lower than that of galactose.Citation14) To avoid the precipitation of maltose in the tubular reactor, we used a feed maltose concentration ranging from 0.5 to 5 wt%.
The maximum yields of maltulose were obtained at the residence time of 500 s within the tested residence time range at any feed maltose monohydrate concentrations (Table ). The maximum yield of maltulose and corresponding conversion of maltose decreased from 29 and 79% at 0.5 wt% maltose monohydrate to 18 and 51% at 5 wt%, respectively. However, the maximum productivity of maltulose increased almost sevenfold to ca. 41 g/(h kg-solution). Besides, the selectivity of maltulose was little affected by the change in feed substrate concentration, and the total sugar content was >70% at a feed substrate concentration of 5 wt%. These results suggest that subcritical aqueous ethanol is a suitable solvent for effectively producing the rare keto-disaccharides from their corresponding common aldo-saccharides.
Table 3. Isomerization of maltose in 60 wt% aqueous ethanol at 200 °C at different feed concentrations.
In conclusion, it was demonstrated that maltulose, palatinose, cellobiulose, lactulose, and melibiulose can be produced by isomerization of the corresponding aldo-disaccharides in subcritical aqueous ethanol. The hydrolytic and isomerization reactions of disaccharides were suppressed and promoted, respectively, by increasing ethanol concentration, which lead to high maximum yields of keto-disaccharides. The type of glycoside linkage and constituent monosaccharides affected the isomerization of disaccharides. Higher yields of keto-disaccharides linked by β-glycosidic bond than by α-glycosidic bond from the corresponding aldo-disaccharides were produced. On the other hand, the keto-disaccharide, palatinose, mainly underwent decomposition rather than isomerization.
Author contributions
All the authors conceived and designed the experiments and discussed the results. D.-M.G. performed the experiments, analyzed the data and wrote the manuscript in consultation with T.K. and S.A.
Funding
This work was partly supported by the Tojuro Iijima Foundation for Food Science and Technology (D.-M.G.) and JSPS KAKENHI [grant Number 26870296]; T. K.
Disclosure statement
No potential conflict of interest was reported by the authors.
References
- Kunz C, Rudloff S. Biological functions of oligosaccharides in human milk. Acta Paediatr. 1993;82:903–912.10.1111/apa.1993.82.issue-12
- Yu Y, Shafie ZM. W H. Cellobiose decomposition in hot-compressed water: importance of isomerization reactions. Ind. Eng. Chem. Res. 2013;52:17006–17014.10.1021/ie403140q
- Martin D, Lichtenthaler FW. Versatile building blocks from disaccharides: glycosylated 5-hydroxymethylfurfurals. Tetrahedron: Asymmetry. 2006;17:756–762.10.1016/j.tetasy.2005.12.010
- Guazzelli L, Catelani G, D’Andrea F, et al. Stereoselective access to the β-D-N-acetylhexosaminyl-(1→4)-1-deoxy-D-nojirimycin disaccharide series avoiding the glycosylation reaction. Eur. J. Org. Chem. 2014;2014:6527–6537.
- Schuster-Wolff-Bühring R, Fischer L, Hinrichs J. Production and physiological action of the disaccharide lactulose. Int. Dairy J. 2010;20:731–741.10.1016/j.idairyj.2010.05.004
- Seki N, Saito H. Lactose as a source for lactulose and other functional lactose derivatives. Int. Dairy J. 2012;22:110–115.10.1016/j.idairyj.2011.09.016
- Corbett WM, Kenner J. The degradation of carbohydrates by alkali. Part IX. Cellobiose, cellobiulose, cellotetraose, and laminarin. J. Chem. Soc. 1955;1431–1435.10.1039/jr9550001431
- Corbett WM, Kenner J. 462. The degradation of carbohydrates by alkali. Part II. Lactose. J. Chem. Soc. 1953;2245–2247.10.1039/jr9530002245
- Corbett WM, Kenner J. The degradation of carbohydrates by alkali. Part V. Lactulose, maltose, and maltulose. J. Chem. Soc. 1954;1789–1791.10.1039/jr9540001789
- Shukla R, Verykios XE, Mutharasan R. Isomerization and hydrolysis reactions of important disaccharides over inorganic heterogeneous catalysts. Carbohydr. Res. 1985;143:97–106.10.1016/S0008-6215(00)90699-2
- Gounder R, Davis ME. Monosaccharide and disaccharide isomerization over Lewis acid sites in hydrophobic and hydrophilic molecular sieves. J. Catal. 2013;308:176–188.10.1016/j.jcat.2013.06.016
- Shen Q, Yang R, Hua X, et al. Enzymatic synthesis and identification of oligosaccharides obtained by transgalactosylation of lactose in the presence of fructose using β-galactosidase from Kluyveromyces lactis. Food Chem. 2012;135:1547–1554.10.1016/j.foodchem.2012.05.115
- Gao D-M, Kobayashi T, Adachi S. Promotion or suppression of glucose isomerization in subcritical aqueous straight-and branched-chain alcohols. Biosci. Biotechnol. Biochem. 2015;79:470–474.10.1080/09168451.2014.973366
- Gao D-M, Kobayashi T, Adachi S.. Production of rare sugars from common sugars in subcritical aqueous ethanol. Food Chem. 2015;175:465–470.10.1016/j.foodchem.2014.11.144
- Gao D-M, Kobayashi T, Adachi S. Kinetic analysis for the isomerization of glucose, fructose, and mannose in subcritical aqueous ethanol. Biosci. Biotechnol. Biochem. 2015;79:1005–1010.10.1080/09168451.2014.1003129
- Gao D-M, Kobayashi T, Adachi S. Kinetics of sucrose hydrolysis in a subcritical water-ethanol mixture. J. Appl. Glycosci. 2014;61:9–13.10.5458/jag.jag.JAG-2013_006
- Bazaev AR, Abdulagatov IM, Bazaev EA, et al. PVT measurements for pure ethanol in the near-critical and supercritical regions. Int. J. Thermophys. 2007;28:194–219.10.1007/s10765-007-0158-2
- Sommer D, Kleinrahm R, Span R, et al. Measurement and correlation of the (p, ρ, T) relation of liquid cyclohexane, toluene, and ethanol in the temperature range from 233.15 K to 473.15 K at pressures up to 30 MPa for use as density reference liquids. J. Chem. Thermodyn. 2011;43:117–132.10.1016/j.jct.2010.08.010
- Pfeffer PE, Hicks KB. Characterization of keto disaccharides in solution by deuterium-induced, differential isotope-shift 13C-NMR spectroscopy. Carbohydr. Res. 1982;102:11–22.10.1016/S0008-6215(00)88046-5
- Low NH, Brisbane T, Bigam G, et al. Carbon-13 nuclear magnetic resonance for the qualitative and quantitative analysis of structurally similar disaccharides. J. Agric. Food Chem. 1988;36:953–957.10.1021/jf00083a014
- Kimura H, Nakahara M, Matubayasi N. Noncatalytic hydrothermal elimination of the terminal D-glucose unit from malto- and cello-oligosaccharides through transformation to D-fructose. J. Phys. Chem. A. 2012;116:10039–10049.10.1021/jp3034165
- Corbett WM, Kenner J. The degradation of carbohydrates by alkali. Part VIII). Melibiose. J. Chem. Soc. (Resumed) 1954;3281–3283.
- Haghighat Khajavi S, Kimura Y, Oomori T, et al. Decomposition kinetics of maltose in subcritical water. Biosci. Biotechnol. Biochem. 2004;68:91–95.
- Vuorinen T. Kinetics of alkaline degradation of maltose in ethanol-water solutions. Carbohydr. Res. 1982;108:213–219.10.1016/S0008-6215(00)81791-7
- Isbell HS, Frush HL, Wade CWR, et al. Transformations of sugars in alkaline solutions. Carbohydr. Res. 1969;9:163–175.10.1016/S0008-6215(00)82132-1
- Vuorinen T, Sjöström E. Kinetics of alkali-catalyzed isomerization of D-glucose and D-fructose in ethanol-water solutions. Carbohydr. Res. 1982;108:23–29.10.1016/S0008-6215(00)81886-8